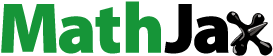
Abstract
A newly isolated fungus, Aspergillus terreus NCFT4269.10, was employed in both solid state (SSF) and liquid static surface culture (LSSC) for the production of protease using different agro-residues. Among different substrates appraised, chickling vetch peels (CVP) supported the enhanced production of protease both at LSSC and SSF (499.99 ± 11 U/ml; 5266.8 ± 202.5 U/gds, respectively). In the presence of peptone (1%, w/v), leucine (5 mM/100 ml), Fe2+ (1 mM) and riboflavin (10 mg/100 ml) with a medium pH of 5.0 incubated at 30 °C for 96 h, 3-fold higher protease production was achieved in LSSC compared with control. Fermentation kinetics studies revealed that the highest specific growth rate of A. terreus was observed in fermentation medium supplemented with riboflavin (10 mg/100 ml), i.e., 256.45 mg l−1 h−1. The growth-associated coefficient of enzyme production (α) by A. terreus was maximal when protease was produced using Fe2+. Further, the protease was purified to electrophoretic homogeneity and its molecular mass was determined as 23.8 kDa. The present strain suggests the potential utilization of inexpensive agro-residues (CVP) as medium components for the efficient industrial production using LSSC.
1 Introduction
As high-flying industrial enzymes, proteases catalyze the hydrolysis of peptide bonds present in proteins and can be categorized into two extensive groups, exopeptidases and endopeptidases, owing to the site of action. Depending on the presence of amino acids at the active site, these proteases can be further classified as serine, threonine, cysteine and aspartate proteases; metalloproteases; and glutamic acid proteases. Nonetheless, proteases can also be grouped into three types depending on their pH optima, i.e., acid proteases: pH optimum below 7.0 (2.0–5.0), alkaline proteases: pH optimum above 7.0 and neutral proteases: pH optimum 7.0. The multifarious proteases of different types exhibit specificity in substrate, active site, catalytic mechanism, pH, temperature optima and stability profiles. Studies concerning the properties of these proteases are vital for the successful exploitation of biocatalysts in industries [Citation1], such as detergents, leather processing, silver recovery [Citation2], pharmaceuticals, food and feed processing, chemical industries, waste treatment [Citation3] and amino acid mixtures resolution, with features for biotechnological applications [Citation4]. Commercially, proteases are the most significant industrial enzymes, accounting for approximately 60% of the total global trade of enzymes [Citation5].
Proteases of protozoa, bacteria, yeast and fungal origins degrade complex proteins for nutrition [Citation6]. Nonetheless, proteases from fungal genera, including Aspergillus, Penicillium, Cephalosporium, Mucor, Candida, Neurospora and Rhizopus, have industrial significance. Amongst the many filamentous fungi, proteases from Aspergillus spp. have extensively been exploited in diverse industries, reflecting the activity of these enzymes at various pH levels and temperatures [Citation7]. Acid proteases are produced from Mucor miehei, Mucor hiemalis, Mucor racemosus and Mucor bacilliformis [Citation8]. Similarly, pepsin-like acid proteases are produced from Aspergillus [Citation9] and Rhizopus species [Citation10].
Fermentation expenses for the production of “bulk-ware” proteases under most conditions supports solid-state fermentation (SSF) compared with submerged fermentation (SmF), as SSF employs intricate, heterogeneous agricultural residues as substrates that are plentiful, economical and functional with inexpensive technology concerning sterility and regulation demands. Surprisingly, the process and downstream factors (constancy of the secreted enzymes at high temperature or extreme pH) have also been documented as superior in SSF [Citation11]. Catabolite repression or protein degradation through proteases, an unavoidable dilemma in SmF is often reduced or absent in SSF. Furthermore, filamentous fungi are the finest tailored microorganisms for solid-state fermentation (SSF), reflecting the hyphal mode of fungal growth, tolerance to low water activity, high osmotic pressure conditions and competitiveness of these organisms in natural microflora for the bioconversion of solid substrates.
Thus, the aim of the present study was to determine the production and optimization of the fermentation and its purification conditions for proteases for significant employment in various industrial processes.
2 Materials and methods
2.1 Substrates and chemicals
In the present study, different agro-substrates were procured from the locality of Bhubaneswar, Odisha and transported in new steel containers to the laboratory. These substrates include mustard oil cake (MoC), neem oil cake (NoC), groundnut oil cake (GnoC), black gram peels (BGP), green gram peels (GGP), chickling vetch peels/grass pea peels (CVP), wheat bran (WB), pearl millet residues (PMR), finger millet waste (FMW), broken rice (BR), banana peels (BP), apple pomace (AP) and orange peels (OP). These materials were dried at 60 °C for up to 48 h inside a hot air oven and powdered using a blender. Analytical reagent grade chemicals were purchased from Sigma, Hi-Media Limited, SRL Pvt. Limited and Merck India Limited.
2.2 Fungal spore inoculum preparation
The young slant culture of Aspergillus terreus NCFT 4269.10 (GenBank accession Number: KT222271.1), a protease-producing strain [Citation12], was used for subsequent experiments. The pre-fermentation culture of A. terreus NCFT 4269.10 was developed with a final spore concentration of 5.0 × 108 spores ml−1 at 30 ± 1 °C. Further, dilutions were obtained using double-sterilized water to generate a working spore concentration of 1 × 107 spores ml−1, as determined using a UV-VIS spectrophotometer [Citation13].
2.3 Screening for selection of suitable substrate
The screening of pre-processed substrates (10 g) was performed using liquid static surface culture (LSSC) and solid-state fermentation (SSF). These substrates were used as the principal media components and 50 ml of sterilized medium formulated with either MoC, NoC, GnoC, BGP, GGP, CVP, WB, PMR, FMW, BR, BP, AP or OP as substrates inoculated with 1 × 107 spores ml−1 from a seven-day-old pre-fermentation culture broth and incubated at 30 ± 1 °C under static conditions. Similarly, solid-state fermentation was performed using the above substrates (5 g, w/w) under the conditions described above. After fermentation, both fermented media (LSSC and SSF) were processed for the recovery of crude protease and subsequently stored at −20 °C until further analysis. The biomass formed during LSSC was oven dried at 80 °C for up to 24 h to determine the dry weight.
2.4 Optimization of the fermentation parameters for enhanced protease production
Environmental, nutritional and other fermentation parameters were established for the enhanced production of protease using CVP as the fermentation medium through SSF and LSSC. The optimized process parameters were pH (3–10), temperature (24–45 °C at 3 °C interval), incubation time (24–168 h), additional carbon sources (1.0%, w/w), nitrogen sources (organic and inorganic, 1.0%, w/w), amino acids (1.0 mM), metal ions (1.0 mM), antioxidant vitamins (10–50 mg/100 ml), growth hormones (0.0025 mg/g, w/w), combined agro-wastes, inoculum size (2–10%) and initial moisture content (20–100%). The effect of soaking time on the fermented products (1–96 h), repeated extraction and various extractants were also standardized for the easy, proficient and economic down streaming of the protease. The adopted approach was to scale up one variable-at-a-time (OVAT), independent of the other factors and consequently, the established conditions were adopted in subsequent experiments.
2.5 Purification of the protease
The crude protease (∼500 ml) was precipitated through the gradual addition of 40–80% ammonium sulphate [(NH4)2SO4] with constant stirring using a magnetic stirrer at 4 °C for up to 24 h. Each precipitate was separated from the supernatant through centrifugation at 10,000 × g for 15 min at 4 °C. After centrifugation, the supernatant was decanted, and the solid precipitate was dissolved in phosphate buffer (pH 6.5) at a ratio of 0.1 g/ml to obtain 10-times more concentrated enzyme solution [Citation14]. Thereafter, the ammonium sulphate-precipitated enzyme solution was dialyzed for 24 h at 4 °C with constant stirring against a large volume (1 L capacity) of phosphate buffer (pH 6.5) for the complete removal of lower molecular weight metabolites and ammonium sulphate from the dialysate. To improve the solute exchange, the dialysis buffer was replaced every 2 h to obtain a new concentration gradient. Subsequently, the dialyzed enzyme was loaded onto a Sephadex G-100 column (2.5 cm × 70 cm) and eluted with 50 mM phosphate buffer (pH 6.5) at a flow rate of 1 ml min−1. Fractions of 2 ml each were subsequently collected to estimate the protein concentration [Citation15]. The fractions showing maximum absorption at 750 nm were collected and evaluated for enzyme activity. The enzyme-positive fractions with higher enzyme activity were pooled, lyophilized and stored at −20 °C until further characterization.
2.6 Analytical methods
2.6.1 Total protein determination
The total protein content of the crude and purified protease was estimated [Citation15], using bovine serum albumin as the standard and expressed as μg of protein per ml of extract obtained after fermentation and purification.
2.6.2 Protease assay
Extracellular protease activity was determined according to the method of van den Hombergh et al. [Citation16]. Each 450-μl sample was incubated with 50 μl of 1% (w/v) BSA (Sigma) in 0.1 M sodium acetate buffer (pH 4.0) at 37 °C. After 30 min of incubation, the reaction was terminated with 500 μl of 10% (w/v) trichloroacetic acid (TCA). After incubation at 0 °C for 30 min, the precipitated proteins were removed through centrifugation at 6000 rpm for 5 min and the absorbance of the TCA-soluble fraction was estimated as per Lowry et al. [Citation15]. One unit (U) of protease activity was defined as the amount of enzyme that releases 1 μg of tyrosine in 1 min under the assay conditions.
2.6.3 SDS-PAGE and zymographic analysis of protease
The samples (crude and purified protease) were electrophoresed using 10% SDS-PAGE for molecular weight determination [Citation17]. For visualization of the bands, the gels were stained in a staining solution [0.1% (w/v) Coomassie-Brilliant Blue R-250, 50% (v/v) methanol, 7% (v/v) glacial acetic acid and 43 ml MilliQ-distilled water] for 90 min at room temperature, followed by destaining with 30% (v/v) methanol, 7% (v/v) acetic acid and 63 ml MilliQ-distilled water until the background was clear. The relative positions of the bands were analyzed using a Bio-Rad Gel documentation system. Different molecular weight protein markers ranging from 7 to 175 kDa (Bangalore Genei Ltd.) were used for SDS-PAGE.
For the in-gel activity assay, a 10% non-denaturing gel containing 0.1% casein (casein was incorporated into the resolving gel prior to the addition of APS and polymerization) was run for 8–12 h in running buffer (50 mM Tris-0.1 M glycine, pH 8.3) at 4 °C with a 20 mA current. After running, the gel was rinsed in 0.25% Triton-X for 1 h and incubated 2 h to facilitate proteolysis in 0.1 M Tris-HCl buffer (pH 9) at 50 °C. The gel was stained for 1 h in a solution of Brilliant Blue R-250 and subsequently destained with methanol–acetic acid–water (4:1:5) until the background became clear [Citation18]. The gel image was captured in a Bio-Rad Gel doc system.
2.7 Fermentation kinetics study of protease
For fermentation using agro-wastes from A. terreus, the logistic Eq. Equation(A.1)(A.1)
(A.1) and the Luedeking–Piret model (Eq. Equation(B.2)
(B.2)
(B.2) ) were employed for microbial growth and enzyme production, respectively.
(A.1)
(A.1)
(B.2)
(B.2) where dx/dt: biomass accumulation in the culture medium (g l−1 h−1); dP/dt: enzyme accumulation in the culture medium (U ml−1 h−1); x: biomass (mg ml−1) at time (t); μ: specific growth rate (h−1); μmax: highest specific growth rate observed during batch culture (mg l−1 h−1); xmax: maximum attainable biomass (mg ml−1); α: growth-associated coefficient of enzyme production (U g−1); β: growth-independent coefficient of enzyme production (U g−1 h−1).
2.8 Statistical data analysis
All experiments were performed in triplicate (n = 3) and repeated three times. The samples collected from each replicate were analyzed for enzyme production, including enzyme activity and the optimization (scaled up) of the culture conditions. Each value represents an average of three parallel replicates. The ± and error bars indicate standard deviation among the replicates. For each individual experiment, one-way ANOVA was calculated using SPSS 16.0 workbook software. Least significant differences were also calculated using Duncan's new multiple range tests.
3 Results and discussion
3.1 Screening and selection of a suitable substrate
For the economical production and recovery of enzymes, many fermentation trends has been altered and process parameters have been optimized. Furthermore, much focus has been given on microbial enzymes compared with enzymes of plant or animal origins, reflecting the availability of an immense array of catalytic activities, promising lofty yields, effortlessness in genetic manipulation, continuous production due to lack of seasonal fluctuations and swift growth of microorganisms on economic fermentation media. There are 250 officially documented species in the filamentous fungal genus Aspergillus [Citation19], of which a variety of fungal strains, including Aspergillus flavus, Aspergillus niger [Citation20], Aspergillus clavatus [Citation21], Aspergillus oryzae [Citation22], A. terreus [Citation13], Aspergillus awamori [Citation23] and Aspergillus fischeri [Citation24], were reported to secrete significant quantities of extracellular proteases and other enzymes.
Traditionally, both SmF and SSF have been employed for the production of proteases, but SSF has been preferred and demonstrated as comparatively better than SmF, reflecting the varying environmental and nutritional advantages of this method. Recently, attention has been focused on the effective utilization of solid agricultural by-products for the production of industrially imperative enzymes. Consistent with studies aimed at the efficient production of high-valued, low-cost proteases, various economical agro-residues (MoC, NoC, GnoC, BGP, GGP, CVP, WB, BR, PM, FM, BP, AP and OP) were examined for the production of protease. Amongst all substrates, chickling vetch peels (CVP) were the most suitable substrate for the production of protease through LSSC and SSF (499.99 ± 11 U/ml; 5266.8 ± 202.5 U/gds) (). However, all substrates supported the production of protease. Similarly, rice [Citation25], wheat bran [Citation26] and other agro-industrial by-products were also utilized for the production of protease.
Table 1 Protease production from Aspergillus terreus NCFT4269.10 in liquid static surface culture and solid-state culture systems using various agricultural wastes as substrates.
3.2 Culture amendment for enhanced production of protease
3.2.1 Effects of initial medium pH
To optimize the pH of the fermentation medium for the enhanced production of protease from A. terreus NCFT 4692.10, the initial medium pH ranging from 3.0 to 10.0 was adjusted and fermentation was performed. The results showed that pH 5.0 was optimal for the production of this enzyme. The pH positively influenced biomass production in case of protease, whereas the synthesis of enzymes was reduced with increasing pH (a). However, the protease was produced at all pH values, indicating that protease production is pH stable. The final pH of the medium has drastically changed. In addition, the final pH of the medium remained the same with respect to initial pH 6.0. Hence, the secreted enzyme was an acidic protease. A. niger produced proteases at all pH ranges (4.4–9.0), after which the production ceased, consistent with the findings of previous studies [Citation20]. Similar studies on the influence of pH on protease production have been conducted in A. terrus between pH 5.5 and 9.5 [Citation27]. Enzyme secretion from microbial strains strongly depends on the extracellular pH, as the culture pH strongly influences many enzymatic reactions.
Fig. 1 (a) Effect of pH (3.0–10.0) and temperature (24–45 °C at 3 °C interval) on protease production and mycelia growth in liquid static surface culture (LSSC) in A. terreus NCFT 4269.10 (for pH, biomass of A. terreus: ▴; protease: ♦; for temperature, biomass of A. terreus: ○; protease: ▵). (b) Effect of incubation time (24–144 h) on protease production and mycelia growth in liquid static surface culture (LSSC) in A. terreus NCFT 4269.10. (c) Effect of various carbon and nitrogen sources on the mycelia growth and protease production in A. terreus, where, CON: Control; GLU: Glucose; MAL: Maltose; LAC: Lactose; MANI: Mannitol; SUC: Sucrose; STA: Starch; CEL: Cellulose; FRU: Fructose; GAL: Galactose; MAN: Mannose; APS: Ammonium persulphate; AN: Ammonium nitrate; AS: Ammonium sulphate; AC: Ammonium chloride; SN: Sodium nitrate; UR: Urea; PEP: Peptone; TRY: Tryptone; GLY: Glycine, GEL: Gelatin; YE: Yeast extract and BE: Beef extract).
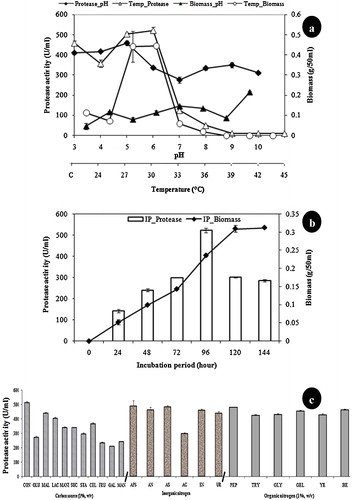
3.2.2 Effect of temperature
LSSC was performed at 24–45 °C (at an interval of 3 °C) using 1 × 107 spores as inoculum for 96 h to evaluate the effect of incubation temperatures on the generation of mycelial biomass and the production of protease. The temperature strongly influences enzyme and biomass production. The optimum temperature for the production of protease was 30 °C (a). Temperatures of 30 °C, 35 °C and 37 °C have been determined as optimal for protease production in cases of Aspergillus saitoi, Aspergillus tamari, Aspergillus nidulans and A. awamori, respectively [Citation28,Citation23]. Nevertheless, the assessment of enzyme activities in numerous microorganisms is complicated, although discrete culture parameters and enzyme activity determinations have been employed [Citation29].
3.2.3 Effect of fermentation duration on production of protease
At optimized pH and temperature conditions, LSSC was performed at varying fermentation durations for the production of protease. The production of protease was initiated at 24 h and increased for up to 96 h, displaying a gradual decline thereafter. The biomass was substantially increased after 24 h and continued for up to 144 h, with maximum enzyme production at 96 h that subsequently remained stable before showing a decline in the growth profile (b). The effect of the incubation period on protease production in A. terreus was consistent with the results of Negi and Banerjee [Citation23] using A. awamori. Chimata et al. [Citation30] observed the production of maximum protease at 120 h from a newly isolated Aspergillus species when wheat bran, rice bran and green gram husk were employed as substrates. Paranthaman et al. [Citation31] reported that from A. niger, maximum protease production was observed after incubation for 72 h in SSF using rice bran as the substrate, which differs from the present study. These differences might reflect the dependency on factors regulating the growth and production of enzymes in the medium. The optimization of the incubation regimen is an obligatory stride in making the production of industrial enzymes economically feasible.
3.2.4 Effect of carbon sources on the production of protease
Amongst all carbon sources supplemented with the CVP fermentation broth, none has participated in enhancement of protease production compared with the control (c). Negi and Banerjee [Citation23] reported that the supplementation of lactose as a carbon source enhanced protease production. There are several reports on the utilization of diverse carbon sources and strain type that differentially influence the production of this extracellular enzyme [Citation32].
3.2.5 Influence of nitrogen sources on protease production
Different organic and inorganic nitrogen sources were individually supplemented into the fermentation medium and evaluated for the enhanced production of protease. The supplementation of peptone only favoured the highest protease production amongst all organic and inorganic nitrogen sources evaluated (c). Amongst the organic nitrogen substrates utilized, peptone supported protease yield, consistent with the results of Akhter et al. [Citation33].
3.2.6 Effect of amino acids on production of protease
In the present study, seventeen amino acids were supplemented into the CVP fermentation medium and the suitability for the production of protease was evaluated. The results showed that leucine at a concentration of 1 mM enhanced ∼1.5-fold synthesis of protease, whereas maximum biomass was attained with proline (). Similarly, cysteine, histidine and alanine also supported the production of protease.
Table 2 Effect of amino acids, metal ions, vitamins, growth regulators and mixed substrates on mycelia growth and protease production in A. terreus NCFT 4269.10Table Footnotea.
3.2.7 Effect of metal ions
Amongst the metal ions evaluated, Fe2+ significantly affects the enhanced production of protease (). It was also reported that this protease was activated through Ca2+, Fe2+ and Mg2+ [Citation34]. The role of metal ions in stimulating thermal stability might reflect the consequences of these molecules on the integrity of the enzyme conformation or alterations in the solvent polarity, ionic potency and balance that might alter the surface pH and/or active site conformation [Citation35].
3.2.8 Effect of vitamins on production of protease
The effect of four vitamins (Vitamin C, riboflavin, folic acid and Vitamin E) on the production of protease was tested at varying concentrations as shown in . The results of the present study showed that riboflavin at a concentration of 10 mg/100 ml exhibited ∼3-fold protease activity (1507.05 ± 84.14 U/ml) (). Afifi et al. [Citation36] also reported that vitamin C had the most pronounced effect on the growth, protein accumulation and amylase production in P. olsonii, which validates the demand of vitamin for the production of protease and growth of A. terreus.
3.2.9 Effect of plant growth regulators on production of protease
The effect of growth hormones (BAP, 2-4 D, Kinetin and gibberellic acid) on protease production was examined after supplementing with fermentation medium at a concentration of 0.025% (w/v). However, none of these growth regulators support enzyme secretion as compared with the control (data not shown). Negi and Banerjee [Citation23] also examined the effects of growth hormones on amylase and protease production and concluded that indol acetic acid (IAA) and indol-3-butyric acid (IBA) both stimulated the production of the enzymes produced from A. awamori. Negi and Banerjee [Citation23] indicated that naphthalene acidic acid (NAA) and 2-4, D enhanced protease production but reduced amylase production, which is not vis-a-vis to the findings of A. terreus.
3.2.10 Effect of mixed substrates on enzyme production
The synergistic effect of different combinations of agro-residues was assessed for the enhanced production of protease using the SSF method. The results showed that CVP:Pigeon pea peels at a ratio of 3:7 and CVP:horse gram peels at a ratio of 5:5 exhibited the highest protease production (). Similarly, Chutmanop et al. [Citation37] have reported that rice bran has reduced porosity for agreeable solid-state fermentation and could be more effective only when employed in combination with wheat bran for synthesis of protease from A. oryzae.
3.2.11 Effect of inoculum size
Effect of inoculum size on protease production revealed that a 2% (w/v) inoculum size was optimum for the production of protease (). In all cases, a further increase in the inoculum size resulted in decreased enzyme synthesis. Wu et al. [Citation38] suggested that a 5% inoculum level leads to the enhanced production of protease using A. terreus, whereas, in this present study, an inoculum size of 2% was suitable for the synthesis of protease. A further increase in inoculum size resulted in decreased enzyme synthesis. Studies have also shown that there was a decline in any production with increased inoculum size was associated with high biomass, which is responsible for the production in enzyme production under nutrient scarce conditions in fermentation mashes.
3.2.12 Effect of initial moisture content
As moisture content plays an important role in SSF and greatly affects the production; hence, the moisture content of the substrate was adjusted and fermentation was performed. A moisture content of 50% (w/w) was optimum for the production of protease (). A further increase in moisture content generated in a gradual decrease in protease activity. Similarly, 50% initial moisture content was optimal for protease production from P. godlewskii SBSS 25, indicating that the water-binding capacity of chickling vetch peels was low. High moisture content was not conducive for the mycelial growth of P. godlewskii SBSS 25, reflecting the agglomeration of substrate particles and thereby, reducing the oxygen level [Citation39].
3.3 Down streaming of protease
For the efficient and enhanced recovery of protease from solid fermented medium, different extractants and soaking times were examined. The results showed that soaking for 24 h at first extraction with MilliQ water was superior for the maximum recovery of protease compared with other extractants (). Aikat and Bhattacharyya [Citation26] reported that 0.6 M phosphate buffer (pH 8.0) was optimal for the recovery of protease from Rhizopus oryzae using wheat bran as the substrate with 72 h of soaking time at the first extraction. These authors also reported that Czapek Dox salt solution was better than distilled water. However, distilled water was more efficient in enzyme recovery compared with Czapek Dox solution, suggesting that different solvents can be used for the down streaming of enzymes. Until recently, the mechanism behind this hypothesis has not been clearly understood.
Table 3 Effect of soaking time, repeated extraction and various extractants on protease recovery.
3.4 Purification of protease
The cell-free dialyzed protease displayed 7904.28 U with a specific activity of 225.321 U mg−1 protein, representing 3.95% yield and 1.02-fold purification. The partially purified protease (dialized ammonium sulphate-precipitated fraction) was further fractionated through a column of Sephadex G100 and the maximum amount of protease, representing 1.6-fold purification (). The protease was eventually purified to 1.6-fold homogeneity, 3.08% yield and a specific activity of 344.1 U/mg using ammonium sulphate precipitation followed by Sephadex G-100 gel filtration chromatography (supplementary material). Similarly, Benazir et al. [Citation32] attained approximately 1.17-fold purification with a protease yield of 82.47% isolated from A. niger. Enzymes from A. oryzae KSK-3 were also concentrated through ultrafiltration and sequentially purified using ammonium sulphate precipitation and ion exchange and gel filtration chromatography according to Shirasaka et al. [Citation25].
Table 4 Purification summary of isolated protease.
3.5 Molecular weight determination through SDS-PAGE and zymographic analysis
Both crude and purified proteases were subjected to protein profiling and molecular weight determination. The crude protease displayed 13 bands within the range of 7–175 kDa (), whereas the purified protease displayed a single band with molecular weight of 23.8 kDa (). When the zymogram was prepared using casein, only a single hydrolyzed band was observed. Analyses through SDS-PAGE and zymography for the production of protease from different fungal species were performed. Variations in molecular weights have also been reported for different species, such as A. niger (60 kDa; [Citation32]) and A. oryzae KSK-3 (30 kDa; [Citation25]).
3.6 Fermentation kinetics study
The data obtained from the above experiment was subjected to kinetics analysis for the calculations of μmax (h−1) (specific growth rate), qp (unit product produced/g spores/h), qs (g substrate consumed/g spores/h), Yp/s (unit product produced/g substrate consumed), Yp/x (unit product produced/g spores formed), Yx/s (g spores/g substrate utilized), Qp (g spores produced/l/h) and Qs (g substrate consumed/l/h). The kinetics evaluation also revealed that the optimum fermentation period for the extracellular production of protease from A. terreus was 96 h with a constant growth rate. Nonetheless, growth and enzyme secretion were significantly affected after the engineering of fermentation media (supplementation of nutrients) and optimization of different parameters. The specific production rate and growth coefficient revealed hyperproducibility of the extracellular protease (). Iftikhar et al. also reported similar observations for the production of protease from Rhizopus oligosporus var. microsporus [Citation40].
Table 5 Fermentation kinetics of produced protease.
4 Conclusion
This study revealed the possibilities of the effective utilization of agro-wastes in fermentation (LSSC and SSF) processes as potential substrates that act as carbon and nitrogen sources and ultimately produced industrially relevant enzymes. However, the production of protease will be efficient using CVP as one of the medium components through both LSSC and SSF in A. terreus. Obviously, such amalgamation of processes would not only facilitate the trimming of the entire production cost but also focus on the approaches towards the effective management of agro-wastes. This study portrays a gainful, expedient, non-tedious, relatively easy technique for scaling up the enhanced production of protease.
Acknowledgements
The authors would like to thank the University Grants Commission, New Delhi, India for financial assistance through Rajiv Gandhi National Fellowship Scheme with award letter Number F.14-2 (SC)/2008 (SA-III), Dated March 31, 2009. BKS would also like to thank Dr. Keshab C. Mondal (Head, Department of Microbiology, Vidyasagar University, West Midnapur, West Bengal, India) for assistance with necessary laboratory facilities.
Notes
Peer review under responsibility of Taibah University
References
- C.SandhyaA.SumanthaG.SzakacsA.PandeyComparative evaluation of neutral protease production by Aspergillus oryzae in submerged and solid state fermentationProc. Biochem.40200526892694
- T.GodfreyS.WestIndustrial Enzymology2nd ed.1996The Macmillan Press LtdLondon
- C.G.KumarH.TakagiMicrobial alkaline proteases: from a bioindustrial viewpointBiotechnol. Adv.171999561594
- M.B.RaoA.M.TanksaleM.S.GhatgeV.V.DeshpandeMolecular and biotechnological aspect of microbial proteasesMicrobiol. Mol. Biol. Rev.621998597635
- K.Q.BegR.GuptaPurification and characterization of an oxidation stable, thiol dependent serine alkaline protease from Bacillus mojavensisEnzyme Microb. Technol.322003294304
- H.S.JooC.S.ChangProduction of an oxidant and SDS alkaline protease from an alkalophilic Bacillus clausii I-52 by submerged fermentation: feasibility as a laundry detergent additiveEnzyme Microb. Technol.382006176183
- I.U.HaqH.MukhtariH.UmberProduction of protease by Penicillium chrysogenum through optimization of environmental conditionsJ. Agric. Soc. Sci.2120062325
- H.M.Fernandez-LahoreR.M.AudayE.R.FraileO.CasconeM.B.Jimenez BoninoL.de PirpignaniC.MachalinskiPurification and characterization of an acid proteinase from mesophilic Mucor sp. solid-state cultureJ. Pept. Res.531999599605
- C.R.TremacoldiN.K.WatanabeE.C.CarmonaProduction of extracellular acid proteases by Aspergillus clavatusWorld J. Microbiol. Biotechnol.202004639642
- S.KumarN.S.SharmaM.R.SaharanR.SinghExtracellular acid protease from Rhizopus oryzae: purification and characterizationProc. Biochem.40200517011705
- M.E.Acuna-ArguellesM.Gutierrez-RojasG.Viniegra-GonzálezE.Favela-TorresProduction and properties of three pectinolytic activities produced by Aspergillus niger in submerged and solid-state fermentationAppl. Microbiol. Biotechnol.431995808814
- B.K.SethiP.K.NandaS.L.SahooIsolation, identification and conservation of potent hydrolase producer from different soils of Odisha, IndiaInt. J. Pharm Bio Sci.42(B)201389100
- B.K.SethiJ.R.RoutR.DasP.K.NandaS.L.SahooLipase production by Aspergillus terreus using mustard seed oil cake as a carbon sourceAnn. Microbiol.632013241252
- A.JanaC.MaityS.K.HalderA.DasB.R.PatiK.C.MondalP.K.Das MohapatraStructural characterization of thermostable, solvent tolerant, cytosafe tannase from Bacillus subtilis PAB2Biochem. Eng. J.772013161170
- O.H.LowryN.J.RosebroughA.L.FarrR.J.RandallProtein measurements with the folin phenol reagentJ. Biol. Chem.1931951265275
- J.P.T.W.van den HomberghP.J.I.van de VondevoortN.C.B.A.van der HeijdenJ.VisserNew protease mutants in Aspergillus niger result in strongly reduced in vitro degradation of target proteins: genetic and biochemical characterization of seven complementation groupsCurr. Genet.19952829928308
- U.K.LaemmliCleavage of structural proteins during the assembly of the head of bacteriophage T4Nature2271970680685
- G.CoralB.ArikanM.N.UnaldiH.GuvenmezThermostable alkaline protease produced by an Aspergillus niger strainAnn. Microbiol.5342003491498
- D.M.GeiserR.A.SamsonJ.VargaA.RokasS.M.WitiakA review of molecular phylogenetics in Aspergillus and prospects for a robust genus-wide phylogenyJ.VargaR.A.SamsonAspergillus in the Genomic Era2008Wageningen Academic PublishersNetherlands1732
- M.K.DeviA.R.BanuG.R.GnanaprabhalB.V.PradeepM.PalaniswamyPurification, characterization of alkaline protease enzyme from native isolate Aspergillus niger and its compatibility with commercial detergentInd. J. Sci. Technol.172008410
- M.HajjiA.RebaiN.GharsallahM.NasriOptimization of alkaline protease production by Aspergillus clavatus ES1 in Mirabilis jalapa tuber powder using statistical experimental designAppl. Microbiol. Biotechnol.792008915923
- G.SrinubabuN.LokeswariK.JayarajuScreening of nutritional parameters for the production of proteases from Aspergillus oryzaeElectr. J. Chem.422007208215
- S.NegiR.BanerjeeOptimization of culture parameters to enhance production of amylase and protease from Aspergillus awamori in a single fermentationAfr. J. Biochem. Res.4320107380
- K.SaravanakumarA.ThiyagarajanV.KaviyarasanOptimization of medium constituents for the production of extracellular alkaline Protease by Aspergillus fischeri using response surface experimental designJ. Biosci. Res.132010118129
- N.ShirasakaM.NaitouK.OkamuraM.KusudaY.FukutaT.TerashitaPurification and characterization of a fibrinolytic protease from Aspergillus oryzae KSK-3Mycoscience532012354364
- K.AikatB.C.BhattacharyaProtease extraction in solid state fermentation of wheat bran by a local strain of Rhizopus oryzae and growth studies by the soft gel techniqueProc. Biochem.352000907914
- S.K.ChakrabartiN.MatsumuraR.S.RanuPurification and characterization of an extracellular alkaline serine protease from Aspergillus terreus (IJIRA 6.2)Curr. Microbiol.402000239244
- P.CharlesV.DevanathanP.AnbuM.N.PonnuswamyP.T.KalaichelvanB.K.HurPurification, characterization and crystallization of an extracellular alkaline protease from Aspergillus nidulans HA-10J. Basic Microbiol.4852008347351
- M.D.H.C.MacielP.N.HerculanoT.S.PortoM.F.S.TeixeiraK.A.MoreiraC.M.de Souza-MottaProduction and partial characterization of proteases from forage palm by Aspergillus niger URM4645Afr. J. Biotechnol.1013201124692475
- M.K.ChimataP.SasidharS.ChallaProduction of extracellular amylase from agricultural residues by a newly isolated Aspergillus species in solid state fermentationAfr. J. Biotechnol.932201051625169
- R.ParanthamanK.AlagusundaramJ.IndhumathiProduction of protease from rice mill wastes by Aspergillus niger in solid state fermentationWorld J. Agric. Sci.52009308312
- J.F.BenazirR.SuganthiA.HariV.Ramesh KumarM.P.AswathiG.NiraimathiM.K.MalaS.SukanyaR.SanthiBio-utilization of agroindustrial waste in solid state fermentation by Aspergillus niger for the production of proteaseAsiat. J. Biotechnol. Resour.242011422435
- N.AkhterM.A.MorshedA.UddinF.BegumT.SultanA.K.AzadProduction of protease by Aspergillus niger cultured in solid state mediaInt. J. Biosci.1120113342
- A.SumanthaC.SandhyaG.SzakacsC.R.SoccolA.PandeyProduction and partial purification of a neutral metalloprotease by fungal mixed substrate fermentationFood Technol. Biotechnol.4342005313319
- J.LaiZ.LiY.LuZ.YangSpecific ion effects of ionic liquids on enzyme activity and stabilityGreen Chem.13201118601868
- A.F.AfifiE.M.KamelA.A.KhalilM.A.FoaadE.M.FawziM.M.HousenyPurification and characterization of α-amylase from Penicillium olsonii under the effect of some antioxidant vitaminsGlob. J. Biotechnol. Biochem.3120081421
- J.ChutmanopS.ChuichulchermY.ChistiP.SrinophakunProtease production by Aspergillus oryzae in solid state fermentation using agroindustrial substratesJ. Chem. Technol. Biotechnol.83200810121018
- T.Y.WuA.W.MohammadJ.M.JahimN.AnuarInvestigations on protease roduction by a wild-type Aspergillus terreus strain using diluted retentate of pre-filtered palm oil mill effluent (POME) as substrateEnzyme Microbial Technol.39200612231229
- R.SindhuG.N.SuprabhaS.ShashidharOptimization of process parameters for the production of alkaline protease from Penicillium godlewskii SBSS 25 and its application in detergent industryAfr. J. Microbiol. Res.392009515522
- T.IftikharM.NiazM.A.ZiaI.U.HaqProduction of extracellular lipases by Rhizopus oligosporus in a stirred fermentorBraz. J. Microbiol.41201011241132