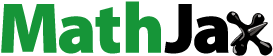
Abstract
The adsorption and corrosion inhibition behavior of (E)-2-methyl-N-(thiophen-2-ylmethylidene)aniline (T) at steel/1.0 M HCl interface was investigated using gravimetric and electrochemical methods at 308 K. Inhibition efficiency increased with increase in the concentration of new synthesized Tiophene Schiff base. The potentiodynamic polarization studies revealed that this compound as mixed-type inhibitor. Data, obtained from EIS measurements, were analyzed to model the corrosion inhibition process through appropriate equivalent circuit model; a constant phase element (CPE) has been used. The inhibition was assumed to occur via adsorption of the components of the Thiophene derivative on the mild steel surface which can be approximated by Langmuir adsorption isotherm model. Quantum chemical parameters are calculated using the density functional theory (DFT) method. Correlation between theoretical and experimental results is discussed.
1 Introduction
Iron and its alloys play crucial roles in our daily lives due to their excellent properties, such as high structural and mechanical strengths [Citation1,Citation2]. These materials are used in various industrial and engineering applications. Acid-pickling, acid-cleaning, acid descaling and oil-well acidizing are well-known industrial processes for cleaning the surfaces of metals. Acid solutions are used for these applications. It is necessary to use acid solutions to remove undesirable scale and corrosion products from metals. Hydrochloric acid and sulphuric acid are commonly used for this purpose; however, these acids attack the metal and initiate corrosion. This corrosion can cause serious damage to the metal and degrade its properties, thereby limiting its applications [Citation3–Citation8]. To prevent from acidic solutions aggression, the use of organic inhibitors is one of the most practical methods and cost-effective choices for protect metals against corrosion. These organic inhibitors are usually adsorbed on the metal surface via formation of a coordinate covalent bond (chemical adsorption) or the electrostatic interaction between the metal and inhibitor (physical adsorption) [Citation9]. This adsorption produces a uniform film, which isolates the metal surface from the aggressive medium and consequently reduces the corrosion extent [Citation10]. In general, the adsorption depends on (i) the nature and the state of the metal surface, (ii) the type of corrosive environment and (iii) the chemical structure of inhibitor [Citation11,Citation12]. Organic compounds containing heteroatoms with electronic lone pair (N, O, S and P), or p systems, or conjugated bonds, or aromatic rings, are generally considered to be effective corrosion inhibitors [Citation13–Citation21]. As important S-heterocyclic compounds, Schiff's bases are also used as efficient inhibitors for mild steel corrosion in HCl media due to the presence of –C=N– group [Citation22,Citation23]. The lone pair of electrons on N and S atoms, and the planarity of the molecule are useful for its adsorption to the metal surface [Citation24]. As they are non-toxic and biodegradable; the examination of their anticorrosive proprieties is significant in the context of the current priority to synthesize inhibitors with low environmental impact.
As it is known, some authors have explained that not only the p electron of the Schiff bases enter unoccupied orbitals of transition metals, but the π* orbital can also accept the electrons of transition metal d-orbitals to form feedback bonds [Citation25] and thereby enabling existence in metal-inhibitor bond. In addition to this, Schiff bases have been recently reported as effective corrosion inhibitors for steel, aluminum and copper in acidic media. The greatest advantages of Schiff bases are [Citation26,Citation27]:
(a) | they can be synthesized conveniently from inexpensive raw materials |
(b) | they contain the electron cloud on the aromatic ring or, the electronegative atoms such as nitrogen and sulfur in the relatively long chain compounds |
(c) | harmless for environment, which encouraged us to test this type of inhibitor. |
In the present study, density functional theory (DFT) was used to determine the molecular structure of (E)-2-methyl-N-(thiophen-2-ylmethylidene)aniline (T) as a corrosion inhibitor for mild steel. The corrosion inhibition behavior of new Thiophene derivative on the mild steel in acidic solution was investigated using some experimental techniques.
2 Materials and methods
2.1 Materials
All commercial reagents were analytical grade and used without further purification. Melting points were determined by using a BUCHÏ 510 m.p. apparatus. The NMR spectra were obtained with a Bruker AC 300 spectrometer (CNRS). Molecular weights were determined on a JEOL JMS DX-300 Mass Spectrometer.
2.1.1 Synthesis of (E)-2-methyl-N-(thiophen-2-ylmethylidene)aniline (T)
This product was prepared by mixing ortho-toluidine (2.23 g, 20 mmol) and furan-2-carbaldehyde (2 g, 20 mmol) in 50 ml of dray diethyl ether. The reaction mixture was stirred for 7 days at room temperature using acetic acid as catalyst. The reaction completion was confirmed by TLC and the product was purified on silica gel column flash-chromatography using CH2Cl2/MeOH. The resulting product was precipitated in petrol ether, filtrated and dried to get pure product as brown powder. Yield 57% (2.1 g, 11.33 mmol). M.p. 62 °C. Rf = 0.77 (silica, CH2Cl2/MeOH, 9/1). 1H NMR (300 MHz, CDCl3) δ ppm: 8.17 (s, 1H, CH=N); 7.61 (d, 1H, furan-Hα); 7.23 (d, 1H, phenyl, C3H); 7.19 (t, 1H, phenyl, C5H); 7.14 (t, 1H, phenyl, C4H); 7.00 (d, 1H, phenyl, C6H); 6.91 (t, 1H, furan-Hγ); 8.74 (m, 1H, furan-Hβ); 2.51 (s, 3H, CH3). 13C NMR (75 MHz, CDCl3) δ ppm: 148.07 (1C, C=N); 145.67 (1C, furan-Cα); 131.99 (1C, phenyl-C5-CH3); 130.39 (1C, phenyl-C3); 126.75 (1C, phenyl-C4); 125.92 (1C, phenyl-C5); 117.85 (1C, furan-Cγ); 116.02 (1C, phenyl-C6); 112.20 (1C, furan-Cβ); 17.95 (1C, CH3). m/z (M+): 185.91. IR: ν(CH=N, imine) = 1630 cm−1. The synthetic route of inhibitor (T) is shown in and the structure of inhibitor is shown in .
The steel used in this study is a mild steel with a chemical composition (in wt%) of 0.09% P, 0.01% Al, 0.38% Si, 0.05% Mn, 0.21% C, 0.05% S and the remainder iron (Fe).
The aggressive solutions of 1.0 M HCl were prepared by dilution of analytical grade 37% HCl with distilled water. The concentration range of (E)-2-methyl-N-(thiophen-2-ylmethylidene)aniline (T) used was 5 × 10−5 M to 1 × 10−3 M.
2.2 Weight loss measurements
Gravimetric experiments were performed according to the standard methods [Citation28], the mild steel specimens of dimension 1.5 cm × 1.5 cm × 0.05 cm, were abraded successively with different grades of emery papers, washed with distilled water, cleaned with acetone. After being weighed accurately with high sensitivity balance, the specimens were immersed in 80 ml of 1.0 M HCl with and without various concentrations of the studied Thiophene derivative (T) at 308 K in aerated condition. After a 6 h immersion, the specimens were taken out, rinsed thoroughly with distilled water, dried and weighed accurately again. The corrosion rate (ν) and the inhibition efficiency (ηWL) were calculated by the following equations [Citation29]:(1)
(1)
(2)
(2) where W is the three-experiment average weight loss of the mild steel, S is the total surface area of the specimen, t is the immersion time and ν0 and ν are values of the corrosion rate without and with addition of the inhibitor, respectively.
2.3 Electrochemical measurements
The electrochemical measurements were carried out using Volta lab (Tacussel-Radiometer PGZ 100) potentiostate and controlled by Tacussel corrosion analysis software model (Voltamaster 4) at under static condition. The corrosion cell used had three electrodes. The reference electrode was a saturated calomel electrode (SCE). A platinum electrode was used as auxiliary electrode of surface area of 1 cm2. The working electrode was mild steel. All potentials given in this study were referred to this reference electrode. The working electrode was immersed in test solution for 30 min to a establish steady state open circuit potential (Eocp). After measuring the Eocp, the electrochemical measurements were performed. All electrochemical tests have been performed in aerated solutions at 308 K. The EIS experiments were conducted in the frequency range of 100 kHz to 10 mHz at open circuit potential, with 10 points per decade, at the rest potential, after 30 min of acid immersion, by applying 10 mV ac voltage peak-to-peak. Nyquist plots were made from these experiments. The impedance data were analyzed and fitted with the simulation ZView 2.80, equivalent circuit software.
After ac impedance test, the potentiodynamic polarization measurements of mild steel substrate in inhibited and uninhibited solution were scanned from cathodic to the anodic direction, E = Ecorr ± 300 mV, with a scan rate of 1 mV s−1. The potentiodynamic data were analyzed using the polarization VoltaMaster 4 software. The linear Tafel segments of anodic and cathodic curves were extrapolated to corrosion potential to obtain corrosion current densities (Icorr). From the polarization curves obtained, the corrosion current (Icorr) was calculated by curve fitting using the equation:(3)
(3) βa and βc are the anodic and cathodic Tafel slopes and ΔE is E − Ecorr.
The inhibition efficiency was evaluated from the measured Icorr values using the following relationship:(4)
(4) where Icorr and Icorr(i) are the corrosion current densities for steel electrode in the uninhibited and inhibited solutions, respectively.
2.4 Quantum chemical calculations
Density functional theory (DFT) has been recently used [Citation30–Citation33], to describe the interaction between the inhibitor molecule and the surface as well as the properties of these inhibitors concerning their reactivity. The molecular band gap was computed as the first vertical electronic excitation energy from the ground state using the time-dependent density functional theory (TD-DFT) approach as implemented in Gaussian 03 [Citation34]. For these seek, some molecular descriptors, such as HOMO and LUMO energy values, frontier orbital energy gap, molecular dipole moment, electronegativity (χ), global hardness (η), softness (σ), were calculated using the DFT method and have been used to understand the properties and activity of the newly prepared compounds and to help in the explanation of the experimental data obtained for the corrosion process.
According to Koopman's theorem [Citation35], the ionization potential (IE) and electron affinity (EA) of the inhibitors are calculated using the following equations.(5)
(5)
(6)
(6) Thus, the values of the electronegativity (χ) and the chemical hardness (η) according to Pearson, operational and approximate definitions can be evaluated using the following relations [Citation36]:
(7)
(7)
(8)
(8) Global chemical softness (σ), which describes the capacity of an atom or group of atoms to receive electrons [Citation31], was estimated by using the equation:
(9)
(9)
3 Results and discussion
3.1 Weight loss measurements
The inhibition efficiency values for mild steel in 1.0 M HCl media at different concentrations of the inhibitor are presented in . It is apparent that the inhibition efficiency increased with the increase in inhibitor concentration in 1.0 M HCl media. This behavior can be explained based on strong interaction of the inhibitor molecule with the metal surface resulting in adsorption [Citation37]. The extent of adsorption increases with the increase in concentration of the inhibitor leading to increased inhibition efficiency. In the acid solutions, the maximum inhibition efficiency was observed at an inhibitor concentration of 10−3 M. Generally, organic inhibitors suppress the metal dissolution by forming a protective film adsorbed to the metal surface and separating it from the corrosion medium [Citation38–Citation42]. The corrosion suppressing ability of the inhibitor molecule originates from the tendency to form either strong or weak chemical bonds with Fe atoms using the lone pair of electrons present on the S and N atoms and the π electrons present in >C=N– moiety and in benzene ring.
Table 1 Weight loss data for mild steel 1.0 M HCl without and with different concentrations of Thiophene derivative at 308 K.
3.2 Adsorption isotherm and thermodynamic parameters
It is well recognized that the first step in inhibition of metallic corrosion is the adsorption of organic inhibitor molecules at the metal/solution interface and that the adsorption depends on the molecule's chemical composition, the temperature and the electrochemical potential at the metal/solution interface. In fact, the solvent H2O molecules could also adsorb at metal/solution interface. So the adsorption of organic inhibitor molecules from the aqueous solution can be regarded as a quasi-substitution process between the organic compounds in the aqueous phase [Org(sol)] and water molecules at the electrode surface [H2O(ads)] as represented in Eq. Equation(10) [Citation43]:
Org(sol) and Org(ads) are the organic molecules in the aqueous solution that adsorbed to the metal surface. While H2O(ads) is the water molecule on the metal surface in which n is the coefficient that represent water molecules replaced by a unit of Thiophene derivative.
The adsorption behavior of the inhibitor molecules on metal surface explains their inhibition mechanism [Citation44,Citation45]. Several adsorption isotherms can be used to assess the adsorption behavior of the inhibitors. Langmuir adsorption isotherm is the best description of the adsorption behavior of the inhibitor molecules on the mild steel surface, which obeys Eq. Equation(12)(12)
(12) as follows [Citation46,Citation47]. The values of surface coverage (θ) for different concentrations at 308 K have been used to explain the best isotherm that determines the adsorption process. The surface coverage values (θ) were evaluated using corrosion rate values obtained from the weight loss method. Coverage can be obtained from weight loss measurement by the following equation:
(11)
(11)
Attempts were made to fit θ values to various isotherms including Langmuir, Frumkin, Freundlich and Temkin isotherms as follows [Citation48–Citation51]:(12)
(12)
(13)
(13)
(14)
(14) θ is the surface coverage, K is the adsorption–desorption equilibrium constant, C is the concentration of inhibitor and f is the factor of energetic inhomogeneity. Again, the weight loss measurements were employed in this experiment with the concentration range 10−6–10−3 M at 308 K. The corresponding plots are shown in , where the R2 value for Langmuir isotherm (A) was 0.99952, Frumkin isotherm (B) was 0.95934 and Temkin isotherm (C) was 0.98542. From this observation, it is concluded that Langmuir isotherm shows the best correlation with the experimental data. The kinetic thermodynamic model isotherm [Citation52] is given by:
(15)
(15) where K′ is a constant, and y is the number of inhibitor molecules occupying one active site. A plot of Ln(θ/1 − θ) versus Ln C gives a straight line of slop y and intercept of Ln K′, as shown in . Equilibrium constant corresponding to adsorption isotherm is given by, K = K′1/y. Value of y > 1 implies the formation of multilayer of inhibitor on the surface of metal. Value of y < 1 mean a given inhibitor molecules will occupy more than one active site. The behavior of equilibrium constants obtained from Langmuir model was similar to the values which obtained by kinetic–thermodynamic model. The free energy of adsorption
, also can be calculated using the following equation:
(16)
(16) where 55.5 is the molar concentration of water, R is the universal gas constant and T is the temperature in K. The calculated value of free energy of adsorption was found to be
, where adsorption–desorption equilibrium constant K value was obtained from the linear regression of Langmuir isotherm (66794.47 L mol−1).
Fig. 2 (A) Langmuir, (B) Frumkin and (C) Temkin isotherm for the adsorption of Thiophene derivative on the surface of mild steel in 1.0 M HCl.
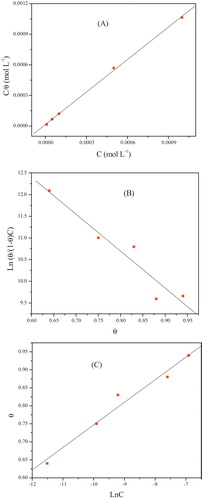
The negative value of indicates that the inhibitor, in this case Thiophene derivative is spontaneously adsorbed onto the mild steel surface. It is well known that values of
around −20 kJ mol−1 or lower are associated with the physisorption phenomenon where the electrostatic interaction assemble between the charged molecule and the charged metal, while those around −40 kJ mol−1 or higher are associated with the chemisorption phenomenon where the sharing or transfer of organic molecules charge with the metal surface occurs [Citation53,Citation54]. The calculated value of
of Thiophene derivative in HCl indicates that the adsorption mechanism of this compound on mild steel involves both chemisorption and physisorption [Citation55–Citation57]. Owing to the adsorbed water molecules on the surface of mild steel, it may be assumed that the adsorption occurs first due to the electrostatic interaction, and then the removal of water molecules from the surface is accompanied by chemical interaction between the metal surface and the adsorbate [Citation58].
3.3 Polarization measurements
shows the polarization curves for mild steel in 1.0 M HCl in the absence and presence of (E)-2-methyl-N-(thiophen-2-ylmethylidene)aniline (T). It can be noticed that the addition of Thiophene derivative causes a remarkable decrease in the corrosion rate, shifting both anodic and cathodic Tafel curves to lower current densities. This phenomenon indicates that both anodic and cathodic reactions are suppressed and the suppression effect becomes more pronounced with the increase of the concentration of this inhibitor. The electrochemical parameters such as corrosion potential (Ecorr), cathodic Tafel slope (βc), and corrosion current density (Icorr) obtained by extrapolating the Tafel line are given in . The corrosion inhibition efficiency (ηTafel) of this compound is calculated using the relationship Equation(4)(4)
(4) .
Fig. 4 Tafel polarization curves for mild steel obtained at 308 K in 1.0 M HCl solution containing different concentrations of Thiophene derivative.
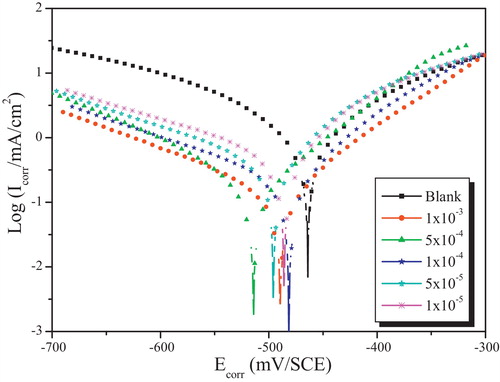
Table 2 Potentiodynamic electrochemical parameters for the corrosion of mild steel in 1.0 M HCl solution in the absence and presence of the investigated inhibitor at 308 K.
We can classify an inhibitor as cathodic or anodic type if the displacement in corrosion potential is more than 85 mV with respect to corrosion potential of the blank [Citation59]. In the presence of this Thiophene derivative, the corrosion potential of mild steel shifted to the negative side only 49 mV (vs. SCE). This can be interpreted that inhibitor acts as a mixed type inhibitor and shows more pronounced influence in the cathodic polarization plots compared to that in the anodic plots. The parallel cathodic current–potential curves suggest that the addition of this inhibitor does not modify the hydrogen evolution mechanism and the hydrogen evolution is activation-controlled.
This suppression of the corrosion process may be attributed to the covering of adsorbed (E)-2-methyl-N-(thiophen-2-ylmethylidene)aniline (T) molecules on the mild steel surface [Citation60]. For anodic polarization curves of mild steel with this compound, it is apparent that the presence of the Thiophene derivative does not modify the current vs. potential characteristics obviously. The data in reveals that when the concentration of this inhibitor increased, the inhibition efficiency increases and the corrosion current density decreases sharply. This may be due to the adsorption layer of the inhibitor on the metal surface.
3.4 Electrochemical impedance spectroscopy
The corrosion of mild steel in 1.0 M HCl solution in the presence of Thiophene derivative was investigated by EIS at room temperature after an exposure period of 30 min. Nyquist plots for mild steel obtained at the interface in the absence and presence of this inhibitor at different concentrations is given in .
A single semicircle has been observed at high frequency which can be attributed to charge transfer of the corrosion process and the diameter of the semicircle increased with increasing inhibitor concentration. clearly shows that the impedance spectra are not a perfect semicircle. They seem to be depressed with center under real axis and resemble depressed capacitive loops. Such phenomenon often corresponds to surface heterogeneity which may be the result of surface roughness, dislocations, distribution of the active sites, or adsorption of the inhibitor molecules [Citation61–Citation63]. An equivalent circuit was introduced to explain the EIS data as shown in .
This circuit is generally used to describe the iron/acid interface model [Citation64]. In this circuit Rs is solution resistance, Rct is charge transfer resistance, and CPE is a constant phase element. The impedance function of the CPE is as follows:(17)
(17) where Y is the magnitude of the CPE, ω is the angular frequency, and the deviation parameter n is a valuable criterion of the nature of the metal surface and reflects microscopic fluctuations of the surface. For n = 0, ZCPE represents a resistance with R = Y1−; n = −1 an inductance with L = Y−1 and n = 1 an ideal capacitor with C = Y [Citation65]. In iron/acid interface systems, ideal capacitor behavior is not observed due to the roughness or uneven current distributions on the electrode surface which results in frequency dispersion [Citation66–Citation68].
lists impedance parameters of the Nyquist plots of the Thiophene derivative in different concentrations.
Table 3 Impedance parameters and inhibition efficiency for mild steel in 1.0 M HCl solutions containing different concentrations of Thiophene derivative.
The inhibition efficiency of the inhibitor was calculated from the charge transfer resistance values using the following equation:(18)
(18) where
and
are the charge transfer resistance in absence and in presence of inhibitor, respectively. The EIS measurement reveals that at the concentration of 10−3 M, the percentage of inhibition efficiency is highest (90% ηz). The result strongly supports the observation that 10−3 M of this compound could work best as an inhibitor.
The results also show that Rct values increased with increase in additive concentration except few cases. The percentage inhibition efficiencies calculated from the Rct values indicate that Thiophene derivative acts as a good corrosion inhibitor of mild steel in HCl medium. The CPE values found to decrease with increase in concentration of inhibitor solutions. This behavior is generally seen for system where inhibition occurred due to the formation of a surface film by the adsorption of inhibitor on the metal surface [Citation69,Citation70]. Decrease in CPE, which can result from a decrease in local dielectric constant and/or an increase in the thickness of the electrical double layer, suggest that the inhibitor molecules act by adsorption at the metal/solution interface [Citation71]. The values of n obtained for this inhibitor system were close to unity which shows that the interface behaves nearly capacitive [Citation72].
3.5 Quantum chemical calculations
Quantum chemical calculation has been widely used to evaluate the inhibition performance of corrosion inhibitors, which can quantitatively study the relationship between inhibition efficiency and molecular reactivity [Citation73–Citation76]. With this method, the capability of inhibitor molecules to donate or accept electrons can be predicted with analysis of global reactivity parameters, such as the energy gap between HOMO and LUMO, chemical hardness, and dipole moment.
The reactive abilities of Thiophene derivative are closely related to their frontier molecular orbitals including the highest occupied molecular orbital (HOMO) and the lowest unoccupied molecular orbital (LUMO). EHOMO indicates the tendency of an organic molecule to donate electrons. The higher the value of EHOMO, the greater the ability of a molecule to donate electrons while ELUMO indicates the propensity of a molecule to accept electrons. The lower ELUMO is, the greater is the ability of that molecule to accept electrons. Thus, the binding ability of organics to the metal surface increases with an increase in energy of the HOMO and a decrease in the value of energy of the LUMO. The energy gap, ΔE, is an important parameter which indicates the reactivity tendency of organics toward the metal surface [Citation77]. As ΔE decreases, the reactivity of the molecule increases, leading to an increase in adsorption onto a metal surface. A molecule with low energy gap is more polarizable and is generally associated with high chemical reactivity and low kinetic stability. Thus, ΔE has been used in literature to characterize the binding ability of organics to the metal surface [Citation78]. The reactivity of corrosion inhibitors may also be discussed in terms of chemical hardness and softness parameters. These quantities are often associated with the Lewis theory of acid and bases and Pearson's hard and soft acids and bases theory [Citation79]; a hard molecule has a large ΔE and therefore is less reactive; a soft molecule has a small ΔE and is therefore more reactive. Adsorption occurs most probably at the region of the molecule where softness (σ) has the highest value [Citation80]. and show the optimized geometry, the HOMO density distribution and the LUMO density distribution.
Fig. 8 The frontier molecule orbital density distributions of Thiophene derivative: HOMO (left) and LUMO (right).
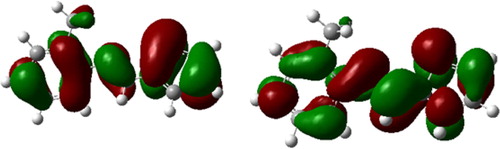
shows the calculated quantum chemical properties for Thiophene derivative.
Table 4 Molecular properties of Thiophene derivative obtained from the optimized structure using DFT at the B3LYP/6-31G*.
It is evident from , the highest value of EHOMO = −0.324 eV of the studied compound indicates the better inhibition efficiency. On the other hand, it has also been found that an inhibitor does not only donate an electron to the unoccupied d orbital of the metal ion but can also accept electrons from the d orbital of the metal leading to the formation of a feedback bond. Therefore, the tendency for the formation of a feedback bond would depend on the value of ELUMO. The lower value of the ELUMO = −0.209 eV indicates the easier of the acceptance of electrons from the d orbital of the metal [Citation81,Citation82]. The band gap energy, ΔE = ELUMO − EHOMO is an important parameter as a function of reactivity of the inhibitor molecule toward the adsorption on metallic surface. As ΔE decreases, the reactivity of the molecule increases leading to increase the inhibition efficiency of the molecule. The calculations indicate that our studied molecule has a small value of gap energy (0.115 eV) which means the highest reactivity and accordingly the highest inhibition efficiency which agrees well with the experimental observations.
Absolute hardness and softness are important properties to measure the molecular stability and reactivity. A hard molecule has a large energy gap and a soft molecule has a small energy gap. Soft molecules are more reactive than hard ones because they could easily offer electrons to an acceptor. For the simplest transfer of electrons, adsorption could occur at the part of the molecule where σ, which is a local property, has the highest value [Citation83]. In a corrosion system, the inhibitor acts as a Lewis base while the metal acts as a Lewis acid. Bulk metals are soft acids and thus soft base inhibitors are most effective for acidic corrosion of those metals. In our present work the studied molecule has low hardness value 0.0575 eV and a highest value of softness of 17.3913.
The most widely used quantity to describe the polarity is the dipole moment of the molecule [Citation84]. Dipole moment is the measure of polarity of a polar covalent bond. It is defined as the product of charge on the atoms and the distance between the two bonded atoms. The total dipole moment, however, reflects only the global polarity of a molecule. For a complete molecule the total molecular dipole moment may be approximated as the vector sum of individual bond dipole moments. The dipole moment (μ in Debye) is another important electronic parameter that results from non-uniform distribution of charges on the various atoms in the molecule. The high value of dipole moment probably increases the adsorption between chemical compound and metal surface [Citation85]. In our study, the value of the dipole moment is 0.818 Debye.
3.6 Inhibition mechanism
From the results obtained from different electrochemical and weight loss measurements, it was concluded that Thiophene derivative inhibits the corrosion of mild steel in 1.0 M HCl through its adsorption at the metal/solution interface. The Thiophene derivative can be easily protonated because its molecules are made of planer aromatic rings of benzene and the thiophene ring, and also contain S and N atoms and π electrons. Generally, in acid solutions, the inhibition of metallic corrosion occurs through (1) electrostatic interaction of protonated molecules with already adsorbed chloride ions, (2) donor–acceptor interactions between the p electrons of the aromatic ring and the vacant d-orbital of the iron atoms, and (3) interaction between unshared electron pairs of hetero atoms and the vacant d-orbital of the iron surface atoms [Citation47]. In the present study, the calculated value, indicating that the adsorption of esomeprazole on the mild steel surface follows both physisorption (ionic) and chemisorption (molecular) mechanisms.
4 Conclusion
(E)-2-methyl-N-(thiophen-2-ylmethylidene)aniline shows good inhibitive properties for mild steel corrosion in 1.0 M HCl solution and the inhibition efficiency increases with increasing the concentration of the inhibitor. The results of potentiodynamic polarization measurements demonstrate Thiophene derivative behaves as a mixed type inhibitor and could suppress both anodic metal dissolution and cathodic hydrogen evolution reactions. (E)-2-methyl-N-(thiophen-2-ylmethylidene)aniline on the metal surface obeyed Langmuir adsorption isotherm. The value of the adsorption equilibrium constant shows that inhibitor is strongly adsorbed on the metal surface. The quantum mechanical approach may well be able to foretell molecule structures that are better for corrosion inhibition.
Conflict of interest
The authors declare no competing financial interest.
Notes
Peer review under responsibility of Taibah University.
References
- D.M.StricklandThe resistivity of iron and its application to the chemical industryInd. Eng. Chem.151923566569
- J.D.HatfieldA.V.SlackG.L.CrowH.B.ShafferCorrosion in fertilizer equipment, corrosion of metals by liquid mixed fertilizersJ. Agric. Food Chem.61958524531
- I.A.AdejoroF.K.OjoS.K.ObafemiCorrosion inhibition potentials of ampicillin for mild steel in hydrochloric acid solutionJ. Taibah Univ. Sci.201510.1016/j.jtusci.2014.10.002
- D.Ben HmamouR.SalghiA.ZarroukM.R.AouadO.BenaliH.ZarrokM.MessaliB.HammoutiM.M.KabandaM.BouachrineE.E.EbensoWeight loss, electrochemical, quantum chemical calculation, and molecular dynamics simulation studies on 2-(benzylthio)-1,4,5-triphenyl-1H-imidazole as an inhibitor for mild steel corrosion in hydrochloric acidInd. Eng. Chem. Res.5220131431514327
- H.ZarrokR.SaddikH.OuddaB.HammoutiA.El MidaouiA.ZarroukN.BenchatM.Ebn Touhami5-(2-Chlorobenzyl)-2,6-dimethylpyridazin-3-one: an efficient inhibitor of C38 steel corrosion in hydrochloric acidDer Pharm. Chem.42011272282
- A.GhazouiR.SaddikN.BenchatB.HammoutiM.GuenbourA.ZarroukM.RamdaniThe role of 3-amino-2-phenylimidazo[1,2-a]pyridine as corrosion inhibitor for C38 steel in 1 M HClDer Pharm. Chem.42012352364
- A.H.Al HamziH.ZarrokA.ZarroukR.SalghiB.HammoutiS.S.Al-DeyabM.BouachrineA.AmineF.GuenounThe role of acridin-9(10H)-one in the inhibition of carbon steel corrosion: thermodynamic, electrochemical and DFT studiesInt. J. Electrochem. Sci.8201325862605
- C.M.GoulartA.Esteves-SouzaC.A.Martinez-HuitleC.J.F.RodriguesM.A.M.MacielA.EchevarriaExperimental and theoretical evaluation of semicarbazones and thiosemicarbazones as organic corrosion inhibitorsCorros. Sci.672013281291
- G.AvciInhibitor effect of N,N′-methylenediacrylamide on corrosion behavior of mild steel in 0.5 M HClMater. Chem. Phys.1122008234238
- E.S.MereshtT.S.FarahaniJ.Neshati2-Butyne-1,4-diol as a novel corrosion inhibitor for API X65 steel pipeline in carbonate/bicarbonate solutionCorros. Sci.5420123644
- M.GopiramanN.SelvakumaranD.KesavanR.KarvembuAdsorption and corrosion inhibition behaviour of N-(phenylcarbamothioyl)benzamide on mild steel in acidic mediumProg. Org. Coat.732012104111
- A.ZarroukB.HammoutiH.ZarrokR.SalghiA.DafaliLh.BazziL.BammouS.S.Al-DeyabElectrochemical impedance spectroscopy and weight loss study for new pyridazine derivative as inhibitor for copper in nitric acidDer Pharm. Chem.42012337346
- A.ZarroukB.HammoutiH.ZarrokS.S.Al-DeyabM.MessaliTemperature effect, activation energies and thermodynamic adsorption studies of l-cysteine methyl ester hydrochloride as copper corrosion inhibitor in nitric acid 2MInt. J. Electrochem. Sci.6201162616274
- A.ZarroukB.HammoutiA.DafaliH.Zarrokl-Cysteine methyl ester hydrochloride: a new corrosion inhibitor for copper in nitric acidDer Pharm. Chem.32011266274
- A.ZarroukB.HammoutiH.ZarrokI.WaradM.BouachrineN-containing organic compound as an effective corrosion inhibitor for copper in 2 M HNO3: weight loss and quantum chemical studyDer Pharm. Chem.32011263271
- A.GhazouiN.BencahtS.S.Al-DeyabA.ZarroukB.HammoutiM.RamdaniM.GuenbourAn investigation of two novel pyridazine derivatives as corrosion inhibitor for C38 steel in 1.0 M HClInt. J. Electrochem. Sci.8201322722292
- A.ZarroukH.ZarrokR.SalghiN.BouroumaneB.HammoutiS.S.Al-DeyabR.TouzaniThe adsorption and corrosion inhibition of 2-[bis-(3,5-dimethyl-pyrazol-1-ylmethyl)-amino]-pentanedioic acid on carbon steel corrosion in 1.0 M HClInt. J. Electrochem. Sci.7 20121021510232
- H.BendahaA.ZarroukA.AounitiB.HammoutiS.El KadiriR.SalghiR.TouzaniAdsorption and corrosion inhibitive properties of some tripodal pyrazolic compounds on mild steel in hydrochloric acid systemsPhys. Chem. News64201295103
- H.ZarrokA.ZarroukR.SalghiY.RamliB.HammoutiM.AssouagE.M.EssassiH.OuddaM.Taleb3,7-Dimethylquinoxalin-2-(1H)-one for inhibition of acid corrosion of carbon steelJ. Chem. Pharm. Res.4201250485055
- H.ZarrokK.Al MamariA.ZarroukR.SalghiB.HammoutiS.S.Al-DeyabE.M.EssassiF.BentissH.OuddaGravimetric and electrochemical evaluation of 1-allyl-1h-indole-2,3-dione of carbon steel corrosion in hydrochloric acidInt. J. Electrochem. Sci.720121033810357
- D.DaoudT.DouadiS.IssaadiS.ChafaaAdsorption and corrosion inhibition of new synthesized thiophene Schiff base on mild steel X52 in HCl and H2SO4 solutionsCorros. Sci.7920145058
- S.IssaadiT.DouadiA.ZouaouiS.ChafaaM.A.KhanG.BouetNovel thiophene symmetrical Schiff base compounds as corrosion inhibitor for mild steel in acidic mediaCorros. Sci.53201114841488
- R.SolmazE.AltunbasG.KardasAdsorption and corrosion inhibition effect of 2-((5-mercapto-1,3,4-thiadiazol-2-ylimino)methyl)phenol Schiff base on mild steelMater. Chem. Phys.1252011796801
- S.DengX.LiX.XieHydroxymethyl urea and 1,3-bis(hydroxymethyl) urea as corrosion inhibitors for steel in HCl solutionCorros. Sci.802014276289
- R.HasanovM.SadıkoğluS.BilgiçElectrochemical and quantum chemical studies of some Schiff bases on the corrosion of steel in H2SO4 solutionAppl. Surf. Sci.253200739133921
- R.A.PrabhuT.V.VenkateshaA.V.ShanbhagG.M.KulkarniR.G.KalkhambkarInhibition effects of some Schiff's bases on the corrosion of mild steel in hydrochloric acid solutionCorros. Sci.50200833563362
- ASTM G 31-72American Society for Testing and Materials1990Philadelphia, PA
- X.H.LiS.D.DengH.FuG.N.MuN.ZhaoSynergism between rare earth cerium(IV) ion and vanillin on the corrosion of steel in H2SO4 solution: weight loss, electrochemical, UV–vis, FTIR, XPS, and AFM approachesAppl. Surf. Sci.254200855745586
- H.MaS.ChenZ.LiuY.SunTheoretical elucidation on the inhibition mechanism of pyridine–pyrazole compound: a Hartree Fock studyJ. Mol. Struct. (THEOCHEM)77420061922
- J.H.Henríquez-RománL.Padilla-CamposM.A.PáezJ.H.ZagalA.María RubioC.M.RangelJ.CostamagnaG.Cárdenas-JirónThe influence of aniline and its derivatives on the corrosion behaviour of copper in acid solution: a theoretical approachJ. Mol. Struct. (THEOCHEM)757200517
- L.M.Rodrguez-ValdezA.Martnez-VillafaneD.Glossman-MitnikComputational simulation of the molecular structure and properties of heterocyclic organic compounds with possible corrosion inhibition propertiesJ. Mol. Struct. (THEOCHEM)71320056570
- Y.FengS.ChenW.GuoY.ZhangG.LiuInhibition of iron corrosion by 5,10,15,20-tetraphenylporphyrin and 5,10,15,20-tetra-(4-chlorophenyl)porphyrin adlayers in 0.5 M H2SO4 solutionsJ. Electroanal. Chem.6022007115122
- M.J.FrischG.W.TrucksH.B.SchlegelG.E.ScuseriaM.A.RobbJ.R.CheesemanJ.A.MontgomeryJr.T.VrevenK.N.KudinJ.C.BurantJ.M.MillamS.S.IyengarJ.TomasiV.BaroneB.MennucciM.CossiG.ScalmaniN.RegaG.A.PeterssonH.NakatsujiM.HadaM.EharaK.ToyotaR.FukudaJ.HasegawaM.IshidaT.NakajimaY.HondaO.KitaoH.NakaiM.KleneX.LiJ.E.KnoxH.P.HratchianJ.B.CrossV.BakkenC.AdamoJ.JaramilloR.GompertsR.E.StratmannO.YazyevA.J.AustinR.CammiC.PomelliJ.W.OchterskiP.Y.AyalaK.MorokumaG.A.VothP.SalvadorJ.J.DannenbergV.G.ZakrzewskiS.DapprichA.D.DanielsM.C.StrainO.FarkasD.K.MalickA.D.RabuckK.RaghavachariJ.B.ForesmanJ.V.OrtizQ.CuiA.G.BaboulS.CliffordJ.CioslowskiB.B.StefanovG.LiuA.LiashenkoP.PiskorzI.KomaromiR.L.MartinD.J.FoxT.KeithM.A.Al-LahamC.Y.PengA.NanayakkaraM.ChallacombeP.M.W.GillB.JohnsonW.ChenM.W.WongC.GonzalezJ.A.PopleGaussian 03Revision E. 012004Gaussian, Inc.Wallingford CT
- M.J.S.DewarW.ThielGround states of molecules. 38. The MNDO method – approximations and parametersJ. Am. Chem. Soc.99197748994907
- R.G.PearsonAbsolute electronegativity and hardness: application to inorganic chemistryInorg. Chem.271988734740
- R.S.ChaudharyS.SharmaInfluence of auramine O on the corrosion behaviour of mild steelInd. J. Chem. Technol.61999202206
- G.K.GommaCorrosion of low-carbon steel in sulphuric acid solution in presence of pyrazole–halides mixtureMater. Chem. Phys.551998241246
- National Association of Corrosion EngineersCorrosion Basics: An Introduction1984NACE329
- A.I.OnuchukwuS.P.TrasattiS.TrasattiHydrogen permeation into aluminium AA1060 as a result of corrosion in an alkaline medium. Influence of anions in solution and of temperatureCorros. Sci.36199418151817
- I.F.FishtikI.I.VatamanF.A.SpatarThe mechanism of ion-pair formation in the inner part of the double layerJ. Electroanal. Chem.165198418
- S.MartinezI.SternInhibitory mechanism of low-carbon steel corrosion by mimosa tannin in sulphuric acid solutionsJ. Appl. Electrochem.312001973978
- S.ChengS.ChenT.LiuX.ChangY.YinCarboxymethyl chitosan as an ecofriendly inhibitor for mild steel in 1 M HClMater. Lett.61200732793280
- L.NarvaezE.CanoD.M.Bastidas3-Hydroxybenzoic acid as AISI 316L stainless steel corrosion inhibitor in a H2SO4–HF–H2O2 pickling solutionJ. Appl. Electrochem.352005499506
- W.H.DurnieB.J.KinsellaR.deMarcoA.JeffersonA study of the adsorption properties of commercial carbon dioxide corrosion inhibitor formulationsJ. Appl. Electrochem.31200112211226
- Y.H.TangW.Z.YangX.S.YinY.LiuR.WanJ.T.WangPhenyl-substituted amino thiadiazoles as corrosion inhibitors for copper in 0.5 M H2SO4Mater. Chem. Phys.1162009479483
- M.S.MoradCorrosion inhibition of mild steel in sulfamic acid solution by S-containing amino acidsJ. Appl. Electrochem.38200815091518
- M.A.QuraishiD.JamalCorrosion inhibition of N-80 steel and mild steel in 15% boiling hydrochloric acid by a triazole compound – SAHMTMater. Chem. Phys.682001283287
- R.AgrawalT.K.G.NamboodhiriThe inhibition of sulphuric acid corrosion of 410 stainless steel by thioureasCorros. Sci.3019903752
- D.DoAdsorption Analysis: Equilibria and Kinetics1980Imperial College Press
- T.P.ZhaoG.N.MuThe adsorption and corrosion inhibition of anion surfactants on aluminium surface in hydrochloric acidCorros. Sci.41199919371944
- A.S.YaroA.A.KhadomH.F.IbraheemPeach juice as an anti-corrosion inhibitor of mild steelAnti Corros. Methods Mater.52011116124
- F.M.DonahueK.NobeTheory of organic corrosion inhibitorsJ. Electrochem. Soc.1121965886891
- E.KhamisF.BellucciR.M.LatanisionE.S.H.El-AshryAcid corrosion inhibition of nickel by 2-(triphenosphoranylidene) succinic anhydrideCorrosion471991677686
- A.YurtG.BereketA.KivrakA.BalabanB.ErkEffect of Schiff bases containing pyridyl group as corrosion inhibitors for low carbon steel in 0.1 M HClJ. Appl. Electrochem.35200510251032
- A.M.FekryR.R.MohamedAcetyl thiourea chitosan as an eco-friendly inhibitor for mild steel in sulphuric acid mediumElectrochim. Acta55201019331939
- V.R.SaliyanA.V.AdhikariQuinolin-5-ylmethylene-3-{[8-(trifluoromethyl)quinolin-4-yl]thio}propanohydrazide as an effective inhibitor of mild steel corrosion in HCl solutionCorros. Sci.5020085561
- L.M.VračarD.M.DražiAdsorption and corrosion inhibitive properties of some organic molecules on iron electrode in sulfuric acidCorros. Sci.44200216691680
- E.S.FerreiraC.GiancomelliF.C.GiacomelliA.SpinelliEvaluation of the inhibitor effect of l-ascorbic acid on the corrosion of mild steelMater. Chem. Phys.832004129134
- F.M.MahgoubB.A.Abdel-NabeyY.A.El-SamadisyAdopting a multipurpose inhibitor to control corrosion of ferrous alloys in cooling water systemsMater. Chem. Phys.1202010104108
- W.R.FawcettZ.KovacovaA.J.MotheoC.A.FossApplication of the AC admittance technique to double layer studies on polycrystalline gold electrodesJ. Electroanal. Chem.326199291103
- K.JuttnerElectrochemical impedance spectroscopy (EIS) of corrosion processes on inhomogeneous surfacesElectrochim. Acta35199015011508
- S.K.ShuklaM.A.Quraishi4-Substituted anilinomethylpropionate: new and efficient corrosion inhibitors for mild steel in hydrochloric acid solutionCorros. Sci.51200919901997
- F.MansfeldRecording and analysis of AC impedance data for corrosion studies: background and method of analysisCorrosion371981301307
- J.R.MacdonaldImpedance spectroscopy and its use in analyzing the steady-state AC response of solid and liquid electrolytesJ. Electroanal. Chem. Int. Electrochem.22319872550
- U.RammeltG.ReinhardThe influence of surface roughness on the impedance data for iron electrodes in acid solutionsCorros. Sci.271987373382
- F.B.GrowcockJ.H.JasinskiTime resolved impedance spectroscopy of mild steel in concentrated hydrochloric acidJ. Electrochem. Soc.136198923102314
- J.PangA.BricenoS.ChanderA study of pyrite/solution interface by impedance spectroscopyJ. Electrochem. Soc.137199034473455
- K.C.EmregulO.AtakolCorrosion inhibition of iron in 1 M HCl solution with Schiff base compounds and derivativesMater. Chem. Phys.832004373379
- I.L.RosenfieldCorrosion Inhibitors1981McGraw-HillNew York
- M.MaCaffertyN.HackermanDouble layer capacitance of iron and corrosion inhibition with polymethylene diaminesJ. Electrochim. Soc.1191972146154
- M.HosseiniS.F.L.MertensM.GhorbaniR.M.ArshadiAsymmetrical Schiff bases as inhibitors of mild steel corrosion in sulphuric acid mediaMater. Chem. Phys.782003800808
- N.O.Obi-EgbediI.B.ObotM.I.El-KhaiaryQuantum chemical investigation and statistical analysis of the relationship between corrosion inhibition efficiency and molecular structure of xanthene and its derivatives on mild steel in sulphuric acidJ. Mol. Struct.100220118696
- E.E.EbensoM.M.KabandaT.ArslanM.SaracogluF.KandemirliL.C.MurulanaA.K.SinghS.K.ShuklaB.HammoutiK.F.KhaledM.A.QuraishiI.B.ObotN.O.EddyQuantum chemical investigations on quinoline derivatives as effective corrosion inhibitors for mild steel in acidic mediumInt. J. Electrochem. Sci.7201256435676
- M.M.KabandaL.C.MurulanaM.OzcanF.KaradagI.DehriI.B.ObotE.E.EbensoQuantum chemical studies on the corrosion inhibition of mild steel by some triazoles and benzimidazole derivatives in acidic mediumInt. J. Electrochem. Sci.7201250355056
- N.O.Obi-EgbediI.B.ObotM.I.El-KhaiaryS.A.UmorenE.E.EbensoComputational simulation and statistical analysis on the relationship between corrosion inhibition efficiency and molecular structure of some phenanthroline derivatives on mild steel surfaceInt. J. Electrochem. Sci.7201256495675
- I.B.ObotN.O.Obi-EgbediA.O.EseolaAnticorrosion potential of 2-mesityl-1H-imidazo[4,5-f][1,10]phenanthroline on mild steel in sulfuric acid solution: experimental and theoretical studyInd. Eng. Chem. Res.50201120982110
- A.AytacS.BilgicG.GeceN.AncinS.G.OztasExperimental and theoretical study of the inhibition effects of some Schiff bases as corrosion inhibitors of aluminium in HClMater. Corros.6382012729734
- R.G.PearsonRecent advances in the concept of hard and soft acids and basesJ. Chem. Educ.641987561567
- M.K.AwadM.R.MustafaM.M.Abo ElngaComputational simulation of the molecular structure of some triazoles as inhibitors for the corrosion of metal surfaceJ. Mol. Struct. (THEOCHEM)95920106674
- A.Y.MusaA.H.KadhumA.B.MohamadA.b.RohomaH.MesmariElectrochemical and quantum chemical calculations on 4,4-dimethyloxazolidine-2-thione as inhibitor for mild steel corrosion in hydrochloric acidJ. Mol. Struct.9692010233327
- M.A.AminK.F.KhaledS.A.Fadl-AllahTesting validity of the Tafel extrapolation method for monitoring corrosion of cold rolled steel in HCl solutions – experimental and theoretical studiesCorros. Sci.522010140151
- G.GeceS.BilgicQuantum chemical study of some cyclic nitrogen compounds as corrosion inhibitors of steel in NaCl mediaCorros. Sci.51200918761878
- O.KikuchiSystematic QSAR procedures with quantum chemical descriptorsQuant. Struct.: Act. Relat.61987179184
- E.E.EbensoD.A.IsabiryeN.O.EddyAdsorptionQuantum chemical studies on the inhibition potentials of some thiosemicarbazides for the corrosion of mild steel in acidic mediumInt. J. Mol. Sci.11201024733249
- I.AhamadS.KhanK.R.AnsariM.A.QuraishiPrimaquine: a pharmaceutically active compound as corrosion inhibitor for mild steel in hydrochloric acid solutionJ. Chem. Pharm. Res.32011703717