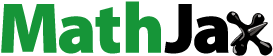
Abstract
Among the most promising nanomaterials, metallic nanoparticles with antibacterial and antitumor properties are expected to open new avenues to fight and prevent various tumours and infectious diseases. The study of bactericidal nanomaterial is particularly timely considering the recent increase in new resistant strains of bacteria to the most potent antibiotics and the potential role of bactericidal nanomaterial as anticancer agents. This has promoted the research of the well-known activity of silver ions and silver-based compounds, including silver nanoparticles. The present work is the study of silver nanoparticles synthesized from Penicillium brevicompactum (MTCC-1999). The colour of the cell filtrate changes to dark brown upon addition of 1 mM AgNO3, suggesting the formation of silver nanoparticles. These silver nanoparticles (AgNPs) were characterized and analyzed by UV–vis spectrophotometric analysis, which showed a peak of absorbance at 420 nm. Fourier transform infrared (FTIR) analysis showed amines and amides that are responsible for the stabilization of AgNPs. To determine the particle size, atomic force microscopy (AFM) analysis was used, which showed that the nanoparticles are spherical and are 30–50 nm in size. High-resolution transmission electron microscopy (HRTEM) showed that AgNPs were well dispersed, spherical, and well within the range of 40–50 nm. These nanoparticles displayed good antibacterial activity and also increased the antibiotic activity of gatifloxacin, tetracycline, and vancomycin. These nanoparticles were further studied for their anticancer activity and showed high toxicity towards the MCF-7 breast cancer cell line.
1 Introduction
Nanobiotechnology is a promising and advanced area of science and technology that utilizes noble metals at a nano-scale level. Nanobiotechnology also has immense applications in various sectors of life, especially in the field of medicine. Silver and silver nanoparticles are important materials that are used in the fields of biomedicine and biotechnology, as well as in industrial applications, such as biosensors and catalytic, therapeutic and antibacterial materials [Citation1–Citation3]. Various chemical, physical, and biological methods are now available to synthesize silver nanoparticles. The biological method for the synthesis of nanoparticles is preferred over other methods because it is inexpensive, free from toxic materials, safe, and environmentally friendly [Citation4].
Some pathogenic bacteria have become resistant to various antibiotics, which can make it challenging to identify new antibacterial material that can control bacterial resistance. The antibacterial activities of silver and silver-based ions are well known [Citation5,Citation6]. Several experts have described various applications of nanosilver and silver nanoparticles, including for catheters and wound healing [Citation7]. Many experts have proposed various mechanisms regarding the effect of silver nanoparticles on bacteria and the various target sites where nanoparticles attack bacteria. The unique shape, small and controlled size, and high surface area give silver nanoparticles time to interact with the bacterial cell membrane where they release metal ions. The metal ions enter the cell by binding to the phosphorus and nitrogen groups of DNA strands, which causes damage to DNA by breaking the strands and subsequently causing cell death [Citation8,Citation9].
In the present study, we focused on the biosynthesis of extracellular AgNPs synthesized from the filamentous fungus Penicillium brevicompactum (MTCC-1999). The biosyntheses of several nanoparticles were characterized to determine their potential antibacterial effects and synergistic effects with antibiotics (e.g., tetracycline, gatifloxacin, and vancomycin). These nanoparticles were also tested for their anticancer activity against the MCF-7 breast cancer cell line by utilizing the MTT assay.
2 Material and methods
2.1 Collection of samples
The fungal strain P. brevicompactum (MTCC-1999) was obtained from the Institute of Microbial Technology (IMTECH) in Chandigarh, India and subcultured on potato dextrose agar media (PDA) to regulate its activity for the duration of the study. The subcultures were performed under strict laboratory conditions. A fresh fungal culture was prepared as needed for the duration of the study.
2.2 Biosynthesis of silver nanoparticles
P. brevicompactum (MTCC-1999) fungal species was utilized for the production of silver nanoparticles. Biomass production was carried out by growing the fungal strain in aerobic conditions [in a 250-ml flask containing 100 ml of potato dextrose broth (PDB)] at 30 °C for 72 h in a shaker. After incubation, the biomass of the fungal strain was collected by filtering the media through Whatman filter paper No.1. The biomass was then washed with sterile distilled water to remove any remnants of the media. The resulting clean and fresh biomass of the fungal strains were taken into the 200-ml flasks containing 100 ml of deionized Milli-Q water, mixed thoroughly, and then kept in a shaker for incubation at 30 °C for 72 h at 150 rpm. After 72 h of incubation, clean and fresh biomass was sonicated and filtered through Whatman filter paper No.1. The cell filtrate was used for the production of the nanoparticles. Then, 1 mM AgNO3 prepared in 50 ml of Milli-Q water was mixed with 50 ml of the cell filtrate extract, agitated, and kept in a shaker at 30 °C in the dark overnight. Fungal cell filtrate without silver nitrate (which served as a control) was maintained along with the experimental flask.
2.3 Characterization of silver nanoparticles
When a change in the colour of the cell filtrate upon addition of silver nitrate was observed, that absorbance was measured using a UV–visible spectrophotometer. A sample of 1 ml was taken after 24 h, and the maximum absorbance was measured with the reference range between 300 and 600 nm. FTIR was used to analyze possible functional groups, biomolecules, and proteins that play an important role in the reduction of AgNO3 to AgNPs. Additionally, other molecules that are involved in the stabilization of nanoparticles were used as capping agents in the P. brevicompactum (MTCC-1999) cell filtrate extract. For FTIR analysis, dried powder from AgNPs was mixed with potassium bromide (KBr), and the dried pellet was analyzed using the infrared spectra at a wavelength range of 500–4000 cm−1.
AFM analysis was used to check the particle size and shape of the nanoparticles. For AFM analysis, each sample was sonicated for 10 min, followed by centrifugation at 15,000 rpm for 10 min. Then, a small thin film of the pellet on a glass slide was prepared and examined under a microscope.
For the TEM analysis of silver nanoparticles, the samples were prepared by placing a drop of the AgNP suspension on a carbon-coated copper grid and allowing the samples to evaporate inside a vacuum dryer. The samples were then scanned at a high resolution.
2.4 Antimicrobial activity
Biologically synthesized AgNPs using P. brevicompactum (MTCC-1999) were used to check for its antibacterial activity by the disc diffusion method. The antimicrobial activities of the AgNPs were tested against several isolated pathogenic bacteria: Staphylococcus epidermidis, Staphylococcus aureus, Vibro cholerae, Proteus vulgaris, and Escherichia coli. Furthermore, to determine if there was a synergistic effect, the AgNPs were evaluated with antibiotics such as tetracycline, gatifloxacin and vancomycin. The inhibition zone was measured after overnight incubation at 37 °C [Citation10].
2.5 Anticancer assay of silver nanoparticles
The anticancer effect of AgNPs on MCF-7 breast cancer cells was determined using MTT (3-(4,5-dimethylthiazol-2-yl)-2,5-diphenyltetrazolium bromide) colorimetric technique (National Institute of Cell Science (NICS), Poona). The AgNPs were first weighed, and the required nanoparticles solution was prepared in sterile double-distilled water and diluted to the required concentrations (10, 30, 50, 70, 90, and 110 μg/mL) using the cell culture medium. The required concentrations of AgNPs were then added to the cell cultures to obtain the final concentration of AgNPs and incubated for 24 and 48 h at 37 °C. Cells treated without the nanoparticles were used as controls. After 24 and 48 h of incubation, the cells were washed with phosphate buffer solution, and then 100 μL of the yellow tetrazolium MTT solution (3-(4,5-dimethylthiazol-2-yl)-2,5-diphenyltetrazolium bromide) was added to each microtiter well. Microtiter plates were incubated for 2–3 h at 37 °C for the reduction of the MTT dye (NADH to NADPH) by normal living cells. Then, 100 μL of DMSO was added to the each well to solubilize the MTT crystals. These plates were kept in a shaker for 10–15 min to allow for complete crystal formation so that the crystals could solubilize properly. The cytotoxicity effect on MCF-7 cells was detected by eluting the dye with DMSO, and the optical density was measured using spectrophotometer. Optical density (OD) values were read at 595 nm. All experiments were performed in triplicates. A cell control and sample were included in each assay to compare the full cell viability after cytotoxicity. The percentage of cell viability was calculated using the following formula:
3 Results and discussion
For the current study, the fungus P. brevicompactum (MTCC-1999) was utilized for the extracellular biosynthesis of AgNPs, and 1 mM AgNO3 was added to the cell-free filtrate. The solution colour changes to dark brown indicating nanoparticle formation (). These nanoparticles were further analyzed by UV–visible spectrophotometry, which showed a peak absorption at 420 nm due to the interband transition and plasmon vibration of the nanoparticles () [Citation11,Citation12]. Those observations are consistent with the qualitative analysis of silver nanoparticles.
Fig. 1 Synthesis of silver nanoparticles from P. brevicompactum (MTCC-1999). (A) Before AgNO3. (B) After AgNO3.
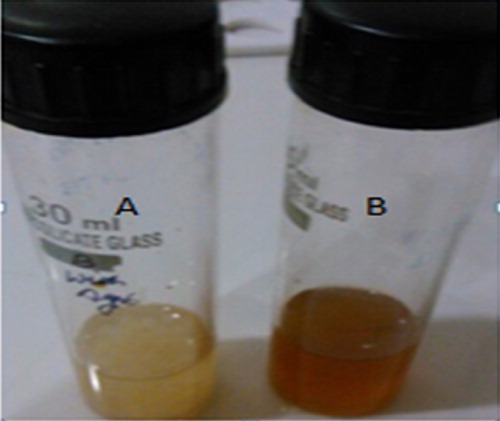
Fig. 2 UV analysis of AgNPs synthesized from P. brevicompactum (MTCC-1999). Absorption peak of cell filtrate with AgNPs (A) and cell filtrate without AgNPs (B).
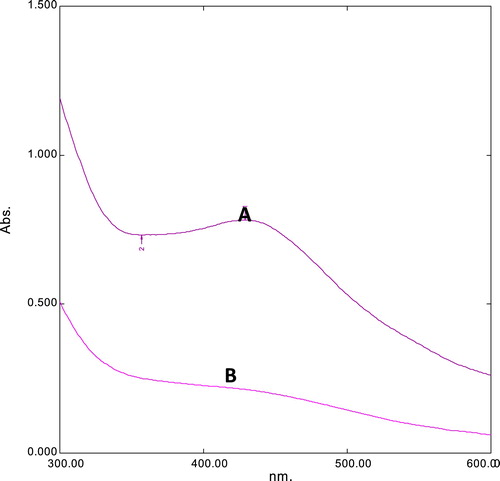
The FTIR analysis was used for the characterization of AgNPs in cell filtrate extract from P. brevicompactum (MTCC-1999). The IR analysis showed the following: the sharp peaks at 3425.4 cm−1 were indicative of the N–H stretching of amides, the peak at 1643.2 cm−1 were attributed to the N–H bending of amines, the peak at 1380.9 cm−1 was ascribed to the NO2 asymmetric stretching of nitro compounds, and the peak at 1080 cm−1 was attributed to the C–N stretching of amines. Certain small peaks were observed at 864.4 cm−1 (N–H wag of amines), 617.17 cm−1 (C–Cl stretch of alkyl halides) and 540.02 cm−1 (C–Br stretch of alkyl halides). Sharp and prominent broad peaks showed that amide and amine bonding was involved in the capping of silver nanoparticles, which play an important role in stabilizing nanoparticles. However, there were some weak peaks (864.4 cm−1, 617.17 cm−1, and 540.02 cm−1), which were negligible and not observed as prominent groups (see ).
AFM analysis was used to check the particle size of nanoparticles. A two-dimensional horizontal cross section of the AgNPs indicated that the nanoparticles were well defined, spherical, and between 30 and 50 nm in size. A histogram analysis revealed that the average particle size was approximately 40 nm. The three-dimensional image showed the average roughness and inhomogeneity of the cluster formation of nanoparticles ().
Fig. 4 AFM analysis of AgNPs. (A) 2D image of the AgNP horizontal cross section. (B) Line profiling. (C) 3D image of AgNPs. (D) Histogram analysis showing average particle size.
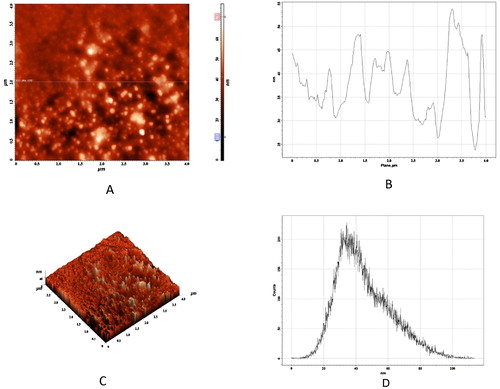
TEM analysis revealed that the average size of the AgNPs was between 30 and 45 nm, and the small particles were well-dispersed and spherical in morphology, as shown in . The selected area of the electron diffraction pattern showed continuous ring patterns which originated from the polycrystalline state. This observation indicated that there were crystallites that attached to the surface of single AgNPs.
Antibacterial analysis of AgNPs synthesized from P. brevicampactum was checked against S. epidermidis, V. cholerae, E. coli, S. aureus, and P. vulgaris by the disc diffusion method. Each disc was impregnated with 30 μg of AgNPs to carry out the investigation. Each antibiotic disc of tetracycline and gatifloxacin was evaluated alone and in combination with the nanoparticles (antibiotic discs were impregnated with 15 μg/disc of nanoparticles to determine the synergistic effects against the bacterial pathogens). The synthesized AgNPs showed good antibacterial activity. Antibiotics showed good antibacterial activity alone and also in combination with AgNPs, with a high antimicrobial property rating, as shown in [Citation13]. In the case of gatifloxacin, the presence of AgNPs in the highest zone of inhibition was observed with E. coli (35 mM) followed by P. vulgaris (32 mM). For tetracycline, highest zone of inhibition was observed with E. coli (22 mM) followed by S. aureus (20 mM). For vancomycin, the highest zone of inhibition was observed with P. vulgaris (31 mM) followed by V. cholerae (30 mM). Each experimental procedure was repeated three times, and the standard deviation (SD) was applied to calculate the average values. and show the graphical representation of the combined effects of AgNPs with tetracycline, gatifloxacin, and vancomycin [Citation14].
Table 1 Inhibition zone (mM) of tetracycline, gatifloxacin and vancomycin against bacterial pathogens in absence and presence of silver nanoparticles.
The exact mechanism of how nanoparticles inhibit the growth of bacteria is not yet known, but several hypotheses indicate that silver nanoparticles attack the enzymes present inside the bacteria, which causes morphological changes that lead to DNA damage. This DNA damage could result from the production of free radicals, which attack the phosphorus groups of DNA and eventually inhibits the growth of bacteria [Citation13].
An anticancer analysis (via MTT assay) was carried out to check the efficacy of AgNPs on MCF-7 cancer cells. These AgNPs showed a concentration-dependent anticancer effect on MCF-7 cells. The IC50 value was 70 μg/ml after 24 h of incubation. Upon further incubation for 48 h, the toxicity increased and the IC50 value was 50 μg/ml, as shown in . Similar observations were previously reported by other investigators [Citation15,Citation16]. Thus, these nanoparticles display anticancer activity against MCF-7 breast cancer cells and can be used as anticancer and antibacterial agents. However, animal studies are needed to determine the in vivo toxicity of AgNPs before clinical applications can be considered.
4 Conclusions
AgNPs synthesized from fungi P. brevicompactum (MTCC-1999) display antibacterial activity against clinically isolated bacterial pathogens and also enhance the antibacterial activity of antibiotics. These nanoparticles also display anticancer activity against MCF-7 breast cancer cells, which suggests that AgNPs could also be used as anticancer agents. To that end, further cytotoxicity studies and animal trials have to be carried out before considering the clinical use of AgNPs.
Acknowledgements
We acknowledge the Department of Biomedical Engineering at the Sathyabama University in Chennai, India, and also the Department of Pharmacy at the University of Kuala Lumpur, Royal College of Medicine in Ipoh, Malaysia, for providing the necessary facilities to carry out the research work.
Notes
Peer review under responsibility of Taibah University.
References
- M.RaiA.YadavA.GadeSilver nanoparticles as a new generation of antimicrobialsBiotechnol. Adv.2720097683
- P.D.MarcatoN.DuranNew aspects of nano pharmaceutical delivery systemsJ. Nanosci. Nanotechnol.8200822162229
- N.DuránP.D.MarcatoR.D.ContiO.L.AlvesF.T.M.CostaM.BrocchiPotential use of silver nanoparticles on pathogenic bacteria, their toxicity and possible mechanisms of actionJ. Braz. Chem. Soc.212010949959
- C.BuzeaI.I.PachecoK.RobbieNanomaterials and nanoparticles: sources and toxicityBiointerphases22007771
- V.Siva KumarB.M.NagarajaV.ShashikalaA.H.PadmasriS.S.MadhavendraB.D.Rajuet al.Highly efficient Ag/C catalyst prepared by electro-chemical deposition method in controlling microorganisms in waterJ. Mol. Catal. A Chem.2232004313319
- I.SondiB.Salopek-SondiSilver nanoparticles as antimicrobial agent: a case study on E. coli as a model for gram-negative bacteriaJ. Colloid Interface Sci.2752004177182
- J.S.KimAntimicrobial effects of silver nanoparticlesNanomedicine3200795101
- D.W.BrettA discussion of silver as an antimicrobial agent: alleviating the confusionOstomy Wound Manag.5220063441
- E.HidalgoC.DominguezStudy of cytotoxicity mechanisms of silver nitrate in human dermal fibroblastsJ. Toxicol. Lett.981998169179
- A.W.BauerM.KirbyJ.C.SherrisM.TruckAntibiotic susceptibility testing by a standardized single disk methodAm. J. Clin. Pathol.451996493496
- S.S.ShankarA.RaiA.AhmadM.SastryRapid synthesis of Au, Ag and Au core-Ag shell nanoparticles using Neem (Azadiracta indica) leaf brothJ. Colloid Interface Sci.2752004496502
- P.MulvaneySurface plasmon spectrocopy of nanosized metal particlesLangmuir121996788800
- A.NandaS.MajeedEnhanced antibacterial efficacy of biosynthesized AgNPs from Penicillium glabrum (MTCC1985) pooled with different drugsInt. J. PharmTech Res.62014217223
- S.PalY.K.TakJ.M.SongDoes the antimicrobial activity of silver nanoparticles depend on the shape of the nanoparticle? A study of the gram-negative bacterium Escherichia coliJ. Appl. Environ. Microbiol.73200717121720
- M.SafaepourA.R.ShahverdiH.R.ShahverdiM.R.KhorramizadehA.R.GohariGreen synthesis of small silver nanoparticles using geraniol and its cytotoxicity against Fibrosarcoma-Wehi 164Avicenna J. Med. Biotechnol.12009111115
- M.I.SriramS.B.M.KanthK.KalishwaralalS.GurunathanAntitumor activity of silver nanoparticles in Dalton's lymphoma ascites tumor modelInt. J. Nanomed.52010753762