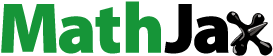
Abstract
New acyclonucleoside analogs tethered by a 1,2,4-triazole scaffold were synthesized through the condensation of 4-amino-5-(2-phenyleth-1-yl)-2,4-dihydro-3H-1,2,4-triazole-3-thione (2) with benzaldehyde followed by the alkylation of the resulting Schiff base (3)with 2-bromoethanol, 3-chloropropanol and/or 3-chloropropan-1,2-diol. Voltammetric studies were carried out for the analysis of 1 × 10−6 mol L−1 of the newly synthesized acyclonucleoside analogs (4–6) using square wave-adsorptive stripping voltammetry (SW-AdSV). The sharp voltammetric peak and high reduction current were recorded using a Britton–Robinson B–R pH 10 buffer at Ep = −1250 mV on the hanging mercury drop surface (HMDE) and Ag/AgCl reference electrode. Several experimental conditions were studied, such as the supporting electrolytes, the pH, and the accumulation time, as well as the potential, the scan rate, the frequency and the step potential for 4-benzylideneamino-5-(2-phenyleth-1-yl)-3-[(2,3-dihydroxyprop-1-yl)thio]-1,2,4-triazole (6). The analytical performance of the voltammetric technique was investigated through the analysis of the calibration curve, the detection limit, the recovery and the stability. The voltammetric analytical applications were evaluated by the recovery of compound (6) in the urine and plasma samples. The HPLC technique was also applied for the separation of compound (6) from interference using a C-18 (5 μm) column with UV detection at 254 nm.
1 Introduction
During the last several years, the 1,2,4-triazole nucleus has emerged as one of the most attractive azoles in medicinal chemistry, exhibiting anticancer, anti-inflammatory, analgesic and anticonvulsant activities [Citation1–Citation4]. Moreover, the incorporation of an azomethine Schiff base linkage into a 1,2,4-triazole scaffold resulted in the formation of several therapeutically active compounds, which may significantly potentiate antimicrobial activities [Citation5–Citation7]. On the other hand, heterocycles carrying hydroxylated acyclic side chains such as acyclovir (ACV), ganciclovir (GCV), penciclovir (PCV), and (S)-9-(2,3-dihydroxypropyl) adenine are important classes of acyclonucleosides endowed with significant antiviral activity [Citation8–Citation11].
Consequently, the synthesis of heterocycles incorporating open chain carbohydrate residues was the subject of extensive research articles that focused on modification of the heterocyclic base and/or the acyclic side chain [Citation12–Citation15].
Recently, considerable attention has been devoted to the application of electrochemical analysis in analytical chemistry owing to these methods’ high sensitivity, accuracy and low cost instrumentations. The most common methods are voltammetry techniques, which have been extensively used in the pharmaceutical industry, the metal industry, complexation chemistry, biological samples and environmental applications. The most widespread voltammetric techniques are polarography, square wave voltammetry, cyclic voltammetry and stripping techniques [Citation16–Citation22]. On the other hand, HPLC has gained much attention as a powerful, accurate and regularly used analytical technique in environmental, industrial and biological analyses [Citation23–Citation30].
During the continuation of our search on the synthesis of acyclonucleoside analogs [Citation31–Citation33], a new series of acyclonucleoside analogs bearing a 1,2,4-triazole Schiff base moiety were synthesized with the intention to explore their voltammetric behavior using square wave-adsorptive stripping voltammetry.
2 Results and discussion
2.1 Chemistry
The synthetic methodology employed for the preparation of the precursor 4-amino-5-(2-phenyleth-1-yl)-2,4-dihydro-3H-1,2,4-triazole-3-thione (2) required multistep reactions as outlined in . Thus, the treatment of 3-phenylpropanoic acid hydrazide with carbon disulfide in ethanolic potassium hydroxide solution, at 0 °C for 16 h, afforded the corresponding potassium dithiocarbazate salt, which upon refluxing with hydrazine hydrate for 4 h yielded the corresponding aminotriazole (2) in 90% yield. The synthesized triazole was identical to that previously prepared in 63% yield by the fusion of 3-phenylethanoic acid with thiocarbohydrazide for 20–25 min [Citation34]. The multistep synthesis strategy adopted in the present work has been widely reported in literature for the preparation of several 4-amino-1,2,4-triazole-2-thione derivatives in higher yield [Citation35,Citation36], although the strategy required a longer time and several steps compared to the one pot synthesis of similar derivatives via the reaction of the appropriate carboxylic acid with thiocarbohydrazide [Citation37,Citation38].
The formation of triazole (2) was confirmed by spectroscopic data. Its IR spectrum revealed the presence of the characteristic absorption bands for the triazole ring at 3275–3348 cm−1 (N–H, NH2), 1605 cm−1 (C=N) and 1295 cm−1 (C=S). In the 1H NMR spectrum, the appearance of two characteristic singlets at δH 5.67 and 14.33 ppm, corresponding to NH2 and NH protons, confirmed the predominance of the thione form.
Conversely, thermal condensation of aminotriazole (2) with benzaldehyde in refluxing ethanol in the presence of a catalytic amount of hydrochloric acid provided the corresponding Schiff base (3) in 92% yield ().
The 1H NMR spectrum of Schiff base (3) confirmed the absence of the singlet corresponding to the NH2 group and the presence of a distinctive singlet at δH 9.74 attributed to the azomethine proton. The 13C NMR analysis also supported the formation of Schiff base (3) in the thione form, through the appearance of two characteristic signals at δC 163.04 and 165.48 ppm belonging to the C=N and C=S groups, respectively.
Base catalyzed alkylation (NaOEt) of Schiff base (3) with bromoethanol, chlorobutanol and/or 3-chloropropane-1,2-diol, in refluxing ethanol for 6 h, afforded the desired acyclonucleoside analogs (4–6) in 84–88% yield. The structural assignments of the acyclonucleoside analogs (4–6) were established on the basis of their IR, 1H NMR and 13C NMR spectral data. In the 1H NMR spectra of compounds (4) and (5), the SCH2 protons appeared as triplets at δH 3.24–3.32 ppm and integrated for two protons. The OH proton attached to the ethyl side-chain in compound (4) appeared as a triplet at δH 5.04 ppm (J = 4.4 Hz) while the OH proton bonded to the butyl side chain in compound (5) resonated as a broad singlet at δH 4.62 ppm. In addition, the 1H NMR spectrum of compound (6) showed two exchangeable proton signals at δH 4.70 and 4.99 ppm integrating for two and are characteristic of the two hydroxyl groups of the glycerol side chain.
2.2 Voltammetric results
A solution of 1 × 10−6 mol L−1 of 4-benzylideneamino-5-(2-phenyleth-1-yl)-3-[(2,3-dihydroxyprop-1-yl)thio]-1,2,4-triazole (6) was analyzed by SW-AdSV using different analytical parameters, yielded a cathodic voltammogram at Ep = −1250 mV in B–R buffer pH 10, 60 s accumulation time, −0.6 V accumulation potential, 300 mV s−1 scan rate, 10 Hz, 250 mV step potential, 0.6 mm2 drop size and 2600 rpm stirrer rate. The resulted cathodic current was suggested by electrochemical reduction of the exocyclic azomethine group (–N=CH–) as illustrated in .
Scheme 3 Proposed reduction mechanism of 4-benzylideneamino-5-(2-phenyleth-1-yl)-3-[(2,3-dihydroxyprop-1-yl)thio]-1,2,4-triazole (6).
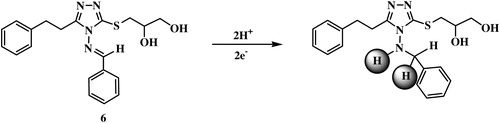
The voltammetric studies were conducted on a 1 × 10−6 mol L−1 sample of 4-benzylideneamino-5-(2-phenyleth-1-yl)-3-[(2,3-dihydroxyprop-1-yl)thio]-1,2,4-triazole (6) using some analytical parameters as summarized in and illustrated in –. These parameters were studied to achieve a high current (sensitivity) and a sharp voltammetric peak. In general, the voltammetric current reached the highest value by use B–R supporting electrolyte, pH 10, 60 s acc time, −0.6 V acc potential, 300 mV s−1 scan rate, 10 Hz frequency, 250 mV step potential, 0.6 mm2 drop size and 2600 rpm stirrer.
Fig. 1 Effect of pH on electrochemical signal of 1 × 10−6 mol L−1 of compound 6 at B–R over the pH range 7.5–11.
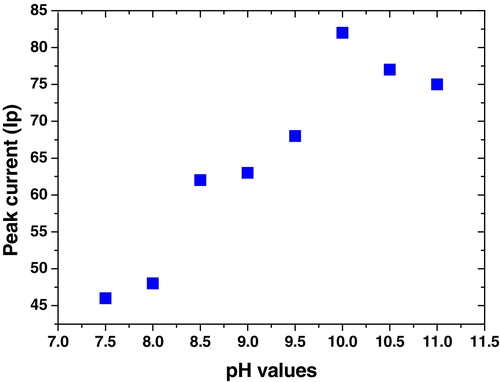
Fig. 2 Effect of acc potential (Eacc) on voltammetric signal of 1 × 10−6 mol L−1 of compound 6 at B–R and pH 10 over the Eacc range −0.8 to +0.4 V.
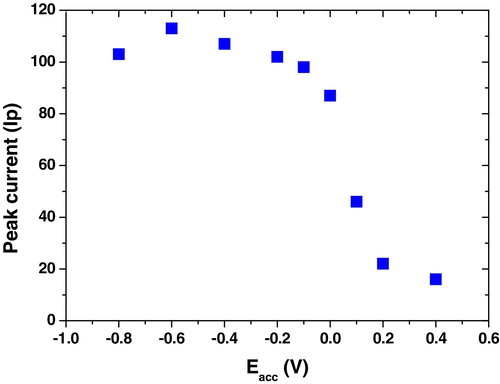
Fig. 3 Effect of frequency (Hz) on voltammetric signal of 1 × 10−6 mol L−1 of compound 6 at B–R, pH 10, −0.6 V Eacc, 60 s tacc and 300 mV s−1 over the range 5–30 Hz.
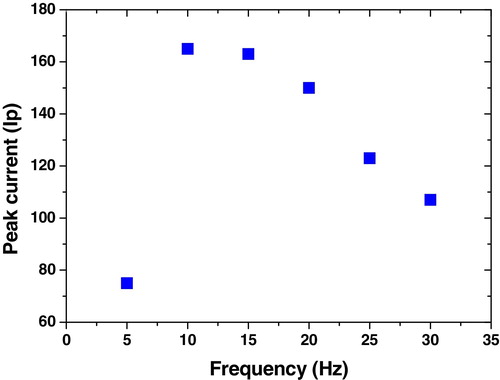
Fig. 4 Effect of step potential (mV) on voltammetric signal of 1 × 10−6 mol L−1 of compound 6 at B–R, pH 10, −0.6 V Eacc, 60 s tacc, 300 mV s−1 and 10 Hz over the range 10–350 mV.
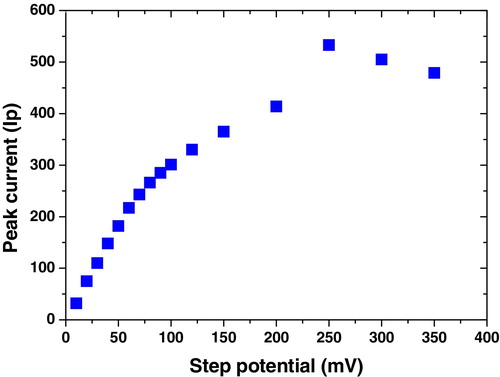
Table 1 Results of the optimum analytical conditions of compound 6 by SW-AdSV.
2.2.1 Voltammograms under optimum conditions
The analytical studies revealed that the selected parameters recorded high reduction current for all synthesized compounds as summarized in , and the square wave-adsorptive stripping, cyclic and multi-cyclic voltammograms were illustrated in – over the potential range from −0.5 to −1.5 V. Cyclic voltammetry confirmed that the analyzed compound did not show any irreversible characteristic nature. Multi-cyclic voltammetry of fifth cathodic sweeps for 1 × 10−6 mol L−1 of compound 6 at 100 mV s−1 scan rate is shown in , which confirmed that the HMDE surface was saturated by the analyte from the first sweep; thereafter, the reduction currents were sharply decreased.
Fig. 5 SW-AdSV voltammogram of 1 × 10−6 mol L−1 of compound 6 at B–R, pH 10, −0.6 V Eacc, 60 s tacc, 300 mV s−1, 10 Hz, 250 mV step potential and 0.6 mm2 over the potential range from −0.5 to −1.5 V.
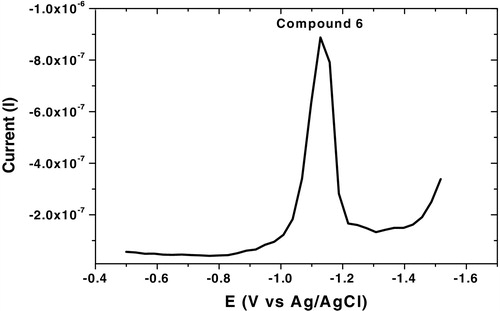
Fig. 6 Cyclic Voltammogram of 1 × 10−6 mol L−1 of compound 6 at B–R, pH 10, −0.6 V Eacc, 60 s tacc, 300 mV s−1, 10 Hz, 250 mV step potential and 0.6 mm2 over the potential range from −0.5 to −1.5 V.
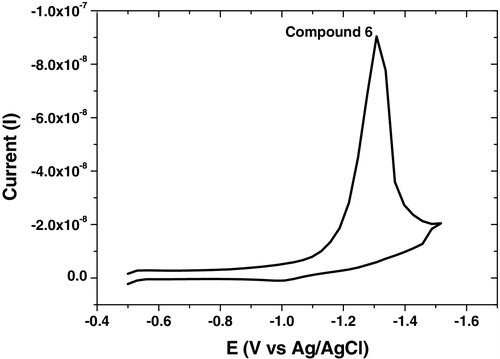
Fig. 7 Multi-Cyclic Voltammogram of 1 × 10−6 mol L−1 of compound 6 at B–R, pH 10, −0.6 V Eacc, 60 s tacc, 300 mV s−1, 10 Hz, 250 mV step potential and 0.6 mm2 over the potential range from −0.5 to −1.5 V.
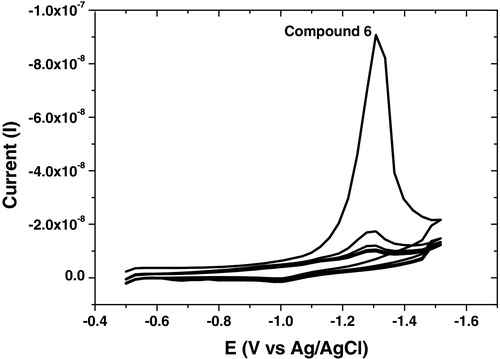
On other hand, SW-AdSV was also investigated for the determination of acyclonucleoside analogs (4) and (5) compared to compound (6) under optimum parameters ( and ). The analytical results revealed that compounds (4) and (5) gave high current (Ip) = 1550 nA at Ep= −1100 mV for each one; compared to that displayed by compound (6) (Ip) = 788 nA at Ep = −1150 mV. This finding may presumably be due to the higher inductive effect resulting from the presence of the two electron withdrawing hydroxyl groups in compound (6).
2.3 Analytical performance characteristics
Several analytical characteristics were investigated for the evaluation of the proposed SW-AdSV technique under the optimum conditions, such as calibration curve, recovery, stability and reproducibility.
2.3.1 Calibration curve
Under the optimum conditions, a linear relationship between the SW-AdSV peak current and compound (6) concentrations was observed () over the range 1 × 10−7 to 2 × 10−6 mol L−1. The calibration curve was calculated using the following least square equations:where Ip, Reduction current in nano-amperes; C, concentration of compound 6 in mol L−1; r, correlation coefficient; n, measurements number.
Fig. 10 SW-AdSV of compound 6 at B–R, pH 10, −0.6 V Eacc, 60 s tacc, 300 mV s−1, 10 Hz, 250 mV step potential and 0.6 mm2 (A = 1 × 10−7, B = 3 × 10−7, C = 5 × 10−7, D = 8 × 10−7, E = 1.2 × 10−6, F = 1.6 × 10−6, G = 2 × 10−6 mol L−1) over the potential range from −0.5 to −1.5 V.
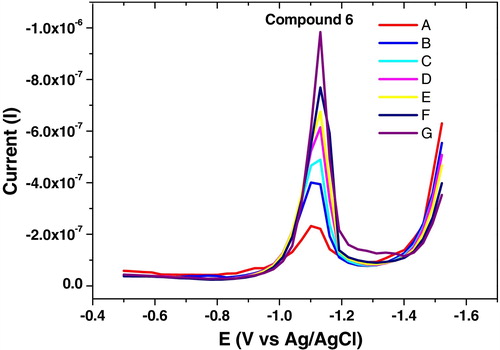
According to the calibration curve, the detection limit of SW-AdSV for compound (6) determination was calculated to be 3.4 × 10−9 mol L−1.
2.3.2 Recovery, stability and reproducibility
The precision and accuracy of the adopted SW-AdSV method was evaluated by the study of the recovery of 2.0 × 10−7 mol L−1, stability and reproducibility of 1.0 × 10−6 mol L−1 of compound (6), and yielded the 104% ± 1.0 recovery value. The cathodic current was almost stable for 80 min and 0.4% relative standard deviation (RSD %) was observed for eight measurements of the analyte.
2.4 Determination of compound 6 in spiked human biological samples
A 2.0 × 10−6 mol L−1 solution of compound 6 was pipetted into centrifuge tubes containing 0.5 mL of biological samples (human urine and plasma), and the centrifuging of the biological sample was run for 3 min. Into the centrifuge tube, 1.0 mL of ZnSO4·7H2O, 0.1 mL of NaOH and 1.0 mL of MeOH were added to the biological samples, and the mixture was centrifuged for 8 min at 4000 rpm. The solution was subsequently filtered using 0.45-μm filter papers, and a 0.5-mL volume of the liquid was transferred into the electrochemical cell and diluted to 10 mL with B–R buffer pH 10. The centrifuging operation was used for removing the interfering substances [Citation39]. The developed SW-AdSV was applied for the recovery of 2.0 × 10−6 mol L−1 of compound 6 in urine and plasma samples, which yielded 95.2% ± 1.1 and 90% ± 1.0, respectively.
2.5 Analytical results of HPLC
The HPLC technique was also investigated for the determination of the chemical behavior of 4-benzylideneamino-5-(2-phenyleth-1-yl)-3-[(2,3-dihydroxyprop-1-yl)thio]-1,2,4-triazole (6) in a mixture of methanol, acetonitrile and water mobile phase (70:20:10, v/v/v%) with UV detector at 254 nm and 1.0 mL/min flow rate. The HPLC signal for compound 6 was observed at 6.0 × 10−4 mol L−1 with 3.7 min as retention time (408 Abs) ().
3 Experimental
3.1 General methods
The melting points were determined on a Melt-temp apparatus and are uncorrected. TLC was performed on Merck silica gel 60 F254, and the spots were visualized by UV light absorption. The IR spectra were measured using potassium bromide pellets on a Perkin-Elmer 1430 series FT-IR spectrometer. 1H NMR spectra were recorded on an Advance Bruker NMR spectrometer at 400 MHz, and the 13C NMR spectra were recorded on the same instrument at 100 MHz using TMS as the internal standard. Elemental analyses were performed using a GmbH-Vario EL III Element Analyzer (Germany).
3.2 Synthesis and characterization of 4-amino-5-(2-phenyleth-1-yl)-2,4-dihydro-3H-1,2,4-triazole-3-thione (2)
Carbon disulfide (15 mmol) was added dropwise to a stirred solution of acid hydrazide 1 (10 mmol) in absolute ethanol (30 mL) containing potassium hydroxide (15 mmol) at 0 °C. Stirring was continued for 16 h at room temperature, and then the solution was diluted with diethyl ether. The resulting potassium dithiocarbazinate salt was filtered, washed with diethyl ether and was used without further purification since it was moisture-sensitive.
A mixture of the potassium dithiocarbazinate salt (10 mmol), hydrazine hydrate (20 mmol) and water (10 mL) was heated under reflux for 4 h. After cooling, it was diluted with water, and then acidified with aqueous hydrochloric acid. The crude triazole thus obtained was filtered and recrystallized from ethanol to give compound 2 in 90% yield, m.p. 229–230 °C, Lit mp: 227.9 °C [Citation34]. IR (υ, cm−1): 3275–3348 (NH, NH2), 3081 (Ar–H), 2913 (Ar–H), 1605 (C=N), 1295 (C=S). 1H NMR: δH 3.00 (t, 2H, 6.0 Hz, CH2), 3.15 (t, 2H, 6.0 Hz, CH2), 5.67 (s, 2H, NH2), 7.30–7.58 (m, 5H, Ar–H), 14.33 (s, 1H, NH triazole) ppm. 13C NMR: δC 31.12 (CH2), 35.46 (CH2), 115.78, 123.60, 127.82, 129.21, 132.56, 134.89, 161.84, 164.87 (Ar–C, C=N, C=S) ppm. Anal. Calc. for C10H12N4S: C 54.52, H 5.49, N 25.43. Found: C 54.63, H 5.41, N 25.29.
3.3 Synthesis and characterization of 4-benzylideneamino-5-(2-phenyleth-1-yl)-2,4-dihydro-3H-1,2,4-triazole-3-thione (3)
A mixture of triazole 2 (10 mmol) and benzaldehyde (10 mmol) was refluxed in ethanol (25 mL) containing HCl (0.5 mL) for 4 h. The solid thus formed on cooling was recrystallized from ethanol to give Schiff base 3 in 92% yield, mp: 180–181 °C. IR (υ, cm−1): 3325 (NH), 3068 (Ar–H), 2936 (Ar–H), 1612 (C=N), 1290 (C=S). 1H NMR: δH 3.02 (t, 2H, 6.0 Hz, CH2), 3.16 (t, 2H, 6.0 Hz, CH2), 7.27–7.75 (m, 10H, Ar–H), 9.74 (s, 1H, H–C=N), 14.29 (s, 1H, NH triazole). 13C NMR: δC 31.35 (CH2), 36.12 (CH2), 114.49, 116.14, 123.71, 126.50, 127.65, 129.44, 131.76, 132.24, 133.65, 140.14, 161.69, 163.04, 165.48 (Ar–C, C=N, C=S). Anal. Calc. for C17H16N4S: C 66.21, H 5.23, N 18.17. Found: C 66.17, H 5.37, N 18.24.
3.4 General procedure for the synthesis of acyclonucleoside analogs 4–6
To a solution of compound 3 (1 mmol) in an ethanolic solution of sodium ethoxide, prepared from sodium metal (0.023 g) in ethanol (15 mL), the appropriate hydroxyl alkylating agent (1.1 mmol) was added with stirring and later heated under reflux for 6 h. The reaction mixture was concentrated, cooled, diluted with water and left overnight. The precipitate formed was filtered, washed with water, and recrystallized from ethanol to yield acyclonucleoside analogs 4–6.
3.4.1 Characterization of 4-benzylideneamino-5-(2-phenyleth-1-yl)-3-[(2-hydroxyeth-1-yl)thio]-1,2,4-triazole (4)
This compound was obtained in 88% yield, m.p. 252–253 °C. IR (υ, cm−1): 3368 (OH), 3042 (Ar–H), 2918 (Ar–H), 1602 (C=N). 1H NMR: δ 3.02 (t, 2H, 6.0 Hz, CH2), 3.13 (t, 2H, 6.0 Hz, CH2), 3.25 (t, 2H, J = 4.4 Hz, SCH2), 3.76–3.81 (m, 2H, CH2O), 5.04 (t, 1H, J = 4.4 Hz, D2O exchangeable, OH), 7.31–7.70 (m, 10H, Ar–H), 9.71 (s, 1H, H–C=N) ppm. 13C NMR: δ 31.62 (CH2), 35.40 (CH2), 42.54 (SCH2), 61.21 (OCH2), 114.78, 116.80, 124.45, 126.23, 127.93, 129.79, 131.23, 132.78, 133.83, 140.46, 162.11, 163.42 (Ar–C, C=N) ppm. Anal. Calc. for C19H20N4OS: C, 64.75; H, 5.72; N, 15.90. Found: C 64.59; H 5.91; N 15.67.
3.4.2 Characterization of 4-benzylideneamino-5-(2-phenyleth-1-yl)-3-[(4-hydroxybut-1-yl)thio]-1,2,4-triazole (5)
This compound was obtained in 84% yield, m.p. 215–216 °C. IR (υ, cm−1): 3346 (NH), 3022 (Ar–H), 2914 (Ar–H), 1611 (C=N). 1H NMR: δ 1.86–1.97 (m, 4H, CH2CH2), 3.01 (t, 2H, 6.0 Hz, CH2), 3.14 (t, 2H, 6.0 Hz, CH2), 3.32 (t, 2H, J = 4.4 Hz, SCH2), 3.69 (t, 2H, J = 4.4 Hz, CH2O), 4.62 (bs, 1H, D2O exchangeable, OH), 7.29–7.70 (m, 10H, Ar–H), 9.70 (s, 1H, H–C=N) ppm. 13C NMR: δC 28.70 (CH2), 29.53 (CH2), 31.23 (CH2), 35.64 (CH2), 41.10 (SCH2), 60.43 (OCH2), 114.90, 116.62, 123.41, 126.62, 127.89, 130.32, 131.14, 132.54, 133.83, 140.70, 161.34, 163.56 (Ar–C, C=N) ppm. Anal. Calc. for C21H24N4OS: C, 66.29; H, 6.36; N, 14.72. Found: C, 66.43; H, 6.58; N, 14.84.
3.4.3 Characterization of 4-benzylideneamino-5-(2-phenyleth-1-yl)-3-[(2,3-dihydroxyprop-1-yl)thio]-1,2,4-triazole (6)
This compound was obtained in 87% yield, m.p. 268–269 °C. IR (υ, cm−1): 3325 (NH), 3068 (Ar–H), 2936 (Ar–H), 1612 (C=N), 1290 (C=S). 1H NMR: δH 3.03 (t, 2H, 6.0 Hz, CH2), 3.18 (t, 2H, 6.0 Hz, CH2), 3.24 (dd, 1H, J = 4.4 Hz, J = 12.8 Hz, SCH2), 3.30–3.39 (m, 3H, SCH2, CH2O), 3.57–3.64 (m, 1H, CHO), 4.70 (bs, 1H, D2O exchangeable, OH), 4.99 (bs, 1H, D2O exchangeable, OH), 7.22–7.70 (m, 10H, Ar–H), 9.76 (s, 1H, H–C=N) ppm. 13C NMR: δC 31.45 (CH2), 35.82 (CH2), 39.61 (SCH2), 64.49 (CH2O), 69.18 (CHO), 114.77, 116.46, 123.62, 126.90, 127.42, 129.08, 131.71, 132.42, 133.48, 140.57, 161.71, 163.46 (Ar–C, C=N) ppm. Anal. Calc. for C20H22N4O2S: C, 62.80; H, 5.80; N, 14.65. Found: C, 63.09; H, 5.69; N, 14.35.
3.5 Voltammetric measurements
The voltammetric measurements were usually were carried out using a 797 VA (Metrohm) controlled by VA computrace 2.0 control software at room temperature. The voltammetric technique was applied by conventional three electrodes system: hanging mercury drop electrode (HMDE), Ag/AgCl reference electrode and platinum auxiliary electrode. The pH values were recorded using a Hanna instrument pH 211. The analyte solution was initially purged with nitrogen gas for 100 s with stirring. In order to obtain voltammograms of the analyte under optimum conditions, 10 mL of supporting electrolyte was injected in dry and clean electrochemical cell, followed by the addition of the analyzed sample. The reduction scanning was applied over the potential range from −0.5 to −1.5 V for 60 s with stirring of the analyzed solution and 300 mV s−1 scan rate.
3.6 HPLC measurements
High-performance liquid chromatography technique was used for the analysis of novel triazole acyclonucleoside analogs using Ultimate 3000, Thermo Scientific Dionex with UV–vis detector, auto sampler, 0.01 mL manual loop injector and 5 μm C-18 column. For injection of triazole samples, micropipette 0.01–1.00 mL was used.
4 Conclusions
The condensation of 4-amino-5-(2-phenyleth-1-yl)-2,4-dihydro-3H-1,2,4-triazole-3-thione (2) with benzaldehyde in acidic media afforded the corresponding Schiff base (3) which, upon alkylation with hydroxyalkylating agents, furnished new acyclonucleoside analogs (4–6) in excellent yields.
In this study, efficient, accurate and fast square wave-adsorptive stripping voltammetric (SW-AdSV) as well as HPLC techniques have been successfully adopted for the determination of the newly synthesized triazolic acyclonucleoside analogs.
The described techniques were demonstrated to be convenient for the determination of all synthesized acyclonucleoside analogs and exhibited high sensitivity and specificity. The accuracy and precision of the presented methods were checked on the optimized parameters that divulged a dynamic linear concentration ranges with a low limit of detection over a wide range of concentrations. The accuracy and selectivity of the developed methods were demonstrated by recovery studies.
Acknowledgements
The authors would like to thank Dr Ziad Musa, Dr Mohammed Reda Aouad and Miss Nada Awaida for their assistance in accomplishing this research.
Notes
Peer review under responsibility of Taibah University
References
- R.LesykaO.VladzimirskaS.HolotaL.ZaprutkoA.GzellaEur. J. Med. Chem.422007641648
- G.T.ZitouniZ.A.KaplancıklıA.OzdemirP.ChevalletH.B.KandilciandB.GumusArch. Pharm. Chem. Life Sci.3402007586590
- G.ZitouniZ.A.KaplancikliK.ErolF.S.KilicII Farmaco541999218223
- M.MahdaviT.AkbarzadehV.SheibaniM.AbbasiL.FiroozpourS.A.TabatabaicA.ShafieeA.ForoumadiIran. J. Pharm. Res.92010265269
- M.RudrapalD.BiplabInt. Res. J. Pure Appl. Chem.32013232249
- Y.LiZ.S.YangH.ZhangB.J.CaoF.D.WangBioorg. Med. Chem.11200343634368
- R.VillarI.EncioM.MigliaccioM.G.GilV.Martinez-MerinoBioorg. Med. Chem.122004963968
- H.J.ShaefferL.BeauchampP.de MirandaG.B.ElionD.J.BauerP.CollinsNature (London)2721978583585
- J.C.MartinC.A.DvorakD.F.SmeeT.R.MatthewsP.H.JulienJ.P.VerheydenJ. Med. Chem.261983759761
- M.R.HardernR.L.JarvestT.H.BaconM.R.BoydJ. Med. Chem.39198716361642
- E.De ClercqP.De SomerA.Hol’yJ. Descam. Sci.2001978563565
- E.S.H.El AshryY.El KilanyAdv. Heterocycl. Chem.671997391438E.S.H.El AshryY.El KilanyAdv. Heterocycl. Chem.681997188E.S.H.El AshryY.El KilanyAdv. Heterocycl. Chem.691997129215
- E.S.H.El AshryN.RashedL.F.AwadA.A.H.Abdel-RahmanH.A.RasheedJ. Chem. Res. (S)22001129130E.S.H.El AshryN.RashedL.F.AwadA.A.H.Abdel-RahmanH.A.RasheedNucleosidesNucleotides Nucleic Acids252006299305
- E.S.H.El AshryA.A.H.Abdel RahmanN.RashedH.A.RasheedPharmazie541999893897
- E.S.H.El AshryA.A.H.Abdel RahmanN.RashedH.A.RasheedArch. Pharm. Med. Chem.3321999327330
- O.A.FarghalyR.S.Abdel HameedH.Abd-Alhakeemand Abu-NawwasInt. J. Electrochem. Sci.9201432873318
- R.JainR.K.YadavJ.A.RatherJ. Pharm. Anal.32013452455
- D.A.QinX.Q.CaiQ.MiaoZ.H.WangM.L.HuInt. J. Electrochem. Sci.9201416081620
- N.T.R.LaurianeR.NajihA.ChtainiPharm. Anal. Acta52013295299
- R.RamachandranV.ManiS.-M.ChenG.G.KumarM.GovindasamyInt. J. Electrochem. Sci.102015859869
- M.BrychtS.SkrzypekK.KaczmarskaB.BurnatA.LeniartN.GutowskaElectrochim. Acta1692015117125
- M.PordelS.A.BeyramabadiA.MohammadinejadDyes Pigments10220144652
- H.S.El-DesokyM.M.GhoneimM.M.Abdel-GaleilJ. Braz. Chem. Soc.212010669679
- A.F.Al-GhamdiAm. J. Anal. Chem.22011174181
- S.EvrimK.TekkeliJ. Anal. Methods Chem.2013201319
- M.L.AltunTurk. J. Chem.262002521528
- E.E.ErtaşH.ÖzerC.AlasalvarFood Chem.1052007756760
- T.K.DasguptaP.D.MelloD.BhattacharyaPharm. Anal. Acta62015114
- L.VlaseD.MunteanS.C.CobzacL.FilipRev. Roum. Chim.592014719725
- H.DodziukA.BielejewskaW.SchilfH.UedaCent. Eur. J. Chem.9201110561061
- E.S.H.El AshryN.RashedL.F.AwadE.RamadanS.M.Abdel-MaggeedN.RezkiNucleosidesNucleotides Nucleic Acids262007423435
- E.S.H.El AshryN.RashedL.F.AwadE.RamadanS.M.Abdel-MaggeedN.RezkiJ. Carbohydr. Chem.2720087085
- M.R.AouadN.RezkiM.MessaliE.H.El AshryNucleosidesNucleotides Nucleic Acids20133241
- T.J.CheeserightM.HolmF.LehmannS.LuikM.GottertJ.L.MelvilleS.LauferJ. Med. Chem.52200942004209
- E.S.H.El AshryA.A.KassemH.Abdel-HamidF.F.S.LouisA.N.KhattabM.R.AouadARKIVOC2006119132
- V.SumangalaB.PoojaryN.ChidanandaT.ArulmoliS.ShenoyEur. J. Med. Chem.5420125964
- M.AshokB.S.HollaJ. Pharmacol. Toxicol.22007256263
- I.KhanA.IbrarN.AbbasEur. J. Med. Chem.632013854868
- A.F.AlghamdiM.M.HefnawyY.El-ShabrawyDigest J. Nanomater. Biostruct.92014355368