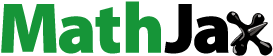
Abstract
In this work, Zn–Al layered double hydroxides intercalated with carbonate, nitrate, chloride and sulphate ions were synthesised via a co-precipitation method at a constant solution pH. The as-synthesised samples were characterised by X-ray diffraction (XRD), Fourier transform infrared spectroscopy (FTIR), transmission electron microscopy (TEM) and simultaneous thermogravimetric/differential thermal analysis (TGA-DTA). The XRD patterns showed that Zn-Al-SO4 had the greatest interlayer spacing, followed by Zn-Al-Cl, Zn-Al-NO3 and Zn-Al-CO3. The FTIR spectra clearly confirmed the presence of intercalated anions in the structure of the LDHs. Batch experiments for methyl orange (MO) adsorption onto synthesised samples were investigated under various conditions such as contact time, initial dye concentration and solution pH. The experimental results show that pH is the most influencing factor. The effective pH range for the MO removal was found to be 3.5–4.5. The kinetics data can be described accurately by a pseudo-second-order kinetic model instead of a pseudo-first-order model. The equilibrium data were analysed using the Langmuir, Freundlich and Dubinin–Radushkevich models. The results showed that the Langmuir and Dubinin–Radushkevich isotherm models fit well to the experimental data. The Zn-Al-LDH samples exhibited exceptional Langmuir maximum adsorption capacities of 2758, 2455, 2270 and 1684 mg/g for Zn-Al-SO4, Zn-Al-Cl, Zn-Al-NO3 and Zn-Al-CO3, respectively.
1 Introduction
Wastewater from the dyestuff manufacturing, dyeing, printing and textile industries presents a serious problem all over the world because dyes and pigments in wastewater undergo chemical changes and destroy aquatic life and can even endanger human health. Most of these dyes are toxic, mutagenic and carcinogenic [Citation1–Citation4]. According to a survey of the ecological and toxicological association of the dyestuffs manufacturing industry (ETAD), over 90% of some 4000 dyes have LD50 values greater than 2000 mg/kg [Citation5,Citation6]. From an environmental point of view, the remediation of wastewater is a great challenge and has attracted tremendous attention in recent years. Different methods have been used for dye removal from wastewaters such as biological degradation [Citation7], photodegradation [Citation8], coagulation [Citation9], membrane filtration [Citation10], reverse osmosis [Citation11], adsorption [Citation12], or the synergic treatment using different methods. Among these methods, the adsorption process is one of the most effective techniques that has been successfully employed for colour removal because it is considered to be less expensive, more efficient and less likely to generate secondary waste [Citation13]. Most conventional adsorption systems use activated carbon despite its high production costs and regeneration difficulty [Citation14]. Recently, many low-cost adsorbents have been investigated in the removal of dyes such as agricultural wastes [Citation15], clays [Citation16], chitosan [Citation17], silica [Citation18], kaolinite [Citation19], perlite [Citation20], sepiolite [Citation21], montmorillonite [Citation22] and some natural biosorbents [Citation23]. However, these materials generally have low adsorption capacities, and hence, a large adsorbent dosage is required to achieve a low dye concentration in the treated effluents.
Layered double hydroxides (LDHs), including hydrotalcite and hydrotalcite-like compounds, have received considerable attention due to their potential applications in catalysis, photochemistry, electrochemistry, polymerisation, magnetisation, biomedical science and environmental applications [Citation24–Citation28]. LDHs are host-guest materials consisting of positively charged metal oxide/hydroxide sheets with intercalated anions and water molecules. They can be represented by the general formula: [M1−x2+Mx3+ (OH)2] (An−)x/n·mH2O, where M2+ is a divalent cation such as Mg2+, Zn2+, Cu2+, etc., and M3+ is a trivalent cation such as Al3+, Cr3+, Fe3+, etc. Due to the partial substitutions of M3+ for M2+, the hydroxide sheets are positively charged and require the intercalation of anions (An−), such as CO32−, NO3−, Cl−, or SO42−, to retain the overall charge neutrality. The composition of LDH materials is tunable. That is, the divalent cation or trivalent cation can be replaced or partly replaced by other cations, and the interlayer anion can also be replaced by other anions; thus, their physical and chemical properties can be altered [Citation29]. LDHs exhibit favourable characteristics for the adsorption of pollutants such as large surface areas, good thermal stabilities, and high sorption and regeneration efficiencies [Citation30–Citation33]. Moreover, LDHs are regarded as a class of materials that are simple and economical to synthesise in the laboratory.
In the present work, zinc aluminium-layered double hydroxides intercalated with carbonate, nitrate, chloride, or sulphate were synthesised via a co-precipitation method. The samples were characterised by powder XRD, FTIR, TEM and TGA-DTA. The effects of various parameters such as solution pH, contact time and dye concentration on the removal of methyl orange by these precursors were studied in detail. The adsorption kinetic data were tested by pseudo-first-order and pseudo-second-order kinetic models. The equilibrium data were analysed using the Langmuir, Freundlich and Dubinin–Radushkevich isotherm models.
2 Experimental
2.1 Materials
All of the chemicals used in this study were of analytical grade. Zn(NO3)2·6H2O, Al(NO3)3·9H2O, Na2CO3, Na2SO4, NaCl, NaNO3 and methyl orange were purchased from Sigma–Aldrich (Germany). NaOH was purchased from Merck (Germany) and HNO3 from Scharlau (Spain). The chemical structure and some other specific characteristics of methyl orange are summarised in .
Table 1 Physicochemical characteristics of methyl orange.
2.2 Synthesis of Zn–Al layered double hydroxides
Zn–Al layered double hydroxides intercalated with CO32−, NO3−, Cl−, or SO42− were synthesised via a co-precipitation method at a constant solution pH of 10. An aqueous solution containing Zn(NO3)2·6H2O (0.75 M) and Al(NO3)3·9H2O (0.25 M) was added dropwise to a vigorously stirred solution of NaOH (2 M) and 0.2 M of the desired anion (CO32−, NO3−, Cl−, or SO42−). The resulting gels were hydrothermally treated at 80 °C for 24 h to improve their crystallinities, were filtered, and then were washed repeatedly with deionised water. Finally, they were dried at 60 °C for 24 h. The obtained products were called Zn-Al-CO3, Zn-Al-NO3, Zn-Al-Cl and Zn-Al-SO4.
2.3 Characterisation of the samples
The powder X-ray diffraction (XRD) patterns were recorded from 2θ = 5–70° using a Bruker-axs D2-phaser advance diffractometer operating at 30 kV and 10 mA with Cu Kα1 (1.54056 Å) and Kα2 (1.54439 Å) radiations. The FTIR spectra were collected on a Nicolet Avatar 330 Fourier transform IR spectrophotometer. The samples were mixed with KBr at a mass ratio of 1:100 and finely powdered to prepare pellets. The spectra were recorded with a 2 cm−1 resolution in the range of 4000–400 cm−1. Transmission electron microscopy (TEM) images were obtained on a TEM TECNAI G2/FEI instrument at an accelerating voltage of 120 kV. The simultaneous thermogravimetric-differential thermal analysis (TGA-DTA) curves were recorded on a SETARAM Labsys™ Evo model instrument under argon atmosphere in the temperature range of 30–700 °C and with a heating rate of 10 °C/min.
2.4 Adsorption experiments
Stock solutions of 1 g/L of MO were prepared by dissolving the appropriate amount of the dye in distilled water, and the concentrations that were used were obtained by dilution. The adsorption experiments were performed in a series of 250-mL beakers containing the desired weight (20 mg) of each LDH and 250 mL of the methyl orange solution at the desired concentration. These experiments were carried out at a constant agitation speed of 500 rpm by varying the pH of the solution from 2 to 12, the contact time from 5 to 360 min and the initial dye concentration from 20 to 1000 mg/L. The solution pH was adjusted to a given value with the addition of HCl (1 N) or NaOH (1 N), and it was measured using a SensION+ PH31 pH meter.
After each adsorption experiment was completed, the solid phase was separated from the liquid phase by centrifugation at 3000 rpm for 15 min. The residual concentrations were determined from UV–Vis characteristics at a maximum absorption wavelength of 465 nm using a TOMOS V-1100 UV–vis spectrophotometer.
The adsorbed amounts at equilibrium (qe) and at any time (qt) were calculated using the following equation:(1)
(1) where qe,t (mg/g) is the adsorbed quantity at equilibrium (qe) or at any time (qt), C0 (mg/L) is the initial dye concentration, Ce,t (mg/L) is the dye concentration at equilibrium (Ce) or at any time (Ct), V (L) is the volume of the solution, and m (g) is the mass of the LDH sample (g).
3 Results and discussion
3.1 Characterisation
3.1.1 XRD analysis
The XRD patterns of the samples, shown in , exhibit the characteristic reflections of layered double hydroxides. The diffraction peaks were indexed to a hexagonal lattice with rhombohedral 3R symmetry [Citation24]. The basal peaks for the (003) and (006) planes at 2θ values between 7 and 26° and the non-basal peaks for the (101), (015), (018), (110) and (113) planes at higher 2θ values can be observed from the figure. The (003) peak indicates the basal reflection of an interlayer anion in LDH materials. The first peak of the doublet close to a 2θ of approximately 60 is due to the orientation from the (110) planes, and its spacing corresponds to the lattice parameter a, which coincides with the closest M–M distance in the brucite-like layers. The unit cell parameters a and c can be calculated from the positions of these reflections: a = 2d110 and c = 3d003. The obtained parameters are presented in . These lattice parameters agree well with those reported for hydrotalcite-like compounds [Citation34]. The interlamellar distances (d003) of the samples were found to be 7.57, 8.84, 8.86 and 11.16 for Zn-Al-CO3, Zn-Al-NO3, Zn-Al-Cl and Zn-Al-SO4, respectively. The difference in the values of the interlamellar distances is evidence of the size and charge of the corresponding anions intercalated between the layers.
Table 2 Unit cell parameters and interlamellar distance of Zn-Al-LDHs.
3.1.2 FTIR
The FTIR spectra of the LDHs shown in reveal bands characteristic of hydrotalcite-like compounds. The broad and strong band centred at 3400 cm−1 is attributed to the stretching of the OH bond of the hydroxyl groups and H2O molecules. The weak band at 1630 cm−1 can be assigned to the H2O bending vibration of the interlayer water. The bands recorded at 460, 550, and 790 cm−1 are ascribed to the Al-O condensed groups, the Zn/Al-OH translation and the Al-OH deformation, respectively. For the Zn-Al-CO3 sample, the band around 1365 cm−1 can be attributed to the anti-symmetric stretching mode (ν3) of carbonate, and bands observed around 870 and 680 cm−1 are attributed to the weak non-planar bending mode (ν2) and the angular bending mode (ν4) of carbonate, respectively. The FTIR spectra of Zn-Al-NO3 reveal a strong peak around 1380 cm−1 attributed to the anti-symmetric stretching mode (ν3) of the nitrate anion present in the LDH [Citation35]. The bands observed around 839 and 670 cm−1 can be attributed to the weak out-of-plane symmetric deformation mode (ν2) and the anti-symmetric deformation mode (ν4) of nitrate, respectively. The distortion of Cl− in the interlayer of Zn-Al-Cl is reflected in the IR spectra with both ν3 and ν4 bands split at 1100–1145 cm−1 and 626–635 cm−1, respectively. For Zn-Al-SO4, the band observed at 1112 cm−1 can be attributed to the stretching mode (ν3) of sulphate, and the weak bands observed around 619 and 989 cm−1 can be attributed to the weak bending modes (ν2) and the symmetric stretching mode (ν1), respectively, for the sulphate anion present in the LDH [Citation35].
3.1.3 TEM observation
presents TEM images of different LDH samples. As shown, the Zn-Al-CO3 and Zn-Al-SO4 samples consisted of hexagonal platelets having some small cracks at the edge of the hexagonal sheets and part of the sheets presenting a vertical crossing at the hexagonal sides. The particle size varied from 20 to 150 nm. The spherical particles can be observed in Zn-Al-NO3. The TEM micrograph of the Zn-Al-Cl sample indicates the formation of agglomerates. This difference in the size and morphology could be attributed to the nature and amount of the interlayer anions.
3.1.4 TGA-DTA analysis
The thermogravimetric analysis of the synthesised LDHs is shown in . All of the TGA-DTA patterns are characterised by a weight loss between 10 and 14% due to the loss of the interlayer water in the temperature range of 50–250 °C. For Zn-Al-CO3, the dehydration takes place in two steps, at 150 and 250 °C. These steps can be interpreted as resulting from the loss of adsorbed and interlayer water followed by water loosely coordinated to the interlayer carbonate. The interlayer carbonate is released as CO2 at approximately 350 °C. The total mass loss was 20.86%. For Zn-Al-NO3, there are mass losses at 150, 250, 350 and 450 °C. The mass losses at 150 and 250 are accompanied by a change in the heat flow; it should result from the removal of the adsorbed surface water and the interlayer water. The second distinct mass loss region of 350–460 °C resulted from two processes, namely the dehydroxylation of the Zn-Al-LDH layers and the decomposition of the interlayer NO3− anions. The total mass loss was 21.5%. TGA-DTA analysis for Zn-Al-Cl shows a similar dehydration behaviour to that of Zn-Al-NO3, but Cl was lost in the temperature range of 400–500 °C. The total mass loss at 700 °C was 18.84%. The decomposition of Zn-Al-SO4 occurred in the following steps: the first mass loss at 150 °C corresponded to the evaporation of surface-adsorbed water followed by evaporation of the interlayer water at 250 °C; a third loss of mass occurring at 350 °C is attributed to the dehydroxylation of the brucite-like octahedral layers. The fourth step at 600 °C was likely due to the elimination of the intercalated SO42− in the Zn–Al LDH interlayers.
3.2 Effect of solution pH on the adsorption of MO
Generally, pH is considered to be an important parameter controlling the adsorption at the water-adsorbent interface. Adsorption experiments were carried out under the same experimental conditions by varying the solution pH from 2 to 12. The obtained results are illustrated in . From the figure, it can be observed that the amounts of MO adsorbed at equilibrium (qe) were higher under acidic conditions. The maximum adsorption was observed at a pH of approximately 4.5 and steadily decreased as the solution pH increased. The equilibrium was difficult to establish in alkaline conditions because of the competition between the excess OH− in the solution and the anionic molecules of MO. However, under acidic conditions, the equilibrium was easy to establish because the rate of adsorption strongly depends on the chemical adsorption effect. The dissociation constant (pKa) of the MO is 3.46; therefore, the monovalent anions of MO were predominantly present at these equilibrium pHs. Furthermore, the structure of the LDH materials was destroyed when the pH was below 4 [Citation36]. In this case, the adsorption of MO strongly decreased.
3.3 Kinetics
The variation with contact time in the amounts of MO adsorbed is shown in . The figure indicates that the adsorbed amounts increased rapidly in the first hour. Then, it slowed until equilibrium was established after 4 h for Mg-Al-CO3 and Mg-Al-Cl and 5 h for Mg-Al-NO3 and Mg-Al-SO4. The figure also indicates that the adsorption affinity increased in the following order: Zn-Al-CO3, Zn-Al-NO3, Zn-Al-Cl and Zn-Al-SO4. This result correlates with the differences in the structural properties of each LDH, essentially the interlayer spacing, as confirmed by XRD analysis.
Fig. 6 Effect of contact time on the adsorption of MO by LDHs (C0 = 20 mg/L; V = 250 mL; m = 20 mg; agitation speed = 500 rpm; T = 25 °C, pHsol = 8).
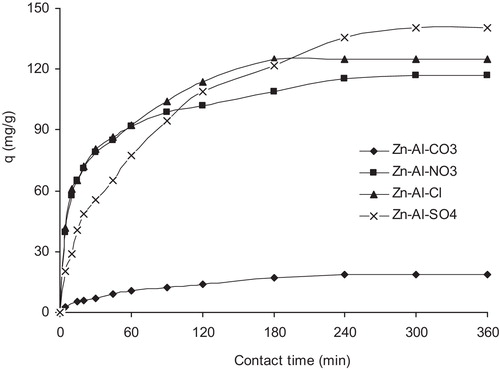
To characterise the kinetics involved in the process of adsorption, pseudo-first-order and pseudo-second-order kinetic models were proposed, and the kinetics data were analysed based on the regression coefficient (r2) and the amount of dye adsorbed per unit weight of the adsorbent.
The first-order rate expression of Lagergren based on the solid capacity is generally expressed as follows [Citation37]:(2)
(2) where qe and q (both in mg/g) are, respectively, the amounts of dye adsorbed at equilibrium and at any time ‘t’, and k1 (1/min) is the adsorption rate constant.
The pseudo-second-order model proposed by Ho and McKay [Citation38] was used to explain the adsorption kinetics. This model is based on the assumption that the adsorption follows a second-order chemisorption mechanism. The pseudo-second-order model can be expressed as:(3)
(3) where k2 (g/mg min) is the rate constant of the pseudo-second-order adsorption.
Parameters of the pseudo-first-order and pseudo-second-order models were estimated with the aid of a non-linear regression. The obtained data and the correlation coefficients, r2, are given in . The table shows that the correlation coefficient for the pseudo-second-order kinetic model is closer to unity than that of the pseudo-first-order models. From these results, it was concluded that the pseudo-second-order model was better than the Lagergren first-order model for the systems investigated in this work. Because ion adsorption follows pseudo-second-order kinetics, this suggested that the boundary layer resistance was not the rate-limiting step [Citation36]. The rate of MO adsorption may be controlled largely by a chemisorption process in conjunction with the chemical characteristics of the LDHs and MO molecules. A similar phenomenon is observed in the adsorption of methyl orange onto Mg/Ni-Al layered double hydroxides [Citation39].
Table 3 Kinetic constants for methyl orange adsorption onto LDHs.
3.4 Adsorption isotherms
The isotherms of the adsorption of methyl orange by LDHs are shown in . They all display the typical “L” shape according to Giles's classification [Citation40]. MO is highly concentration dependent. The increase in the adsorption capacity with the dye concentration in the solution is probably due to a high driving force for the mass transfer. In fact, a high concentration in the solution implicates a large number of dye molecules fixed at the surface of the adsorbent. The Langmuir, Freundlich and Dubinin Redushkevich isotherm models were applied for the analysis of the obtained equilibrium sorption data.
The Langmuir adsorption model is based on the assumption that the maximum adsorption corresponds to a saturated monolayer of solute molecules on the adsorbent surface. Therefore, The Langmuir isotherm assumes that sorption comes from the monolayer coverage of the adsorbate over a homogenous adsorbent surface [Citation41]. This model supposes that sorption occurs on specific homogeneous sites within the adsorbent and that all of the sorption sites are energetically identical. The Langmuir isotherm can be written in the following form:(4)
(4) where qm (mg/g) is the maximum amount of dye adsorbed per unit mass of adsorbent, and KL (L/mg) is the Langmuir constant related to the rate of adsorption. Ce is the equilibrium concentration.
The Freundlich isotherm is an empirical model of a heterogeneous surface with a non-uniform distribution of heat sorption and affinities by means of multilayer adsorption [Citation42].
The form of the Freundlich equation can be stated as follows:(5)
(5) where KF (mg1−1/n/g/Ln) and n are Freundlich constants, n indicates whether the adsorption process is favourable, and KF is related to the adsorption capacity of the adsorbent.
The Dubinin–Radushkevich isotherm model does not assume a homogenous surface or a constant sorption potential, as other models do. It can be noted that this isotherm model is more general than the Langmuir model [Citation43]. The Dubinin–Radushkevich isotherm has been written by the following equations:(6)
(6)
(7)
(7) where B is a constant related to the adsorption energy, qmax is the theoretical saturation capacity, and ɛ is the Polanyi potential.
A non-linear regression is used to determine the best-fitting isotherm, and the applicability of the isotherm equations is compared by comparing the correlation coefficients, r2. shows the obtained results. The table indicates that the Langmuir equation gives the best satisfactory fitting to the adsorption isotherms of the MO adsorption onto the LDH samples with higher correlation coefficients than the others, indicating the homogeneous nature of all the surfaces and the formation of a monolayer of methyl orange molecules on the surface of the adsorbents. The Langmuir maximum adsorption capacities were 2758, 2455, 2270 and 1684 mg/g for Zn-Al-SO4, Zn-Al-Cl, Zn-Al-NO3 and Zn-Al-CO3, respectively.
Table 4 Isotherm models constants for the MO adsorption onto LDHs.
The adsorption capacities decreased in the following order: Zn-Al-SO4 > Zn-Al-Cl > Zn-Al-NO3 > Zn-Al-CO3. This behaviour can be attributed to the peculiar structural properties of each LDH. As confirmed by XRD, the interlayer spacing decreased in the same order. The main mechanism for the MO adsorption on the LDHs could be the ion exchange between the MO anions and previously intercalated anions, although the surface adsorption is also presumed to be involved [Citation44].
4 Conclusion
Zn–Al layered double hydroxides intercalated with CO32−, NO3−, Cl− or SO42− were successfully prepared via a co-precipitation method. The presence of the anions in the hydrotalcite structure was confirmed by infrared spectroscopy. The XRD analyses showed that the interlayer spacing followed this order: Zn-Al-SO4 > Zn-Al-Cl > Zn-Al-NO3 > Zn-Al-CO3. All TGA-DTA curves are characterised by a weight loss between 10 and 14% due to a loss of interlayer water in the temperature range of 50–250 °C. The maximum adsorption capacity of MO was obtained in an acidic pH medium. The equilibrium adsorption was increased by increasing the initial dye concentration in the solution. Adsorption kinetics data fitted well to a pseudo-second-order kinetic model. The adsorption equilibrium data fitted best to the Langmuir isotherm model. The adsorption affinity correlated with the structural properties, essentially the interlayer spacing, in each LDH. The precursors exhibited exceptional adsorption capacities indicating that these materials could be a potentially efficient broad-spectrum adsorbent for dye removal from wastewater. With respect to the selection of sorbents, these results are of practical interest for the optimisation of aquatic environment remediation technologies.
Notes
Peer review under responsibility of Taibah University.
References
- K.ItohY.KitadeC.YatoteA pathway for biodegradation of an anthraquinone dye, C.I. Disperse Red 15, by a yeast strain Pichia anomalaBull. Environ. Contam. Toxicol.561996413418
- H.M.PinherioE.TouraudO.TomasAromatic amines from azo dye reduction: status review with emphasis on direct UV spectrophotometric detection in textile industry wastewaterDyes Pigments612004121139
- N.BarkaS.QourzalA.AssabbaneA.NounahY.Ait-IchouTriphenylmethane dye, patent blue V, photocatalytic degradation on supported TiO2: kinetics, mineralization and reaction pathwayChem. Eng. Commun.198201112331243
- T.SuzukiS.TimofeiL.KuruncziU.DietzeG.SchuurmannCorrelation of aerobic biodegradability of sulfonated azo dyes with the chemical structureChemosphere45200119
- T.RobinsonG.McMullanR.MarchantP.NigamRemediation of dyes in textile effluent: a critical review on current treatment technologies with a proposed alternativeBioresour. Technol.772001247255
- J.ShoreAdvances in direct dyesIndian J. Fibre Text. Res.211996129
- A.B.D.SantosF.J.CervantesJ.B.V.LierReview paper on current technologies for decolorisation of textile wastewaters: perspectives for anaerobic biotechnologyBioresour. Technol.98200723692385
- A.AbaamraneS.QourzalN.BarkaS.Mançour-BillahA.AssabbaneY.Ait-IchouOptimal decolorization efficiency of indigo carmine by TiO2/UV photocatalytic process coupled with response surface methodologyOrient. J. Chem.283201210911098
- M.V.SureshkumarC.NamasivayamAdsorption behavior of Direct Red 12B and Rhodamine B from water onto surfactant-modified coconut coir pithColloids Surf. A: Physicochem. Eng. Aspects3172008277283
- E.Alventosa-deLaraS.Barredo-DamasE.Zuriaga-AgustíM.I.Alcaina-MirandaM.I.Iborra-ClarUltrafiltration ceramic membrane performance during the treatment of model solutions containing dye and saltSep. Purif.129201496105
- K.RavikumarS.KrishnanS.RamalingamK.BaluOptimization of process variables by the application of response surface methodology for dye removal using a novel adsorbentDyes Pigments7220076674
- M.GhaediA.Golestani NasabS.KhodadoustR.SahraeiA.DaneshfarCharacterization of zinc oxide nanorods loaded on activated carbon as cheap and efficient adsorbent for removal of methylene blueJ. Ind. Eng. Chem.212015986993
- M.AramiN.Y.LimaeeN.M.MahmoodiaEvaluation of the adsorption kinetics and equilibrium for the potential removal of acid dyes using a biosorbentChem. Eng. J.1392008210
- N.BarkaA.AssabbaneY.Aît IchouA.NounahDecontamination of textile wastewater by powdered activated carbonJ. Appl. Sci.632006692695
- M.A.AhmadN.A.Ahmad PuadO.S.BelloKinetic, equilibrium and thermodynamic studies of synthetic dye removal using pomegranate peel activated carbon prepared by microwave-induced KOH activationWater Resour. Ind.620141835
- R.ElmoubarkiF.Z.MahjoubiH.TounsadiJ.MoustadrafM.AbdennouriA.ZouhriA.ElAlbaniN.BarkaAdsorption of textile dyes on raw and decanted Moroccan clays: kinetics, equilibrium and thermodynamicsWater Resour. Ind.920151629
- F.-C.TsaiN.MaT.-C.ChiangL.-C.TsaiJ.-J.ShiY.XiaT.JiangS.-K.SuF.-S.ChuangAdsorptive removal of methyl orange from aqueous solution with crosslinking chitosan microspheresJ. Water Process Eng.1201427
- M.Zarezadeh-MehriziA.BadieiHighly efficient removal of basic blue 41 with nanoporous silicaWater Resour. Ind.520144957
- M.DoganM.H.KaraogluM.AlkanAdsorption kinetics of maxilon yellow 4GL and maxilon red GRL dyes on kaoliniteJ. Hazard. Mater.165200911421151
- M.DoganM.AlkanAdsorption kinetics of methyl violet onto perliteChemosphere502003517528
- Y.OzdemirM.DoganM.AlkanAdsorption of cationic dyes from aqueous solutions by sepioliteMicroporous Mesoporous Mater.2006419427
- A.H.GemeayA.S.El-SherbinyA.B.ZakiAdsorption and kinetic studies of the intercalation of some organic compounds onto Na+-montmorilloniteJ. Colloid Interface Sci.2452002116125
- N.BarkaK.OuzaouitM.AbdennouriM.El MakhfoukDried prickly pear cactus (Opuntia ficus indica) cladodes as a low-cost and eco-friendly biosorbent for dyes removal from aqueous solutionsJ. Taiwan Inst. Chem. Eng.4420135260
- F.CavaniF.TrifirbA.VaccariHydrotalcite-type anionic clays: preparation properties and applicationsCatal. Today111991173301
- V.RivesLayered Double Hydroxides: Present and Future2001Nova Science Publishers, Inc.New York
- F.LiX.DuanApplications of layered double hydroxidesStruct. Bond.11912005193223
- D.G.EvansX.DuanPreparation of layered double hydroxides and their applications as additives in polymers, as precursors to magnetic materials and in biology and medicineChem. Commun.52006485496
- S.MandalS.MayadeviAdsorption of fluoride ions by Zn–Al layered double hydroxidesAppl. Clay Sci.4020085462
- T.IshikawaK.MatsumotoK.KandoriT.NakayamaAnion-exchange and thermal change of layered zinc hydroxides formed in the presence of Al(III)Colloids Surf. A: Physicochem. Eng. Aspects2932007135145
- J.W.BoclairP.S.BratermaLayered double hydroxide stability. 1. Relative stabilities of layered double hydroxides and their simple counterpartsChem. Mater.111999298302
- C.D.HoyoLayered double hydroxides and human health: an overviewAppl. Clay Sci.362007103121
- J.DasD.DasK.M.ParidaPreparation and characterization of Mg–Al hydrotalcite-like compounds containing ceriumJ. Colloid Interface Sci.302006569574
- S.V.PrasannaP.V.KamathChromate uptake characteristics of the pristine layered double hydroxides of Mg with AlSolid State Sci.102008260266
- J.T.KloproggeD.WhartonL.HickeyR.L.FrostInfrared and Raman study of interlayer anions CO32−, NO3−, SO42− and ClO4− in Mg/Al-hydrotalciteAm. Miner.872002623
- I.D.MallV.C.SrivastavaN.K.AgarwalRemoval of Orange-G and Methyl Violet dyes by adsorption onto bagasse fly ash kinetic study and equilibrium isotherm analysesDyes Pigments692006210223
- J.DasB.S.PatraN.BaliarsinghK.M.ParidaAppl. Clay Sci.322006252
- S.LagergrenAbout the theory of so-called adsorption of soluble substance Seven VetenskapsakadHandband241898139
- Y.S.HoG.McKayPseudo-second order model for sorption processesProcess Biochem.341999451465
- H.Zaghouane-BoudiafM.BoutahalaL.ArabRemoval of methyl orange from aqueous solution by uncalcined and calcined MgNiAl layered double hydroxides (LDHs)Chem. Eng. J.1872012142149
- C.H.GilesT.H.Mac EwanS.N.NakhwaP.SmithStudies on adsorption – XI. A system of classification of solution adsorption isotherms and its use in diagnosis of adsorption mechanisms and in measurement of specific surface areas of solidsJ. Chem. Soc.111196039733993
- I.LangmuirThe constitution and fundamental properties of solids and liquidsJ. Am. Chem. Soc.38191622212295
- H.FreundlichW.HellerThe adsorption of cis- and trans-azobenzeneJ. Am. Chem. Soc.61193922282230
- M.M.DubininL.V.RadushkevichThe equation of the characteristic curve of the activated charcoalProc. Acad. Sci. USSR Phys. Chem. Sect.551947331337
- E.L.CrepaldiJ.TrontoL.P.CardosoJ.B.ValimSorption of terephthalate anions by calcined and uncalcined hydrotalcite-like compoundsColloid Surf. A: Physicochem. Eng. Aspects2112002103114