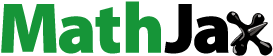
Abstract
A hybrid of chitosan and 3-amino-1,2,4-triazole was prepared using the semi-wet method, which allows for the adsorption of the triazole molecules on the chitosan surface. Moreover, an easy method for applying this hybrid to cotton fabric was established. The tensional strength, uniformity and compatibility of the hybrid components in forming of a strong film were studied using different variables, including the chitosan 3-amino-1,2,4-triazole ratio, fixation temperature and time. The loading of the hybrid onto the fabric in the absence and presence of cross linker (butane tetra carboxylic acid) was also studied. The best conditions for preparing the hybrid was a 1:4 molar ratio of chitosan to 3-amino-1,2,4-triazole at 60 °C for 240 min, and those for the application of the hybrid to cotton fabric were a 1:1 molar ratio at 150 °C with a 5 min curing time. Both hybrid and treated cotton fabrics were characterized using FTIR, SEM, TGA, and DSC as well as the nitrogen content and tensional strength of the treated cotton. Finally, the antibacterial activities of the treated cotton fabric display 100% activity and excellent effects against gram-positive bacteria, Staphylococcus aureus and gram-negative Escherichia coli.
1 Introduction
Chitin and chitosan have amazing properties, including biodegradability, biocompatibility and non-toxicity [Citation1]. Improvements in the chitosan properties have been made to prepare for the utilitarian subordinates of chitosan via the union response, ionic cooperation, and dissolution in organic solvents. Their dissolvability in different media, viscosity, electrolytic behaviour, oxysalt development and formation of film metal chelation and optical and structural proprieties have previously been reported [Citation2–Citation5].
Table 3 Effect of chitosan 3-amino-1,2,4-triazole hybrid concentration on technical properties of fabrics, fixation at 150 °C for 5 min.
Table 4 Effect of chitosan 3-amino-1,2,4-triazole hybrid concentration in presence of BTCA on technical properties of fabrics fixation at 150 °C for 5 min.
Table 5 Effect of curing temperature on the magnitude of interaction of chitosan 3-amino-1,2,4-triazole hybrid and cotton; Curing time 5 min; molar ratio 1:1.
Table 6 Effect of curing time on the magnitude of interaction of chitosan 3-amino-1,2,4-triazole hybrid and cotton.
The solubility of chitosan is best in aqueous acidic solutions of some acids, while N-alkylidination [Citation6,Citation7] and N-acylation [Citation8,Citation9] have been reported to improve water solubility and swelling [Citation2,Citation10]. It is difficult to develop solubility in common organic solvents by these methods.
Chitosan is widely utilized as a biomedical material. It has been reported [Citation11–Citation15] that chitosan is used in several fields, such as pharmaceutical and biomedical designing, paper creation, material completion, photographic items, metal chelation, water treatment, fibre formation, green synthesis and antimicrobial applications.
Cotton is the most imperative of all strands. Cotton fibres have good moisture absorption and wicking properties. Additionally, they have great quality and are considered to produce agreeable material fabrics. Because cotton is a great medium for the growth of organisms, it requires intensive improvement to become antimicrobial. Hence, significant efforts are being made around the world to bestow biotical movement to cotton materials and make them antimicrobial [Citation16].
Heterocyclic nitrogen has been attracting interest over the last decade because of various applications, particularly chemotherapy [Citation17]. One of the important compounds in agricultural, industrial and biological activities is 1,2,4-triazole and its derivatives [Citation18]. Triazoles are known as antimicrobial, antidepressant [Citation19], anticancer [Citation20], anti-inflammatory [Citation21,Citation22], antimalarial [Citation23] agents. Moreover, the majority of triazoles are reported [Citation24] as environmentally friendly materials. As a result, incorporation of triazoles into polymeric systems would allow for improvement of textiles using the conventional pad-dry-cure method. This trend is highlighted in the development of smart and intelligent textiles [Citation25].
The aim of this work is to establish an effective system for preparing chitosan 3-amino-1,2,4-triazole hybrid as well as its application to cotton fabric.
2 Materials and methods
2.1 General
Scoured and bleached plain weave cotton fabric (230 g/m2) was kindly supplied by Misr Company for Spinning and Weaving, Mehalla El Kubra, Egypt. Chitosan with a medium molecular weight, 75–85% deacetylation, and viscosity of 800–200 cps a d 3-amino-1,2,4-triazole were supplied by Aldrich Chemical (Germany).
FTIR spectroscopy was conducted using an FT-IR-FT-Raman instrument, model: Nexus 670 (Nicollet-Madison-WI-USA). The tensile strength of the samples was determined by the ASTM Test Method D5035. A Q-Test 1/5 Tensile Tester was used. The crease recovery angle (CRA) of the treated samples was determined according to the AATCC standard test Method 66-1996. SEM was studied using a scanning electron probe micro analyser (type quanta EFG250-philips) – Holland. The micrographs were taken at two magnifications, 1000 and 2500, using a 30 kV accelerating voltage. Thermo gravimetric analysis (TGA) and differential thermal analysis (DTA) were performed using an SDTQ600 TA company, model Pyris. The runs were performed over a temperature range of 50–600 °C at a heating rate of 10 °C/min under a continuous N2 flow of 100 mL/min.
All antibacterial activity tests were performed three times to ensure reproducibility. The activity towards bacteria of fabric samples was investigated against Escherichia coli and Staphylococcus aureus, (ATCC 1533) bacteria using the method of disk diffusion. A mixture of nutrient broth and agar in 1 L of distilled water at pH 7.2 was collected in empty autoclaved Petri plates. The medium was poured into the Petri plates and cooled under laminar air flow E. coli bacteria (105 colony-forming units) were inoculated on plates; then, fabric samples were placed on the agar plates. The plates were incubated at 37 °C for 24 h and examined to determine whether a zone of inhibition was produced around the samples.
2.2 Methods
2.2.1 Preparation of chitosan 3-amino-1,2,4-triazole hybrid
Based on the preliminary laboratory trials, the modification of chitosan was performed in two steps, chitosan activation and mixing of 3-amino-1,2,4-triazole. In the first step, chitosan activation, 1 g of chitosan was swelled in 5 mL of 20% aqueous acetic acid for 10 min. In the second step, different molar ratios of chitosan and 3-amino-1,2,4-triazole (1:1, 1:2, 1:3, and 1:4) were mixed before heating at 60 °C for different times (0.5–4 h). The hybrid product was washed by isopropyl alcohol and left to dry under ambient conditions.
2.2.2 Application of chitosan 3-amino-1,2,4-triazole hybrid to cotton fabric
2.2.2.1 In the absence of the cross linker
Before any treatment, cotton was washed in an aqueous solution with 2 g/L of nonionic surfactant Adrasil HP and liquor at a ratio of 1:25 for 45 min at 60 °C. Then, the samples were rinsed twice with distilled water and dried overnight at room temperature.
A water-soluble chitosan hybrid was prepared at different concentrations (0.4%, 0.8%, 1%, 2%, and 4%). This mixture was stirred to produce a uniform solution at room temperature for 30 min. Cotton swatches were soaked in the solution for 15 min and then padded through a laboratory wringer. Immediately after padding, the samples were dried at 80 °C for 5 min, and three curing temperatures, 130, 150 and 160 °C, and curing times of 3, 5, and 6 min were used to produce samples. After curing, the fabric samples were rinsed with warm water for 15 min and dried at room temperature.
2.2.2.2 In the presence of butane-1,2,3,4-tetracarboxylic acid (BTCA)
A mixture of hybrid and 5% by weight of BTCA cross linkers and its compatible catalyst sodium hypophosphite (2 g in 50 mL water) was prepared. This mixture was stirred to produce a uniform solution at room temperature for 30 min. Cotton swatches were soaked in the solution for 30 min and then padded through a laboratory wringer. Immediately after padding, the samples were dried at 80 °C for 5 min, and three curing temperatures at 130, 150 and 160 °C with curing times of 3, 5, and 6 min were used to produce samples. After curing, the fabric samples were rinsed with warm water for 15 min and dried at room temperature
2.2.3 Determination of the nitrogen content
Measurements were performed according to Kjeldahl method [Citation26]. Chitosan 3-amino-1,2,4-triazole hybrid (100 mg) was placed into a Kjeldahl flask, and 5 g of potassium sulfate was added; additionally, 0.5 g of cupric sulfate and 20 mL of sulfuric acid were added. The mixture was heated gently until effervescence almost stopped, and the temperature was raised to boil, which continued until it was a clear solution (approximately 2 h). After cooling, 150 mL of water was added, and 85 mL of sodium hydroxide was added dropwise to the gently heated solution. The evolved ammonia was trapped by distillation through 25 mL of 0.005 mol/L sulfuric acid. The excess unreacted sulfuric acid was titrated against 0.1 mol/L sodium hydroxide solution.
3 Results and discussion
3.1 Formation of chitosan 3-amino-1,2,4-triazole hybrid
The formation of chitosan and 3-amino-1,2,4 triazole hybrid was performed under semi-dry conditions (5 mL of 20% of acetic acid) to form a homogeneous, compatible and uniform mixture. This condition allows for maximum adsorption of the amino triazole molecule on the surface of a chitosan backbone.
3.2 Factors affecting the preparation of chitosan 3-amino-1,2,4-triazole hybrid
3.2.1 Molar ratio of chitosan:aminotriazole
shows the effect of chitosan:aminotriazole molar ratios at 600 °C for 2 h on add-on % and nitrogen % of the hybrid. It is clear that the add-on % and nitrogen % increase significantly by increasing the molar ratio, which indicates an increase in the aminotriazole amount due to its penetration and adsorption of the chitosan moiety.
Table 1 Preparation of chitosan 3-amino-1,2,4-triazole hybrid at different molar ratios at 60 °C for 2 h.
3.2.2 Mixing time
shows a total increase in the weight of the hybrid due to longer mixing of aminotriazole with chitosan. There is a significant enhancement in the add-on % and nitrogen % upon increasing of the duration of mixing from 30 to 60 min. Moreover, prolongation of the mixing time up to 240 min is accompanied by sharp increases in the add-on % and nitrogen % of 54.1 and 39.1%, respectively. A long mixing time provides a better opportunity for the hybrid components to closely interact through penetration, adsorption and bulk surface physical modification.
Table 2 Effect of mixing duration on add-on (%) and nitrogen (%) of chitosan 3-amino-1,2,4-triazole hybrid.
3.3 Factors affecting the treatment of cotton fabric with chitosan 3-amino-1,2,4-triazole hybrid
3.3.1 Chitosan 3-amino-1,2,4-triazole concentration
and illustrate the impact of the concentration of the chitosan 3-amino-1,2,4-triazole hybrid on the add-on % and nitrogen % and the strength properties of cotton fabrics in the absence and presence of a cross linking agent, respectively.
It is clear that the cotton fabrics treated with different concentrations of the chitosan 3-amino-1,2,4-triazole hybrid in the presence of BTCA cross linker create fabrics with higher add-on % and nitrogen %, resulting in a lower tensile strength and negligible change in the elongation at break. This suggests that the diffusion of hard particles of the hybrid into the micro fibre structure of cotton and the presence of BTCA improve the crease recovery angle, which lowers the tensile strength.
3.3.2 Curing temperature and time
and show the effect of temperature and time of curing of cotton fabrics at a molar concentration of 1:1 on the add-on % and nitrogen % as well as the tensile strength and elongation at fabric breakage. Evidently, both the temperature and time of curing improve the fabric performance, while the maximum interaction of the hybrid with cotton fabric was achieved at a curing temperature of 150 °C for 5 min.
3.4 Analysis
3.4.1 FTIR-analysis of chitosan 3-amino-1,2,4-triazole and treated cotton fabrics
The FTIR spectrum of chitosan shows peaks assigned to the polysaccharide structure of chitosan. The appearance of a vibrational peak at 3500–3000 cm−1 is attributed to the stretching vibration of the OH and NH groups, while the peak for the C–H bond is observed at 2930–2875 cm−1. Bending vibrations of the CH group are also visible in the range of 1380–1460 cm−1[Citation27]. The vibrations of carbonyl bonds (C=O) of the amide group CONHR appeared in the range of 1680–1480 cm−1, and the vibration of the amine group NH2 is [Citation28]. The spectra in the range 1160–1000 cm−1 have been attributed to asymmetric vibrations of CO in the oxygen bridge [Citation29,Citation30]. The FTIR spectrum of 3-amino-1,2,4-triazole is also very useful in characterizing the triazole ring, with an absorption in the region of 1526 cm−1 attributed to N=N, while the peak at 1614 cm−1 is due to the C=N moiety. The chitosan 3-amino-1,2,4-triazole hybrid that has been characterized by the appearance of NH2 bands in the region of 3415 cm−1 and peaks at 1110 and 1035 cm−1 that indicate the presence of C–O–C group. There was also a peak at 2919 cm−1 for (C–H) sp3 stretching and at 2782 cm−1 for (N–C–H) sp2 stretching vibration.
The FTIR spectroscopy of bleached cotton shows a broad, strong peak at 3400 cm−1 and a medium peak at 612 cm−1 that are attributed to stretching and bending of alcoholic –OH, respectively. A strong peak at 3252 cm−1 of the cotton coated with hybrid indicates the presence of an NH group. A weak peak at 2430 cm−1 is attributed to the stretching vibration of the amino-oxy group. The FTIR of cotton coated with hybrid in the presence of cross linker confirms the presence of a new band at 3408 cm−1 of the amino group of chitosan 3-amino-1,2,4-triazole hybrid. Moreover, the appearance of the C–N group at 1362 cm−1 and 1335 cm−1 indicates the fixation of chitosan 3-amino-1,2,4-triazole hybrid on the cotton fabric (Figs. S1 and S2, supplementary data).
The FTIR spectroscopy of bleached cotton shows a broad, strong peak at 3400 cm−1 and a medium peak at 612 cm−1 that are attributed to stretching and bending of alcoholic –OH, respectively. A strong peak at 3252 cm−1 of the cotton coated with hybrid indicates the presence of an NH group. A weak peak at 2430 cm−1 is attributed to the stretching vibration of the amino-oxy group. The FTIR of cotton coated with hybrid in the presence of cross linker confirms the presence of a new band at 3408 cm−1 of the amino group of chitosan 3-amino-1,2,4-triazole hybrid. Moreover, the appearance of the C–N group at 1362 cm−1 and 1335 cm−1 indicates the fixation of chitosan 3-amino-1,2,4-triazole hybrid on the cotton fabric (Figs. S1 and S2, supplementary data).
3.4.2 Scanning electron microscopy (SEM)
a–d shows the differences in the particle shapes of the blank chitosan a and chitosan aminotriazole b. c and d shows the SEM of the blank cotton and cotton treated with the hybrid, respectively. The pictures show that cotton fibres become more closed and compact than the cotton blank.
3.4.3 Thermo-gravimetric analysis (TGA)
The TGA of chitosan and chitosan 3-amino-1,2,4-triazole hybrid shows three different zones. The first zone has a slight loss in weight due to water evaporation. Then, the break in each thermogram indicates that the decomposition process involves rapid weight loss. Additionally, the end of this break, a slight curvature, is due to the evaporation of some volatile compounds. Finally, the decomposition rate decreases gradually to a constant weight, representing carbonization.
The TGA of chitosan shows two consecutive weight loss steps. The first weight loss was approximately 16.48 wt% at 55–191 °C, corresponding to moisture loss. In the third, the weight loss in the range of 327–840 °C is approximately 52.18 wt%, which is the thermal decomposition of glucosamine residues.
Similarly, modified chitosan shows three consecutive weight loss steps. The first weight loss step was approximately 13.22 wt% at 55–200 °C. The second weight loss step was approximately 48.56 wt% in the range of 230–327 °C due to scission of the ether linkage in the chitosan backbone. The third stage in the range of 327–840 °C had 18.12% weight loss (Fig. S3, supplementary data).
Similarly, modified chitosan shows three consecutive weight loss steps. The first weight loss step was approximately 13.22 wt% at 55–200 °C. The second weight loss step was approximately 48.56 wt% in the range of 230–327 °C due to scission of the ether linkage in the chitosan backbone. The third stage in the range of 327–840 °C had 18.12% weight loss (Fig. S3, supplementary data).
The main observation on the chitosan 3-amino-1,2,4-triazole hybrid TGA is a lower decomposition temperature compared to pure chitosan, which indicates the heat resistance of the modified chitosan.
The TGA curves of the treated and untreated cotton fabric samples show that the chitosan 3-amino-1,2,4-triazole hybrid changes the decomposition temperature from 330 °C for untreated cotton fabric to 365 °C for treated fabric. This reveals higher weight loss (86.63%) for the untreated compared to the treated sample (74.88%) (Fig. S4, supplementary data).
The TGA curves of the treated and untreated cotton fabric samples show that the chitosan 3-amino-1,2,4-triazole hybrid changes the decomposition temperature from 330 °C for untreated cotton fabric to 365 °C for treated fabric. This reveals higher weight loss (86.63%) for the untreated compared to the treated sample (74.88%) (Fig. S4, supplementary data).
3.4.4 Differential scanning calorimetry (DSC)
The DSC curves of chitosan and chitosan 3-amino-1,2,4-triazole hybrid have an endothermic peak at 290 °C, which is attributed to the loss of water content in the polymer. Additionally, the exothermic peak appears at 306 °C, corresponding to the decomposition of the polymer chain [Citation31] (Fig. S5, supplementary data).
The DSC curves of chitosan and chitosan 3-amino-1,2,4-triazole hybrid have an endothermic peak at 290 °C, which is attributed to the loss of water content in the polymer. Additionally, the exothermic peak appears at 306 °C, corresponding to the decomposition of the polymer chain [Citation31] (Fig. S5, supplementary data).
The DSC curve of 3-amino-1,2,4-triazole exhibits two endothermic peaks. These endothermic peaks show a maximum at 153 °C and shoulder at 234 °C (Fig. S6, supplementary data).
The DSC curve of 3-amino-1,2,4-triazole exhibits two endothermic peaks. These endothermic peaks show a maximum at 153 °C and shoulder at 234 °C (Fig. S6, supplementary data).
3.4.5 Antibacterial study
shows the development of antibacterial cotton textile by coating it with chitosan 3-amino-1,2,4-triazole hybrid at different concentrations. The antibacterial activities of the treated cotton materials display 100% activity and have excellent antibacterial effects against gram-positive bacteria, S. aureus and gram-negative E. coli.
4 Conclusions
Innovative functionalization of chitosan is successfully achieved via interaction between chitosan and 3-amino-1,2,4-triazole in semidry conditions, yielding a chitosan 3-amino-1,2,4-triazole hybrid. Factors affecting the interaction, including the molar ratio, reaction time and temperature, were studied. The efficiency of triazole penetration was determined by measuring the nitrogen content of the modified chitosan. Application of chitosan 3-amino-1,2,4-triazole hybrid to cotton fabric improved its properties, such as elongation at break, thermal stability and antibacterial activity. The best conditions for preparing a chitosan 3-amino-1,2,4-triazole hybrid was the 1:4 molar ratio at 60 °C for 240 min, while those for the application of the hybrid to cotton fabric were a 1:1 molar ratio at 150 °C with a curing time 5 min. As a result of coating the cotton fabric with chitosan 3-amino-1,2,4-triazole hybrid, there was significant improvement in the fabric performance, such as excellent antibacterial activity and thermal stability, with little decrease in the tensile strength. The cotton fabric has become a 100% antibacterial textile through treatment with the hybrid in presence of a cross linker.
Authors’ contribution
All authors equally contributed to all sections of this paper.
Supplementary data
Download MS Word (2.4 MB)Acknowledgements
The authors gratefully acknowledge Deanship of Scientific Research of Taibah University for the research funding (1434/4626) that supported this work.
Notes
Peer review under responsibility of Taibah University.
References
- P.K.DuttaJ.DuttaV.TripathiChitin and chitosan: chemistry, properties and applicationsJ. Sci. Ind. Res.6320042031
- M.RaniA.AgarwalY.S.NegiReview: chitosan based hydrogel polymeric beads – as drug delivery systemBioResources5201027652807
- F.CarosioC.Negrell-GuiraoJ.AlongiG.DavidG.CaminoAll-polymer layer by layer coating as efficient solution to polyurethane foam flame retardancyEur. Polym. J.70201594103
- G.LauferC.KirklandA.A.CainJ.C.GrunlanClay-chitosan nanobrick walls: completely renewable gas barrier and flame-retardant nanocoatingsACS Appl. Mater. Interfaces4201216431649
- F.CarosioA.Di BlasioF.CutticaJ.AlongiG.MalucelliSelf-assembled hybrid nanoarchitectures deposited on poly(urethane) foams capable of chemically adapting to extreme heatRSC Adv.420141667416680
- P.K.DuttaM.RavikumarJ.DuttaChitin and chitosan for versatile applicationsJ. Macromol. Sci. C: Polym. Rev.422002307354
- A.Abou-OkeilA.El-ShafieA.HebeishChitosan phosphate induced better thermal characteristics to cotton fabricJ. Appl. Polym. Sci.103200720212026
- A.El-ShafeiM.ElShemyA.Abou-OkeilEco-friendly finishing agent for cotton fabrics to improve flame retardant and antibacterial propertiesCarbohydr. Polym.11820158390
- E.WeilR.EngelR.EngelHandbook of Organophosphorus Chemistry1992 (Chapter 14)
- H.-S.LeeM.Q.YeeY.Y.EckmannN.J.HickokD.M.EckmannR.J.CompostoReversible swelling of chitosan and quaternary ammonium modified chitosan brush layers: effects of pH and counter anion size and functionalityJ. Mater. Chem.2220121960519616
- R.JayakumarN.NweS.TokuraH.TamuraSulfated chitin and chitosan as novel biomaterialsInt. J. Biol. Macromol.402007175181
- Y.WangJ.ZhouL.LiuC.HuangD.ZhouL.FuCharacterization and toxicology evaluation of chitosan nanoparticles on the embryonic development of zebrafish, Danio RerioCarbohydr. Polym.1412016
- N.M.ZainA.StapleyG.ShamaGreen synthesis of silver and copper nanoparticles using ascorbic acid and chitosan for antimicrobial applicationsCarbohydr. Polym.1122014195202
- R.-C.ChienM.-T.YenJ.-L.MauAntimicrobial and antitumor activities of chitosan from shiitake stipes, compared to commercial chitosan from crab shellsCarbohydr. Polym.1382016259264
- M.HosseinnejadS.M.JafariEvaluation of different factors affecting antimicrobial properties of chitosanInt. J. Biol. Macromol.852016467475
- A.El ShafeiS.ShaarawyA.HebeishApplication of reactive cyclodextrin poly butyl acrylate preformed polymers containing nano-ZnO to cotton fabrics and their impact on fabric performanceCarbohydr. Polym.792010852857
- J.do Couto AlmeidaI.M.MarzanoM.PivattoN.P.LopesA.M.D.C.FerreiraF.Pavanet al.Synthesis, cytotoxic and antitubercular activities of copper (II) complexes with heterocyclic bases and 3-hydroxypicolinic acidInorg. Chim. Acta44620168792
- C.W.TornøeC.ChristensenM.MeldalPeptidotriazoles on solid phase:(1, 2, 3)-triazoles by regiospecific copper (I)-catalyzed 1, 3-dipolar cycloadditions of terminal alkynes to azidesJ. Organ. Chem.67200230573064
- F.ReckF.ZhouM.GirardotG.KernC.J.EyermannN.J.Haleset al.Identification of 4-substituted 1, 2, 3-triazoles as novel oxazolidinone antibacterial agents with reduced activity against monoamine oxidase AJ. Med. Chem.482005499506
- W.-T.LiW.-H.WuC.-H.TangR.TaiS.-T.ChenOne-pot tandem copper-catalyzed library synthesis of 1-thiazolyl-1, 2, 3-triazoles as anticancer agentsACS Comb. Sci.1320107278
- D.SarigolA.Uzgoren-BaranB.C.TelE.I.SomuncuogluI.KazkayasiK.Ozadali-Sariet al.Novel thiazolo (3, 2-b)-1, 2, 4-triazoles derived from naproxen with analgesic/anti-inflammatory properties: synthesis, biological evaluation and molecular modeling studiesBioorg. Med. Chem.23201525182528
- S.NarsimhaN.S.KumarB.K.SwamyN.V.ReddyS.A.HussainM.S.RaoIndole-2-carboxylic acid derived mono and bis 1, 4-disubstituted 1, 2, 3-triazoles: synthesis, characterization and evaluation of anticancer, antibacterial, and DNA-cleavage activitiesBioorg. Med. Chem. Lett.262016
- S.ManoharS.I.KhanD.S.RawatSynthesis of 4-aminoquinoline-1, 2, 3-triazole and 4-aminoquinoline-1, 2, 3-triazole-1, 3, 5-triazine hybrids as potential antimalarial agentsChem. Biol. Drug Des.782011124136
- B.D.MertM.E.MertG.KardaşB.YazıcıExperimental and theoretical investigation of 3-amino-1, 2, 4-triazole-5-thiol as a corrosion inhibitor for carbon steel in HCl mediumCorros. Sci.53201142654272
- A.PrasadA.N.RaoT.RamalinganP.SatturSynthesis and biological activity 4-amino-3-aryloxyalkyl-5-mercapto-1, 2, 4-triazolesIndian Drugs251988301304
- A.I.VogelElementary Practical Organic Chemistry2nd ed.1975LongmanLondon652
- J.F.ManoD.KoniarovaR.L.ReisJ. Mater. Sci.: Mater. Med.142003127135
- R.H.MarchessaultF.RavenelleX.X.ZhuPolysaccharides for Drug Delivery And Pharmaceutical Applications2006American Chemical Society
- M.L.DuarteM.C.FerreiraM.R.MarvaoJ.RochaInt. J. Biol. Macromol.31200218
- Y.XuK.KimM.HannaD.NagInd. Crops Prod.212005185192
- L.S.GuinesiE.T.G.CavalheiroThe use of DSC curves to determine the acetylation degree of chitin/chitosan samplesThermochim. Acta4442006128133
Appendix A
Supplementary data
Supplementary data associated with this article can be found, in the online version, at doi:doi:10.1016/j.jtusci.2016.08.008.
Appendix A
Supplementary data
The following are the supplementary data to this article: