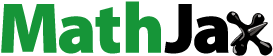
Abstract
A CaO-supported ZnWO4 nanocomposite (ZnWO4/CaO) was successfully synthesized using a novel hydrothermal method and was characterized by scanning electron microscopy (SEM), tunnelling electron microscopy (TEM), X-ray diffraction (XRD), electron diffraction X-ray (EDX), Fourier transform infrared spectroscopy (FTIR) and UV–visible (UV–vis) spectral analysis. The ZnWO4/CaO composites exhibited rod-like morphologies with variable lengths from 45 nm to 147 nm and diameters from 26 nm to 36 nm. The catalytic efficiency of the synthesized ZnWO4/CaO composites was displayed for the photodegradation of oxytetracycline (OTC) antibiotic from the aqueous phase. The synergistic degradation of OTC was investigated in the presence of H2O2 and ZnWO4/CaO. The rate of photodegradation followed pseudo-first-order kinetics. The antibiotic removal was strongly influenced by the catalyst loading, H2O2 concentration, pH and OTC concentration. Using a solar/H2O2/ZnWO4/CaO catalytic system, 85% COD removal was attained for OTC degradation in 210 min. The oxidative degradation occurred through hydroxyl radicals. The prepared nanocomposites possessed high recyclability and were easily separated from the aqueous solution by a simple sedimentation process.
1 Introduction
Antibiotics represent a class of chemicals that are used worldwide in pharmaceuticals and personal care products (PPCPs) and are toxic to the ecosystem [Citation1,Citation2]. Tetracycline (TC) as an important member of the antibiotic group is used for disease treatment, prevention and growth promoters [Citation3,Citation4]. Among all of the antibiotics, oxytetracycline (OTC) is one of the most frequently detected tetracyclines in water bodies and sediments [Citation5,Citation6]. The traditional methods of wastewater decontamination, such as coagulation, flocculation, precipitation, adsorption, membrane separation techniques and aerobic biological treatment, prove inefficient for the complete degradation of organic pollutants into innocuous products but have been used for the removal of antibiotics from wastewater [Citation7,Citation8].
Photocatalytic degradation of organic pollutants by semiconductor photocatalysts has emerged as a potential technology for water purification. The advanced oxidation process is a promising technology due to its low cost, simplicity and high efficiency as well as its ability to generate no secondary pollution [Citation9–Citation11]. When a photocatalyst is excited with photons of energy equal to or higher than its band gap energy, electrons (e−) and hole (h+) pairs are created. The photogenerated holes migrate to the hydroxylated surface and produce highly reactive OH• species (oxidation potential = 2.8 eV). The oxidative degradation occurs through hydroxyl radicals that degrade the organic/inorganic pollutants present in the aqueous phase [Citation12].
Binary metal oxides such as TiO2, ZnO, Cu2O, WO3, V2O5, NiO, ZrO2, and CeO2 have been synthesized and investigated for photocatalytic water purification [Citation12,Citation13]. In this context, as a member of the metal tungstate family, ZnWO4 is emerging as a promising photocatalyst due to its non-toxicity, low cost, high chemical stability and visible light activity [Citation14]. He et al. prepared a Bi2WO6/ZnWO4 composite via a simple hydrothermal method involving the growth of Bi2WO6 nanoparticles on ZnWO4 nanorods [Citation15]. Zhang et al. studied the band structure, morphology and photocatalytic activity of ZnWO4 for the photodegradation of rhodamine blue dye from the aqueous phase [Citation16]. Bai et al. explored the visible light activity of graphene–ZnWO4 nanocomposites for the degradation of methylene blue dye [Citation17].
The adsorption of pollutant onto the catalyst surface is required for the efficient photodegradation process in the aqueous phase [Citation7,Citation10,Citation12]. However, the large-scale application of ZnWO4 is hindered due to the low adsorptional photocatalytic activity [Citation4]. Therefore, the improvement of the adsorptional photocatalytic activity of ZnWO4 still poses a challenge. Calcium oxide (CaO) is used as an industrial compound, catalyst, toxic-waste remediation agent, an additive in refractory processes and for other fundamental applications [Citation18]. Considerable attention has been focused on calcium oxide because it has high CO2 adsorption capacity and high raw material availability (e.g., limestone) at a low cost [Citation19]. H2O2 is the simplest oxidant that reduces a low concentration of pollutants present in wastewater [Citation20]. Under UV light, H2O2 decomposes and generates OH• radicals, which are responsible for the destruction of target pollutants. The use of H2O2 becomes more effective when used in conjunction with other reagents or energy sources capable of dissociating it to generate OH• radicals [Citation21].
The current studies have emphasized the applicability of ZnWO4/CaO composites as a photocatalyst for the degradation of oxytetracycline in the aqueous phase. A facile hydrothermal method was used to synthesize ZnWO4/CaO composites. The ZnWO4/CaO catalyst was characterized by scanning electron microscopy (SEM), transmission electron microscopy (TEM), X-ray diffraction (XRD), energy dispersive X-ray analysis (EDX), Fourier transform infrared (FTIR) and ultraviolet–visible (UV–vis) spectral techniques. The influence of various reaction parameters was evaluated on the photodegradation of OTC. The synergistic effect of adsorption and photocatalysis with the most plausible mechanism on the photodegradation of OTC was also explored. The use of solar light was preferred over an expensive artificial source. Additionally, the recovery and recyclability of the ZnWO4/CaO catalyst was examined.
2 Experimental
2.1 Materials
All of the chemicals used in this study were of analytical grade. Oxytetracycline antibiotic, sodium tungstate dihydrate (Na2WO4·2H2O) and zinc nitrate hexahydrate [Zn(NO3)2·6H2O] were purchased from Sigma Aldrich. Hen eggshells were collected from a local market in Solan, Himachal Pradesh, India. All of the solutions were prepared in double distilled water.
2.2 Preparation of CaO
The hen eggshells were washed with distilled water to remove any adhered impurities. The washed hen eggshells were boiled and heated at 800 °C for 2 h to obtain CaO (Eq. Equation(1)(1)
(1) ). The obtained CaO was dried, ground and sieved to a fragment size less than 200 mesh and preserved for further use:
(1)
(1)
2.3 Preparation of ZnWO4/CaO composites
The ZnWO4/CaO composites were prepared with little modification in the hydrothermal method [Citation22]. In a typical synthesis, a 0.1 M of sodium tungstate dehydrate solution and 0.1 M of zinc nitrate hexahydrate were mixed in 50 ml of distilled water and sonicated for 30 min. After sonicating the mixture, 6.272 g of CaO was added with continuous stirring for 4 h at 70 °C (Eq. Equation(2)(2)
(2) ). The precipitates were filtered, washed with ethanol and heated in a muffle furnace at 400 °C for 2 h. The obtained composites were levelled as ZnWO4/CaO and preserved for further use:
(2)
(2)
2.4 Photocatalytic activity
Photocatalytic, photolytic and dark experiments were executed in a double-walled Pyrex vessel (7.5-cm high × 6-cm wide) surrounded by a thermostatic water circulation arrangement (30 ± 0.3 °C). During the equilibration experiments, the slurry composed of OTC and the ZnWO4/CaO catalyst suspension was continuously stirred and kept under solar light [Citation23,Citation24]. At specific time intervals, an aliquot (3 ml) was withdrawn and centrifuged for 2 min to remove the catalyst particles from the aliquot. The absorbance of OTC was observed at 280 nm. The solar light intensity was measured by a digital lux-metre (35 × 103 ± 1000 lx). Photocatalytic experiments were performed between March and May 2014 (11 am–2 pm). All of the experiments were undertaken in triplicate with errors below 5% and with the average values reported. The chemical oxygen demand (COD) was measured by the closed reflux method. The unreacted oxidant was determined by titrating the sample with ferrous ammonium sulphate using a ferroin indicator. The removal efficiency was calculated using Eq. Equation(3)(3)
(3) [Citation25]:
(3)
(3) where C0 is the initial concentration of sample/COD and Ct/COD is the instant concentration of sample/COD.
The kinetics of OTC degradation was explained by pseudo-first-order kinetics. The rate constant (k) and half-life period (t1/2) were calculated using Eqs. Equation(4)(4)
(4) and Equation(5)
(5)
(5) , respectively [Citation25]:
(4)
(4)
(5)
(5) where the slope was obtained from the plot of ln(c) versus ‘t’.
3 Results and discussion
3.1 Characterization of the ZnWO4/CaO composites
depicts the SEM images of pure CaO (a–b) and ZnWO4/CaO composites (c–d). CaO exhibits a porous surface, which facilitates the adsorption of organic/inorganic molecules. (c) and (d) reveals that ZnWO4 nanoparticles were unevenly distributed on the surface of the pure 200–nm CaO microsphere. The incorporation of ZnWO4 onto the CaO surface reduced the porosity of the composite.
The TEM images of the ZnWO4/CaO composites are shown in (a)–(c). These images indicate that the nanocomposite exhibits a rod-like structure, with spherical particles arranged in an irregular fashion [Citation26]. The particle length varied from 45 nm to 147 nm and the diameter from 26 nm to 36 nm. The dark surface in the images reveals the attachments of ZnWO4 onto the light grey surface of CaO.
(a) shows the UV–vis absorption spectra of the ZnWO4/CaO composite in ethanol. The absorption edge was located at approximately 360 nm. The band gap of the ZnWO4/CaO composite was calculated using the Tauc relationship [Citation12]:(6)
(6) where α is the absorption coefficient = 2.303 A/l, Eg is the optical band gap, B is the band tailing parameter, hυ is the photon energy and n = ½ for a direct band gap. The optical band gap is determined by extrapolating the straight portion of the curve between (αhυ)2 and hυ when α = 0. The band gap of the ZnWO4/CaO composite was 3.44 eV.
Fig. 3 (a) UV–vis absorbance spectra of ZnWO4/CaO composites. (b) X-ray diffraction pattern of pure CaO and ZnWO4/CaO composites. (c, d) EDX pattern of pure CaO and ZnWO4/CaO composites.
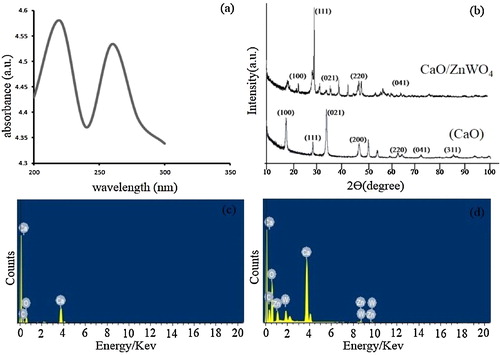
(b) shows the XRD diffractogram of the CaO and ZnWO4/CaO composites. The diffraction pattern of the prepared CaO corresponded to the (111), (220) and (311) planes, which were in good agreement with the standard JCPDS file (No. 82-1691) [Citation28]. The planes of (100), (111), (021), (200), (200), (220), (041) and (311) confirmed the presence of the ZnWO4/CaO composite according to standard JCPDS (No. 15-0774) and (No. 82-169) [Citation27,Citation28]. The characteristic peaks of CaO were observed at 47.123° and 84.743°. The diffraction peaks of the ZnWO4/CaO composite were observed at 22.206° and 28.707°. These shifts of a few plane peaks were due to the attachment of ZnWO4 to CaO. The semi-crystallites of the ZnWO4/CaO composites were in the range of 26–50 nm.
(c) and (d) shows the EDX pattern of CaO and the ZnWO4/CaO composites. The EDX spectrum confirmed the presence of elements Ca, O, C in the CaO sample ((c)). The presence of the Ca, O, C, W and Zn elements indicated the successful preparation of ZnWO4/CaO composites in (d).
illustrates the IR spectra of CaO and the ZnWO4/CaO composite. In the CaO spectrum, the peak at 712 cm−1 was due to Ca–O bonding. The peaks at 2982 cm−1, 2875 cm−1, 2516 cm−1 and 1798 cm−1 were assigned to the amines and amides of the eggshell membrane [Citation29]. The absorption peaks at 1417 cm−1, 866 cm−1 and 3420 cm−1 corresponded to C–O and O–H bonds [Citation30]. The peaks at 592 cm−1 and 798 cm−1 were due to the bending and stretching vibrations of W–O, respectively. The peak at 874 cm−1 was ascribed to the bending and stretching vibrations of Zn–O–W [Citation31]. The peaks at 1384 cm−1, 1628 cm−1 and 3411 cm−1 were due to the O–H stretching and the H–O–H bending vibrations [Citation32]. The weak absorption peaks at 2874 cm−1 and 2985 cm−1 were assigned to C–O vibrations [Citation33].
3.2 OTC removal under different catalytic systems
Photocatalytic degradation of OTC was explored using different catalytic systems under solar light and in the dark. Under solar light, 85% of OTC was removed using the H2O2/ZnWO4/CaO system ((a)), while 50% and 30% of OTC was removed utilizing the ZnWO4 and H2O2 systems, respectively. In the absence of solar light, 45% and 43% of OTC was removed utilizing the H2O2/ZnWO4/CaO and ZnWO4/CaO systems, respectively ((b)). In the dark, H2O2 alone had no effect on OTC removal. The OTC removal followed the trend of H2O2/ZnWO4/CaO (solar light) > ZnWO4/CaO (solar light) > H2O2/ZnWO4/CaO (dark) ≈ ZnWO4/CaO (dark) > H2O2 (solar light) ≫ solar light ≈ H2O2 (dark). Under the given experimental conditions, the solar/H2O2/ZnWO4/CaO system exhibited a higher efficiency for the removal of OTC.
Fig. 5 (a, b) Time profile of OTC degradation in the H2O2/CaO/ZnWO4 system under different reaction conditions (a) solar and (b) in the dark. (c) Time profile for adsorptional photocatalytic degradation of OTC. (d) The plot of log (absorbance) vs. time. Experimental conditions: [OTC] = 1 × 10−4 M, [H2O2] = 1 × 10−4 M, Catalyst dosage = 50 mg/50 ml, pH 5, temperature = 30 ± 0.3 °C and time = 70 min.
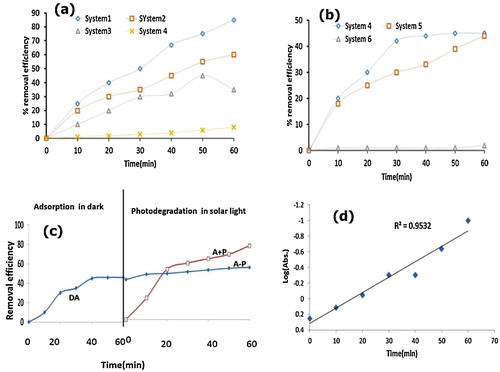
To investigate the role of adsorption in photodegradation, OTC removal using solar/H2O2/ZnWO4/CaO was investigated under three different conditions, i.e., adsorption in the dark (DA), equilibrium adsorption followed by photodegradation (A − P) and simultaneous adsorption and photocatalysis (A + P). (c) shows the removal efficiency of H2O2/ZnWO4/CaO as a function of time using DA, A − P and A + P. The first portion of the graph displays the adsorption process in the dark using the H2O2/ZnWO4/CaO/DA system, while the second fragment was ascribed to A − P and A + P under solar light. During the simultaneous adsorption and degradation process (A + P), 85% of OTC was decolourized in 210 min. Only 53% of OTC was removed during the dark adsorption experiment. The efficiency of various catalytic systems for OTC removal followed the order of H2O2/CaO/A + P > Zr-TiO2/CaO/A − P > Zr-TiO2/CaO/DA. Furthermore, it can be noticed that OTC removal in the A + P system was higher than that for the A − P system. Our results for adsorption and degradation studies were comparable to the previous work by Shoun and co-workers [Citation34]. In this work, the A − P system had a lower removal efficiency than that of DA due to the hindering effect of adsorption on the photocatalytic process. The three processes followed the efficiency order of A + P > DA > A − P. The solar/H2O2/ZnWO4/CaO/A + P catalytic system was the most efficient photocatalytic system, and further OTC degradation studies were explored using this catalytic system. The photodegradation of OTC using the H2O2/ZnWO4/CaO catalytic system followed pseudo-first-order kinetics (R2 = 0.994) with a rate constant of 4.6 × 10−3 min−1 ((c)).
3.3 Effect of reaction parameters on the activity of the solar/H2O2/ZnWO4/CaO/A + P system
(a)–(d) depicts the influence of various reaction parameters, such as OTC concentration, catalyst loading, pH and H2O2 concentration, on the activity of the solar/H2O2/ZnWO4/CaO/A + P system. (a) displays the effect of OTC concentration on the catalytic activity of the solar/H2O2/ZnWO4/CaO system. Because the degradation rate is related to the probability of the formation of OH• radicals on the catalyst surface and the reaction of the radicals with OTC molecules, the OTC concentration was varied from 0.7 × 10−4 M to 9 × 10−4 M. With an increase in OTC concentration from 0.7 × 10−4 M to 5.0 × 10−4 M, the rate constant increased from 2 × 10−4 s−1 to 8.8 × 10−4 s−1. The increase in the rate constant was due to the higher availability of OTC molecules. The rate constant decreased with an increase in OTC concentration beyond 5.0 × 10−4 M. This decrease was because OTC acted as a filter for incident radiation and reduced the photoactive volume [Citation10,Citation34]. Second, the excessive adsorption of OTC molecules on the catalyst surface hindered the competitive adsorption of OH• ions, thus reducing the rate of OH• radical formation [Citation10,Citation34].
Fig. 6 (a–d) Effect of different reaction parameters, OTC (a), catalyst (b), H2O2 (c), pH (d) on OTC removal. Reaction conditions [OTC] = 1 × 10−4 M, catalyst dosage = 50 mg/50 ml, [H2O2] = 1 × 10−4 M, pH 5, temperature = 30 ± 0.3 °C, reaction time = 60 min and solar light intensity = 35 × 103 ± 1000 lx.
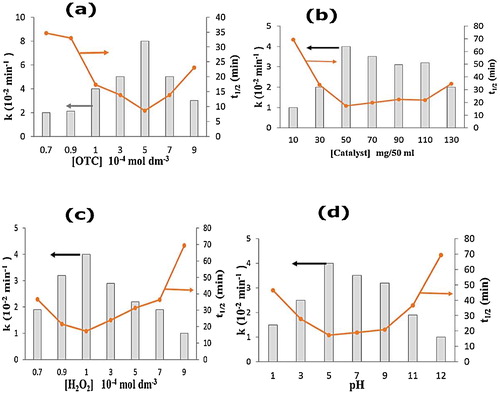
The effect of catalyst loading was investigated by varying the catalyst dose from 10 mg/50 ml to 130 mg/50 ml ((b)). The rate constant increased from 1.0 × 10−2 s−1 to 4.0 × 10−2 s−1 with an increase in catalyst loading from 10 mg/50 ml to 90 mg/50 ml. The higher rate constant was due to a higher OH• formation and a greater availability of catalyst surface for adsorption of OTC molecules. However, above the optimal concentration, excess loading reduced the photoactivity due to screening of solar light by the higher catalyst dose [Citation10,Citation34].
(c) describes the effect of H2O2 concentration on the photodegradation of OTC. The rate constant increased from 4.90 × 10−2 min−1 to 8.5 × 10−2 min−1 with an increase in the H2O2 concentration from 0.7 × 10−4 M to 1.0 × 10−4 M. This result occurred was because H2O inhibited electron–hole recombination by accepting photogenerated electrons from the conduction band of the semiconductor. OH• radicals were also produced by photolysis of H2O2 according to Eqs. Equation(7)(7)
(7) and Equation(8)
(8)
(8) [Citation10,Citation34]:
(7)
(7)
(8)
(8)
However, beyond the optimal concentration of H2O2, the rate constant decreased due to the consumption of hydroxyl radicals. Above the optimal concentration, H2O2 became a scavenger for valence band holes and OH• to form HO2• radicals, which are much weaker oxidants than OH• radicals [Citation35]. The effect of pH on OTC degradation is shown in (d). Initially, the rate constant increased from 1.0 × 10−2 min−1 to 4.0 × 10−2 min−1 with an increase in pH from 1 to 5. At pH 2, OTC was fully protonated as H3OTC+ [Citation35,Citation36]. At low pH, the surface of ZnWO4/CaO was positively charged, resulting in a lower adsorption of OTC onto the catalyst surface. However, the majority of OTC changed to the zwitterionic form (H2OTC±) at pH 5.5 [Citation35,Citation36], resulting in higher adsorption of OTC onto the catalyst surface, leading to an increased rate of OTC photodegradation. At pH values of 8.5 and 11, OTC mainly existed as HOTC̄ and OTC2−, respectively [Citation35,Citation36]. Above pH 7, the negatively charged catalyst surface caused poor adsorption of HOTC̄ and OTC2−. At lower pH values, ZnWO4 dissolved to form salts, and at a higher pH, they form zincates such as [Zn(OH)4]2− [Citation5,Citation35,Citation37].
3.4 Proposed mechanism for OTC degradation under solar/H2O2/ZnWO4/CaO/A + P
Generally, photocatalytic degradation processes occur through hydroxyl radical-mediated oxidation of organic molecules (oxidation potential – 2.8 eV). To explore the role of •OH radicals, OTC degradation experiments were performed with isopropanol. Isopropanol containing α-hydrogen is highly reactive with OH• and is has a low reactivity with the O2− species. Buxton and co-workers reported the high second order rate constant (6 × 109 M−1 s−1) of isopropanol with OH• radicals [Citation37]. The rate of OTC removal was significantly reduced due to presence of isopropanol; only 7% of OTC was removed in the presence of isopropanol due to the quenching of hydroxyl radicals. These results indicated that degradation mainly occurred via a OH• radical-assisted oxidative pathway.
The OTC degradation was confirmed by measuring the COD value at different time intervals. It was found that 8% of OTC was degraded in 60 min using the solar/H2O2/ZnWO4/A + P catalytic system, and OTC was completely degraded in 210 min (). To further explore the mineralization kinetics, the power law model was explored. The real degradation process can be represented as the combination of two parallel branches, namely, photocatalysis and photolysis (Eq. Equation(9)(9)
(9) ):
(9)
(9) where R, R1 and R2 are the net photodegradation, photocatalysis and photolysis rates, respectively. In the present study, photolysis has no significant effect on the antibiotics’ degradation. Therefore, only photocatalysis was considered as the dominant process during long-term photocatalysis for ten hours. Here, the power law model was applied to explore the kinetics of the OTC mineralization process using the ZnWO4/CaO/A + P catalytic system (Eq. Equation(10)
(10)
(10) ):
(10)
(10) where k1 is the rate constant for photocatalysis and n1 denotes the order of the reaction for photocatalysis. To determine the appropriate parameters, the differential method of data analysis was applied to Eq. Equation(10)
(10)
(10) . (a) represents the goodness of fit of the rate in Eq. Equation(10)
(10)
(10) . The reaction order (n1) was determined as 1.54. H2O2/ZnWO4/CaO catalytic systems had a rate constant of 4.57 × 10−8 (mol dm−3)−0.54 min−1. These results clearly indicated that long-term degradation did not follow simple pseudo-first-order kinetics. The degradation process tends to be slower with increased degradation time, and the overall process of mineralization is complex.
Fig. 7 (a) COD removal efficiency and (b) mineralization kinetics during degradation of OTC. Reaction conditions [OTC] = 1 × 10−4 M, catalyst dosage = 50 mg/50 ml, [H2O2] = 1 × 10−4 M, pH 5, temperature = 30 ± 0.3 °C and solar light intensity = 35 × 103 ± 1000 lx.
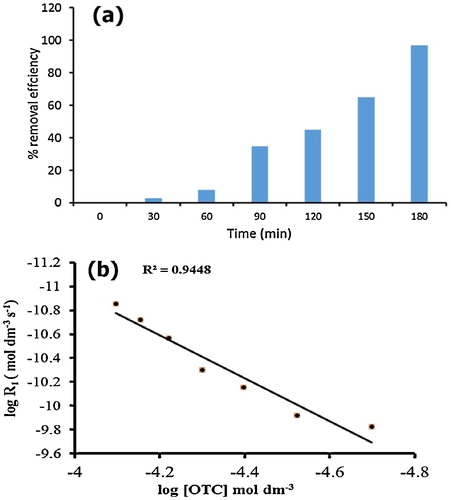
During the photocatalytic process, the illumination of ZnWO4/CaO produced conduction band electrons () and valance band holes (
) (Eqs. (9)–(16)). H2O2 mainly contributed to the degradation process by acting as an electron scavenger of valence band holes, leading to the lower electron–hole pair recombination. The active OH• and (O2•−) were also generated directly during illumination of ZnWO4/CaO [Citation4]. At pH ≈ 5, HOTC− was excited to a singlet state, which crossed to the triplet state HOTC−* via an intersystem crossing mechanism [Citation34]. The triplet state HOTC−* caused the injection of electrons in the conduction band to generate superoxide anion radicals (O2•−) [Citation34]. HOTC− also acted as an electron scavenger for conduction band electrons. Third, HOTC−* also acted as a sensitizer during the solar photocatalytic removal of OTC [Citation34]. The produced active species led to oxidative degradation of OTC [Citation32,Citation34,Citation37]. The overall mechanism was described by Eqs. (11)–(20) ():
(11)
(11)
(12)
(12)
(13)
(13)
(14)
(14)
(15)
(15)
(16)
(16)
(17)
(17)
(18)
(18)
(19)
(19)
(20)
(20)
Recycling efficiency is one of most crucial factors for an efficient photocatalytic process. The recycling efficiency of the ZnWO4/CaO composites was explored for seven successive cycles of OTC removal (). During each run, the catalyst was separated from the solution through centrifugation followed by sedimentation. The efficiency of the ZnWO4/CaO system was reduced to 70% from 85%. The study revealed that ZnWO4/CaO composites were easily recycled after seven catalytic cycles, indicating the reusability of ZnWO4/CaO composites during the photodegradation process ().
4 Conclusions
In this study, the photocatalytic activity of the H2O2/ZnWO4/CaO system was investigated for the degradation of OTC from an aqueous system. The successful formation of the ZnWO4/CaO composite was ascertained by SEM TEM, EDX XRD, FTIR and UV–vis spectral analysis. The solar/H2O2/ZnWO4/CaO catalytic system was the most efficient for OTC degradation. The photodegradation was higher during a simultaneous adsorption and photocatalytic process (A + P). The degradation of OTC was optimal at pH 5. The rate of the reaction was maximal with a 50 mg/50 ml ZnWO4/CaO loading and 5.0 × 10−4 M of OTC concentration. Under solar light, H2O2 displayed a synergistic effect in conjugation with the ZnWO4/CaO composites.
Notes
Peer review under responsibility of Taibah University.
References
- J.C.MaddenS.J.EnochM.HewittM.T.D.CroninPharmaceuticals in the environment: good practice in predicting acute ecotoxicological effectsToxicol. Lett.185200985101
- S.SchnellN.C.BolsC.BarataC.PorteSingle and combined toxicity of pharmaceuticals and personal care products (PPCPs) on the rainbow trout liver cell line RTL-W1Aquat. Toxicol.932009244252
- C.GuK.G.KarthikeyanInteraction of tetracycline with aluminum and iron hydrous oxidesEnviron. Sci. Technol.39200526602667
- J.JeongW.SongW.J.CooperJ.JungJ.GreavesDegradation of tetracycline antibiotics mechanisms and kinetic studies for advanced oxidation/reduction processesChemosphere782010533540
- B.J.RichardsonP.K.S.LamM.MartinEmerging chemicals of concern pharmaceuticals and personal care products (PPCPs) in Asia, with particular reference to Southern ChinaMar. Pollut. Bull.502005913920
- A.J.WatkinsonE.J.MurbyD.W.KolpinS.D.CostanzoThe occurrence of antibiotics in an urban watershed: from wastewater to drinking waterSci. Total Environ.407200927112723
- P.RaizadaP.SinghA.KumarB.PareS.B.JonnalgaddaZero valent iron-brick grain nanocomposite for enhanced solar-Fenton removal of malachite greenSep. Purif. Technol.1332014429437
- T.RobinsonG.McMullanR.MarchantP.NigamRemediation of dyes in textile effluent: a critical review on current treatment technologies with a proposed alternativeBioresour. Technol.772001247255
- D.BahnemannPhotocatalytic water treatment: solar energy applicationSol. Energy772004445459
- P.RaizadaS.GautamB.PriyaP.SinghPreparation and photocatalytic activity of hydroxyapatite supported BiOCl nanocomposite for oxytetracyline removalAdv. Mater. Lett.742016312318
- P.SinghP.RaizadaSolar-Fenton removal of malachite green with novel Fe0-activated carbon nanocompositeAppl. Catal. A: Gen.4762014918
- P.RaizadaP.SinghA.KumarG.SharmaB.PareS.B.JonnalagaddaP.ThakurSolar photocatalytic activity of nano-ZnO supported on activated carbon or brick grain particles: role of adsorption in dye degradationAppl. Catal. A4862014159169
- R.AsahiT.MorikawaT.OhwakiK.AokiVisible-light photocatalysis in nitrogen-doped titaniumScience2932001269271
- H.FuJ.LinL.ZhangPhotocatalytic activities of novel ZnWO4 catalyst prepared by hydrothermal processAppl. Catal. A30620065867
- D.HeL.WangD.XuJ.ZhaiD.WangInvestigation of photocatalytic activity over Bi2WO6/ZnWO4 composite under UV light and its photo induced charge transfer propertiesACS Appl. Mater. Interfaces3201131673171
- C.ZhangH.ZhangK.ZhangX.LiQ.LengC.HuPhotocatalytic activity of ZnWO4, band structure, morphology and surface modificationACS Appl. Mater. Interfaces620141442314432
- X.BaiL.WangY.ZhuVisible photocatalytic activity enhancement of ZnWO4 by graphene hybridizationACS Catal.2201227692778
- B.K.OlgaL.IsabelleV.AlexanderAlkaline-earth oxide nanoparticles obtained by aerogel methods: characterization and rational for unexpectedly high surface chemicalChem. Mater.9199724682472
- Z.H.LeeK.T.LeeS.BhatiaA.R.MohamedPost-combustion carbon dioxide capture: evolution towards utilization of nanomaterialsRenew. Sust. Energy Rev.16201225992609
- E.NeyensJ.BaeyensA review of classic Fenton’s peroxidation as an advanced oxidation techniqueJ. Hazard. Mater.9820033350
- D.RobertS.ParraC.PulgarinA.HrztonJ.V.WeberChemisorption of phenols and acids on TiO2 surfaceAppl. Surf. Sci.16720005158
- P.SinghP.RaizadaP.ThakurPreparation of BSA-ZnWO4 nanocomposites with enhanced adsorptional photocatalytic activity for methylene blue degradationInt. J. Photoenergy20132013 Article ID 726250
- B.PareS.B.JonnalagaddaH.TomarP.SinghV.W.BhagwatZnO assisted photocatalytic degradation of acridine orange in aqueous solution using visible irradiationDesalination23220088090
- B.PareP.SinghS.B.JonnalagaddaDegradation and mineralization of Victoria blue B dye in a slurry photoreactor using advanced oxidation processJ. Sci. Ind. Res.682009724729
- B.PareP.SinghS.B.JonnalagaddaVisibe light-driven photocatalytic degradation and mineralization of neutral red dye in slurry photoreactorIndian J. Chem. Technol.172010391395
- C.YuJ.C.YuSonochemical fabrication, characterization and photocatalytic properties of Ag/ZnWO4 nanorod catalystMater. Sci. Eng. B16920091622
- C.BalazsiZ.KoverE.HorvathC.NemethZ.KasztovszkyS.F.KuruncziWeber Examination of calcium-phosphate prepared from eggshellMater. Sci. Forum537–5382007105112
- D.LiR.ShiC.PanY.ZhuH.ZhaoInfluence of ZnWO4 nanorod aspect ratio on the photocatalytic activityCryst. Eng. Commun.13201146954700
- W.T.TsaiJ.M.YangC.W.LaiY.H.ChengC.C.LinC.W.YehCharacterization and adsorption properties of eggshells and eggshell membraneBioresour. Technol.972006488493
- A.RoyJ.BhattacharyaMicrowave assisted synthesis and characterization of CaO nanoparticlesInt. J. Nanosci.102011413418
- S.KomarneniR.RoyQ.H.LiMicrowave-hydrothermal synthesis of ceramic powdersMater. Res. Bull.27199213931405
- H.D.XieD.Z.ShenX.Q.WangG.Q.ShenGrowth and characterization of KBi(WO4)2 single crystalsCryst. Res. Technol.4220071822
- G.B.KumarK.SivaiahS.BuddhuduSynthesis and characterization of ZnWO4 ceramic powderCeram. Int.362010199202
- H.K.ShounS.VigneswaranH.H.NgoJ.H.HimChemical coupling of photocatalysis with flocculation and adsorption in the removal of organic matterWater Res.39200625492558
- C.YuJ.C.YuSonochemical fabrication: characterization and photocatalytic properties of Ag/ZnWO4nanorod catalystMater. Sci. Eng.16920091622
- C.ZhaoM.PelaezX.DuanH.DengK.O.SheaD.F.KassinosD.D.DionysiouRole of pH on photolytic and photocatalytic degradation of antibiotic oxytetracycline in aqueous solution under visible/solar light: kinetics and mechanism studiesAppl. Catal. B134–13520138392
- G.V.BuxtonC.GreenstockW.P.HellmanA.B.RossCritical-review of rate constants for reactions of hydrated electrons, hydrogen-atoms and hydroxyl radicals (•OH/•O−) in aqueous-solutionJ. Phys. Chem. Ref. Data171988513886