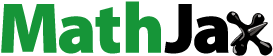
Abstract
Standardized field experiments were carried out to study the performance of five rice genotypes derived from different germplasm in terms of yield, harvest index (HI) and grain quality at eight agro-ecological sites of the tropics and subtropics across Asia during 2001 and 2002. Considering that indica and javanica genotypes adapt to warm climatic conditions, and japonica genotypes to cool agro-climatic conditions, it is hypothesized that indica × japonica hybrids may combine high yields and good quality traits under a wide range of agro-climatic conditions. Grain yield, HI, protein content and amylose content varied considerably among genotypes and environments. Mean rice yields of genotypes ranged from 1.5 to 11 t ha−1 across the eight sites; on average yields were 7.2 t ha−1 under subtropical and 2.7 t ha−1 under tropical conditions. The much lower yields in tropical environments resulted from a low biomass as well as a low HI. Among the genotypes, the indica × japonica hybrid showed the highest yield under subtropical conditions, and a higher yield than the japonica genotypes and the indica × javanica hybrid but lower than the indica genotype under tropical conditions. Phenology of genotypes varied strongly across environments. Low yields at tropical locations were associated with a low light capture due to short growth duration. Post-anthesis light-use efficiencies and the photothermal quotient explained much of the variation in yield. Protein content varied among genotypes depending on location and year. Variation in amylose content of rice grains was mainly associated with genotypic differences and much less with environmental conditions, but contents decreased with higher post-anthesis ambient temperatures. The indica × japonica hybrid combined high yields with a favourable amylose content and showed a better ability to adapt to cool and to warm agro-climatic conditions than the indica or japonica genotypes. Our study showed the magnitude of yield penalties associated with growing rice genotypes in environments to which they are not adapted. The consequences of these findings for improved adaptation of rice are discussed.
1 Introduction
Asia's irrigated rice area of 73 Mha produces more than 70% of the world's rice production [Citation1]. About two-thirds of this area is located in the tropics and the remainder in the subtropics. Although estimates of future rice demand vary considerably [Citation2,Citation3], rice yields will have to increase as area and quality of arable land will decrease as a result of competition for land from urbanization, industrialization and conservation schemes. As the basic dietary energy supply in Asia improves, demand for rice with superior quality properties increases. Grain quality will become even more important in the future, when the economic situation of the very poor – many of whom depend on rice as their staple food – improves and their demand for higher-quality rice increases [Citation4].
The nutritional value of rice is related to its protein content, while its amylose content is an important indicator of cooking and consumption quality [Citation5]. A low amylose content increases water absorption, volume expansion, and stickiness of cooked rice [Citation6], whereas a high amylose content is associated with hard grains after cooking [Citation7]. Preferences for soft and hard rice grains vary widely across Asia. For example, a relatively low amylose content is preferred in Taiwan, rice with a low to intermediate amylose content in Korea, whereas consumers in the Philippines prefer soft rice with an intermediate amylose content [Citation8].
Yield and quality characteristics of rice are affected by environmental, management and genotypic factors and their interactions [Citation9]. A cross-regional study with a wide range of genotypes may provide insight into the opportunities to combine high yield and favourable grain quality.
Yields in tropical areas are often below 5 t ha−1, whereas in subtropical areas they often exceed 6 t ha−1 [Citation10–Citation12]. Comparing the productivity of rice in subtropical and tropical areas, Ying et al. [Citation13] showed that high-yielding genotypes (indica, inbred or hybrid) produced 48% more per unit area of land under subtropical than under tropical conditions. The faster leaf growth during the vegetative stage, leading to higher LAI, higher crop growth rates and more biomass under subtropical vs. tropical conditions were associated with larger panicles (more spikelets per panicle).
Many reports point out that rice yields are affected by genotypic traits, seasons and a genotype × environment (G × E) interaction [Citation14–Citation16]. Kropff et al. [Citation17] found for irrigated rice in the tropics with ample water and N supply that attainable yields in the wet and dry season amounted to 6 and 10 t ha−1, respectively. So seasonal differences should also be taken into account when evaluating crop performance across environments.
A quantitative analysis of the adaptation ability of different genotypes, especially hybrid genotypes, to different climatic conditions may help to assess the magnitude of the G × E interaction. This will facilitate designing new genotypes, identifying test conditions, and selecting for specific well-defined conditions [Citation18,Citation19], including changes in climatic conditions [Citation20]. Given that indica and japonica genotypes are adapted to warm and cool agro-climatic conditions, respectively [Citation21,Citation22], it is hypothesized that hybrid genotypes may out-perform their parental lines under a wide range of agro-climatic conditions. A quantitative assessment of the adaptive capacity of rice genotypes can be made in experiments that include indica, japonica as well as different hybrid genotypes and both tropical and subtropical environments.
The objectives of this study were: (1) to evaluate grain yield and quality performance of five rice genotypes derived from different germplasm grown in two tropical and six subtropical environments in Asia, (2) to examine the variation in grain yield and quality caused by G × E interactions, and (3) to explore the opportunities and constraints on combining high yields and favourable grain quality.
2 Materials and methods
2.1 Experimental locations
Two-year multi-varietal field experiments were carried out in South and East Asia in the framework of the Asian Rice Network (ARICENET), according to the agro-ecological zones in Asia [Citation1] and a standard protocol for experimental design, crop management, observations, measurements and processing of collected data [Citation23]. In 2001, the experiments included seven locations, five of which were in subtropical regions, i.e., Iwate (39°21′N, 141°13′E), Nagano (35°51′N, 138°10′E), Shimane (35°30′N, 132°36′E), and Kyoto (35°01′N, 135°45′E) in Japan, Nanjing (32°06′N, 118°45′E) in China, and two in tropical regions, i.e., Chiangmai (18°47′N, 98°59′E) and Ubon Ratchathani (15°20′N, 104°52′E) in Thailand. In 2002, the subtropical location Taoyuan (26°16′N, 101°02′E) in China was added.
2.2 Experimental design and crop management
At each location, five genotypes were tested. The genotypes selected for this study were derived from different germplasms: indica genotype IR72, japonica genotypes Nipponbare and Takenari, indica × japonica hybrid genotype Takanari, and indica × javanica hybrid IR65564-44-2-2 (). Based on morphological characteristics, the indica, japonica and javanica genotypes are considered three different types of rice, whereas isozyme studies showed that javanica genotypes belong to the japonica genotypes and are designated as tropical japonicas, usually grown in tropical climates [Citation22,Citation24].
Table 1 Rice genotypes used in the experiments.
All experiments were laid out as a randomized block design with 3 replications and an individual plot size of 20 m2. At transplanting, 40 kg N ha−1 was applied, and 20 kg N ha−1 was top-dressed every 20 days until 10 days after heading so that N fertilizer supply (in kg ha−1 per day) varied from 0.94 at Nagano and Shimane to 1.05 at Chiangmai. In addition, 120 kg P2O5 ha−1 and 120 kg K2O ha−1 were applied as a basal dressing. Rice seedlings with 4–5 leaves, depending on genotype, were transplanted at 2 plants per hill, spaced 30 cm between and 15 cm within rows. At the tropical locations Ubon and Chiangmai, the experiments were carried out from June to November in both years. During the growing season, the experimental fields were continuously flooded, and weeds, pests and diseases were adequately controlled with biocides.
2.3 Observations and measurements
In this study the vegetative period was defined as the time from emergence to panicle initiation, the reproductive period from panicle initiation to flowering, and the post-anthesis period from flowering to physiological maturity. Panicle initiation was identified as the stage at which the panicle was visible as a white feathery cone of 1.0–1.5 mm in the main tiller, flowering as the moment the first stamen became visible, and physiological maturity as the moment when less than 10–15% of the grains were still green-coloured.
At transplanting, 20 plants per plot were sampled of each genotype, and at 20 days after transplanting, at panicle initiation, 2 weeks before flowering, at flowering, 2 weeks after flowering, and at maturity 8 hills of each genotype were harvested to measure leaf area, and calculate leaf area index (LAI). At maturity, plants were partitioned into leaves, stems plus leaf sheaths, and panicles. Samples were dried in an oven at 80 °C until constant weight. Grain yields were measured by harvesting a sample of 2 m2 per plot; yield was expressed at a moisture content of 14%. HI was calculated as grain yield divided by total aboveground dry weight.
Grain samples for quality analyses were collected only at Iwate, Kyoto and Nanjing in 2001, and at Iwate, Shimane, Nanjing, Taoyuan and Chiangmai in 2002, due to logistical problems. Grain samples were dried and ground in preparation for protein and amylose analysis. N content was determined with micro-Kjeldahl digestion, distillation, and titration, and converted to protein content using a factor 5.95. Amylose content was determined according to the modified assay described by Juliano et al. [Citation25] and Juliano and Villareal [Citation4].
Daily weather data were recorded in weather stations at each experimental location. Data for total radiation (RD), maximum (Tmax) and minimum (Tmin) temperature were derived from these stations.
2.4 Data analysis
GenStat for Windows, 8th edition (http://www.vsn-intl.com/genstat/) was used to identify effects of genotype, location and their interactions on yield, HI, protein content and amylose content. The method of residual maximum likelihood (REML) was used to obtain efficient estimates of treatment effects in unbalanced designs and analyse incomplete data sets [Citation26] (data of Taoyuan for 2001, and data of two genotypes at Ubon for 2001 were missing). Statistical differences were determined using Wald statistics.
Intercepted photosynthetically active radiation (IPAR) was calculated from radiation (RD) using the following equation:where k is the light extinction coefficient of rice, which was set to 0.4–0.6 depending on crop development stage as used in the rice crop growth model ORYZA2000 [Citation27], and L the leaf area index of the canopy, calculated by linear interpolation of measured LAI at various growth stages.
Light-use efficiency (LUE) was calculated as total aboveground biomass divided by total IPAR.
The photothermal quotient (PTQ, the ratio of average radiation intensity to average temperature above a threshold base temperature) has been proposed as a single indicator to capture the combined effect of the yield-determining factors radiation and temperature on yields [Citation28]. PTQ is a gross measure of light energy available for photosynthesis per unit of development. Considering that the number of spikelets is closely related to crop growth from panicle initiation and flowering [Citation29] and given the differences in crop phenology at the different locations, PTQ was calculated over a period of 30 days prior to anthesis using intercepted radiation [Citation30]:where IRD is intercepted global radiation, Tmin and Tmax are the daily minimum and maximum temperatures, respectively, and Tb is the base temperature, which for rice was set to 8 °C.
Thermal time was calculated by accumulating daily average temperatures above the base temperature.
The expolinear equation from Goudriaan [Citation31] was modified to describe the relationship between PTQ and grain yield.
3 Results
3.1 Weather and phenology
Daily average radiation and minimum and maximum temperatures during the three phenological phases – vegetative, reproductive and post-anthesis – at the eight locations are listed in . The variation in radiation among growth periods was generally smaller in the tropical than in the subtropical environments. On average, radiation during the vegetative and reproductive growth periods was higher at the subtropical locations, but was highest during the post-anthesis period at the tropical locations Chiangmai and Ubon.
Table 2 Daily average incoming radiation and minimum and maximum temperatures during the vegetative, reproductive, and post-anthesis phasesFootnotea of rice at eight locations in Asia (averaged over 2001 and 2002).
Both, minimum and maximum temperatures at the subtropical locations were higher during the reproductive growth period than during the vegetative and post-anthesis periods, whereas at the tropical locations (wet season) temperatures were lower during the post-anthesis period than during the vegetative period. Minimum and maximum daily temperatures during post-anthesis were lowest at Iwate (12.0 and 21.1 °C, respectively), i.e., 10 °C lower than at Chiangmai and Ubon.
The three phenological phases expressed as thermal time (°C days) for the five genotypes at eight locations are shown in . The variation in the duration of growth phases among genotypes differed across locations. At the cooler locations in Japan, the vegetative phase of genotype TA was shorter (<1000 °C days) compared with the other genotypes, whereas at the subtropical locations in China, the vegetative phase of genotype TA did not differ from the other genotypes. The japonica genotypes NI and TE had a shorter reproductive phase at the tropical locations (Chiangmai and Ubon), resulting in early heading at these locations. TA had a longer post-anthesis phase than the other genotypes at the sites in Japan, and a shorter post-anthesis phase than genotypes NI and TE at Chiangmai. Generally, the duration of the vegetative phase was longer than the reproductive and post-anthesis phases except for the tropical location Ubon, where the genotypes TA, IR and NP had similar durations of the vegetative and reproductive phases.
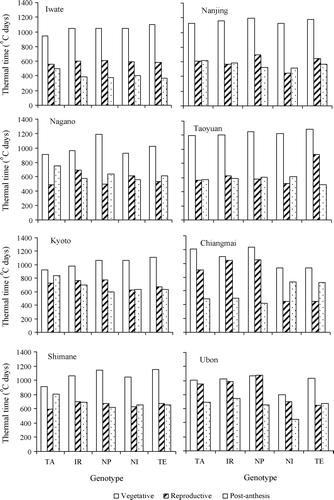
3.2 Yield variation across locations and genotypes
The effect of the G × E interaction on rice yield was statistically significant as a consequence of growing a wide range of genotypes across contrasting climatic environments (). In subtropical environments the crops produced higher yields than in tropical environments (). Under subtropical conditions, the indica × japonica hybrid TA produced the highest (almost 11 t ha−1) and the indica × javanica hybrid NP the lowest yield (about 3.0 t ha−1). Under tropical conditions, the indica genotype IR72 had the highest yield (3.8 t ha−1), whereas the japonica genotypes NI and TE had the lowest yield (about 1.5 t ha−1). Details are presented in and A.
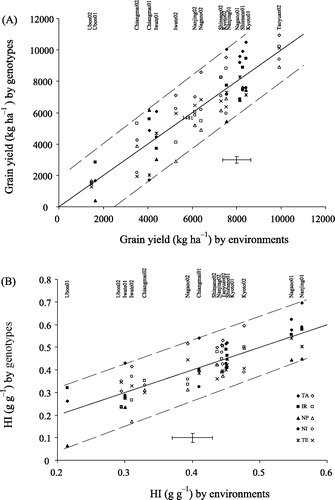
Table 3 Contribution to total variation of yield, harvest index (HI), protein and amylose content of five rice genotypes across locations, by environment, genotype and their interaction. Results of analysis of variance (ANOVA).
Table 4 Rice yields (kg ha−1) of five genotypes at different locations in Asia (averages for 2001 and 2002).
Grain yields were consistently low at Iwate and, especially at Chiangmai and Ubon (tropical climate), i.e., on average 4.8, 3.8 and 1.5 t ha−1, respectively (). Yields of the genotypes TA, IR and TE were highest (on average: 10.9, 10.6 and 10.4 t ha−1, respectively) at Taoyuan (subtropical climate), whereas the genotypes TA (10.0 t ha−1) and IR (9.1 t ha−1) scored second at Kyoto (A). No grain yields were obtained for the japonica genotypes NI and TE at Ubon in 2001 due to their very early heading. The factor ‘environment’ accounted for 72% of the yield variation (sum of squares), genotype for 8%, and their interaction for 9% ().
3.3 Harvest index
The average harvest index (HI) varied significantly among locations (B), from the lowest value at Ubon to the highest at Nanjing, illustrating the effect of growth conditions on HI. On average, HI varied from 0.20 to 0.50 g g−1 across locations, with a large variation within sites due to genotypic differences. The effects of location and genotype and their interactions were statistically significant (). A good yield performance was strongly reflected in a high HI; genotype TA showed a much higher HI than other genotypes in most environments. Variations in HI were strongly associated with post-anthesis temperatures among locations (r2 = 0.66), whereas differences among genotypes showed a weaker association (r2 = 0.22).
3.4 Growth conditions and grain setting
Biomass accumulation during the pre-flowering period is an important yield determining factor. A higher net photosynthesis per unit of thermal time will result in a greater availability of assimilates for the developing inflorescences and as a consequence in more spikelets and grains per unit area of land. Hence, the sink capacity increases, resulting in a higher yield potential. To show this relationship, an expolinear curve was fitted to the relation between grain yield and PTQ during the period 0–30 days before anthesis (). Yield per unit area of land did not increase linearly until PTQ exceeded a value of about 0.48 MJ m−2 d−1 per °C, indicating that the positive effect of pre-flowering PTQ was present only above this threshold value.
3.5 Light capture and light-use efficiency
Light capture was higher before than after flowering for all genotypes and it was lowest for genotype NI before as well as after flowering (). On average, the capability of light capture decreased at tropical locations, where intercepted PAR is only half of that at subtropical locations.
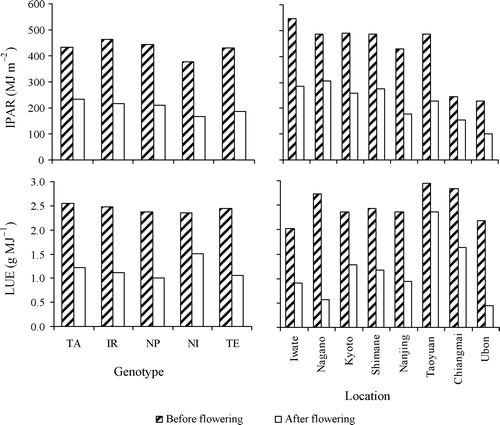
Light-use efficiency (LUE) was higher before than after flowering, and differed strongly across locations (). The LUE of crops at Taoyuan was highest after flowering whereas at Ubon it was lowest. LUE before flowering varied slightly around 2.5 g MJ−1 among genotypes (), whereas after flowering genotype NI had a somewhat higher LUE (1.5 g MJ−1) than other genotypes (1.0–1.2 g MJ−1).
3.6 Protein content
The grain protein content across environments varied from 7% to 8.5% (A), with on general the highest contents for the indica × japonica hybrid TA and the lowest for the indica × javanica hybrid NP. Protein content across environments varied most for the indica and japonica genotypes, contributing to a much higher G × E interaction effect than other sources of variation ().
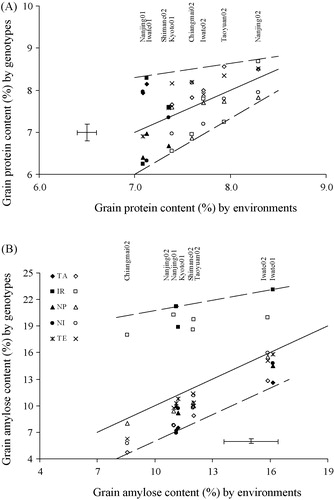
The statistical analysis showed that year × genotype (Y × G) interaction effects on protein content were statistically significant. For example, the variation in protein content of genotypes at the Nanjing site was larger in 2001 than in 2002. Regression analysis showed that protein content was not positively or negatively correlated with yield, indicating that under the prevailing growing conditions there was ample N supply and therefore no N dilution occurred at higher yield levels.
3.7 Amylose content
Average amylose content varied from 8% to 16% among locations and from 5% to 22% among genotypes (B). These differences were statistically significant (). The variation in amylose content is mainly explained by genotype (68%) and less by environment (25%). The highest amylose content was found for genotype IR at Iwate (23%) and the lowest for genotype TA (5%) at Chiangmai. Amylose content of the japonica genotypes and hybrids increased linearly and significantly with decreasing daily average temperatures post-anthesis (), but the amylose content of the indica genotype IR was less sensitive to post-anthesis temperatures.
4 Discussion
4.1 Genetic adaptation for yield responses to environment
About 72% of yield variation was explained by environment and 8% by genotype. The relative contribution of these factors to yield variation may be different for other combinations of genotype and environment [Citation14–Citation16], but environmental factors remain the most important for explaining yield differences of rice grown in tropical and subtropical areas [Citation11,Citation13]. Indica genotypes usually produce higher yields in the tropics than japonica genotypes, whereas under cooler conditions, such as at Iwate (Japan), japonica genotypes perform better due to morphological and physiological adaptation [Citation21,Citation22]. The observed yield variations illustrate that poor adaptation of japonica genotypes to tropical environments resulted in high yield losses in this study (A). The same applies to indica genotypes that are poorly adapted to cool subtropical environments. However, the indica × japonica hybrid showed a yield gain in subtropical environments (B and ), whereas in tropical environments this hybrid did not attain such a yield gain but showed a much better adaptation than the japonica genotypes. The indica × javanica hybrid performed far worse than the indica genotype in cool subtropical environments. The reason may be that javanica genotypes are adapted to tropical environments [Citation22,Citation24]. Maybe the wider adaptation of hybrid genotypes to different climatic conditions can only be expected from germplasm with contrasting morphological and physiological traits such as in genotype TA.
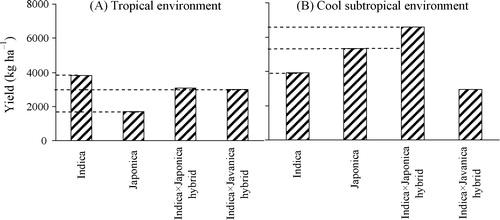
The observed variations in phenology expressed as accumulated thermal time are associated with the ability of genotypes to adapt to different temperature regimes. Poor adaptation may lead to an unfavourable development, resulting in yield loss, such as occurred at Ubon in 2001 with the japonica genotypes NI and TE due to very early heading. The very low yield at Ubon is consistent with reported values [Citation32,Citation33]. Cooper et al. [Citation34] reported that a major factor contributing to Y × G × E interactions for grain yield in North-East Thailand was the variation in days-to-flower. Yoshida et al. [Citation35] reported low numbers of spikelets per unit area of land for most genotypes grown at Ubon, which may indicate a higher sterility due to heat.
Our data show that the thermal time for the same cultivar varied among different environments, and similar results were observed in other studies [Citation36,Citation37]. Yin et al. [Citation38] re-analysed the reported data and concluded that the nonlinear relation between development rate and temperature, and the effect of the temperature amplitude may explain large part of the variation among environments.
IPAR at the tropical locations Chiangmai and Ubon was low, resulting from a poor canopy development and low LAI. The higher LUE during pre-flowering than during post-flowering found in our study was consistent with the results of Campbell et al. [Citation39]. The high LUE during the post-flowering period at Taoyuan was strongly associated with high yields, whereas the low LUE combined with a low light capture at Ubon resulted in the lowest yield. The LUE of 2.76 g MJ−1 (over the entire growing season) at Taoyuan is comparable with values reported by Sinclair and Muchow [Citation40] for high-yielding rice.
PTQ integrates the effects of the yield-determining factors solar radiation and temperature on yields [Citation28]. The expolinear relationship between grain yield and pre-anthesis PTQ indicates a threshold of 0.48 MJ m−2 d−1 per °C, which may be associated with a critical carbohydrate availability to develop spikelets found in sorghum [Citation41]. Generally, grain yield increases linearly with PTQ [Citation28,Citation30], but in our study the relationship was analysed over a wider range of agro-climatic conditions allowing to identify a threshold value.
In addition to temperature and radiation, yield variation may also be associated with differences in yield-limiting factors as determined by nutrient supply, water availability and soil characteristics [Citation42]. Especially indigenous soil-N supply strongly varies across Asian agro-ecosystems [Citation11]. The effect of indigenous soil-N supply on yield decreases with higher N-fertilizer applications [Citation43]. In the experiments, average N-fertilizer supply was about 1 kg N ha−1 day−1, which might have reduced the effect of differences in indigenous soil-N supply on crop yields among locations (0.4–0.8 kg N ha−1 day−1 according to Dobermann et al. [Citation11]). However, the N uptake of crops peaks during the period of canopy development and may exceed 3 kg N ha−1 day−1 [Citation44]. Therefore, differences in N availability between locations cannot be completely ruled out despite the standardized management practices. The rather low yield at Ubon may also be caused by low soil fertility due to poor soil structure, and low pH [Citation45,Citation46]. The influence of different soil characteristics on yield may be further explored using simulation models.
4.2 Grain quality
The quality of rice grains depends on consumer preferences and the intended end-use of the grain. Protein content showed small but statistically significant differences among the genotypes (7–8.5%) and environments (6.2–8.7%); the observed protein contents were within the 4–14% range commonly found in Asian rice genotypes [Citation4].
Amylose content of rice grain – the soluble component of starch – is especially important for its cooking behaviour and thus directly related to the preference of consumers [Citation5]. Juliano [Citation7] indicated that amylose content is lower at higher temperatures during post-anthesis. Umemoto et al. [Citation47] reported higher amylose contents with lower temperatures during the post-anthesis phase due to higher activity of the granule-bound starch synthase in the endosperm. In our study, the lowest post-anthesis temperatures at Iwate and highest at Chiangmai may lead to the highest amylose content at Iwate and the lowest at Chiangmai. Overall, amylose content of all genotypes was linearly and inversely correlated with temperature during the post-anthesis period (). The close relationship between amylose content and temperature during post-anthesis, in addition to the genotypic amylose traits, suggests that amylose content of the rice grain can be predicted with reasonable accuracy.
4.3 Options for combining high grain yield and favourable grain quality
The protein content of rice is mainly affected by N supply [Citation48,Citation49]. Nitrogen availability increases yield and protein content [Citation48,Citation50]; thus N-management strategies are key to attaining high yields and a high grain protein content simultaneously. Without having optimized N management in the experiments, the indica × japonica hybrid TA at Taoyuan combined the highest overall yield with one of the highest observed protein contents among the tested genotypes and locations ().
The amylose content of genotype TA differed almost a factor three between tropical Chiangmai and cool subtropical Iwate (B) under ambient temperatures during grain filling, while the associated yields belong to the highest attained at both locations (A and ) due to this genotype's wide adaptive capacity. TA could meet the grain amylose content that is needed for a favourable end-use quality with little yield loss compared with other genotypes.
5 Conclusions
Variations in rice yields under given management in contrasting agro-ecological environments were mainly the result of differences in climatic conditions rather than in genotypic traits. The indica × japonica hybrid TA showed a better ability to adapt to both cool and warm agro-climatic conditions than the indica genotype IR and the japonica genotypes that only performed well in warm and cool environments, respectively. Crop yields were much lower in tropical than in subtropical regions for all genotypes as a consequence of both low biomass production and low HI, resulting from a fast crop phenological development that restricted light capture. Environments with lower temperatures and less radiation, as at Iwate (Japan), may also restrict biomass production due to a retarded leaf development and a reduced light-use efficiency (LUE). Favourable subtropical conditions like at Taoyuan (China) resulted in high-yielding crops that showed a high LUE after flowering. PTQ, reflecting the pre-anthesis growing conditions, is a strong determinant of yield formation.
Genotypic differences explained 68% of amylose variation while grain amylose content was linearly and inversely correlated with the average post-anthesis ambient temperature. Genotypes with wide adaptation ability, such as hybrid TA, may combine high yield with favourable amylose contents and high protein contents.
Our study shows that there are considerable risks of yield penalties on growing rice cultivars in environments to which they are not adapted. Temperature is a strong driving force for development and growth, affecting biomass production, reproductive capacity, grain yield and grain quality. Climate change is associated with rising temperatures and therefore current rice cultivars may need to be replaced with cultivars that have more appropriate thermal time requirements for given conditions [Citation51]. Developing new cultivars that perform over a wide range of environmental conditions may improve the ability of rice to adapt to weather conditions associated with climate change.
Acknowledgements
This work was supported by the National Natural Science Foundation of China (30800664) and the ARICENET project ‘Development of a Rice Simulator Interfacing Gene Function to Field Performance’. We particularly wish to acknowledge the support of Mrs. Sasaki, Tamura and Akita (Iwate Agricultural Experimental Center), Mr. Kuroda (Iwate University), Mrs. N. Inoue and Hagiwara (Shinshu University), Mrs. Kobata, Ohnishi and Kobayashi (Shimane University), Mrs. T. Shiraiwa, Nakagawa, Matsui and K. Katsura (Kyoto University), Mr. Jongkaewwattana (ChiangMai University), and Mrs. W. Zou (Yunnan Agricultural University).
References
- J.L.MacleanD.C.DaweB.HardyG.P.HettelRice Almanac2002IRRILos Baños, Philippines253 pp
- V.SmilFeeding the world: How much more rice do we need?H.K.L.ToriyamaK.B.HardyWorld Rice Research Conference International Rice Research InstituteLos Baños, Philippines, Tokyo and Tsukuba, Japan(2005) 21–23.
- M. Hossain, A balancing act. Rice facts, Rice Today vol. April–June, 2007, pp. 37.
- B.O.JulianoC.P.VillarealGrain quality evaluation of world riceProceedings of the International Rice Research Institute (IRRI)Los Baños, Philippines(1993) 205 pp.
- B.O.JulianoCriteria and tests for rice grain qualitiesB.O.JulianoRice Chemistry and TechnologySt Paul, MN, USA(1985) American Association of Cereal Chemists. 443–524.
- S.R.DelwicheK.S.McKenzieB.D.WebbQuality characteristics in rice by near-infrared reflectance analysis of whole-grain milled samplesCereal Chemistry731996257263
- B.O.JulianoVarietal impact on rice qualityCereal Foods World431998207211214–216, 218–222.
- O.J. Loewer, D.H. Loewer, G.E. Miller, C. Mutters, J.F. Thompson, J. Williams, Rice Quality Workshop, 2003. http://www.plantsciences.ucdavis.edu/uccerice/QUALITY/contents.htm.
- J.YangJ.ZhangZ.WangQ.ZhuL.LiuActivities of enzymes involved in sucrose-to-starch metabolism in rice grains subjected to water stress during fillingField Crops Research8120036981
- P.RomyenP.HanviriyapantS.RajatasereekulS.KhunthasuvonS.FukaiJ.BasnayakeE.SkulkhuLowland rice improvement in northern and northeast Thailand 2. Cultivar differencesField Crops Research591998109119
- A.DobermannC.WittS.AbdulrachmanH.GinesR.NagarajanT.SonP.TanG.WangN.ChienV.ThoaC.PhungP.StalinP.MuthukrishnanV.RaviM.BabuG.SimbahanM.AdvientoSoil fertility and indigenous nutrient supply in irrigated rice domains of AsiaAgronomy Journal952003913923
- Q.JingH.van KeulenH.HengsdijkW.CaoP.S.BindrabanT.DaiD.JiangQuantifying N response and N use efficiency in rice-wheat (RW) cropping systems under different water managementThe Journal of Agricultural Science1472009303312
- J.YingS.PengQ.HeH.YangC.YangR.VisperasK.CassmanComparison of high-yield rice in tropical and subtropical environments. I. Determinants of grain and dry matter yieldsField Crops Research5719987184
- L.WadeC.McLarenL.QuintanaD.HarnpichitvitayaS.RajatasereekulA.SarawgiA.KumarH.AhmedA.SarwotoR.SinghJ.RodriguezS.Siopongco SarkarungGenotype by environment interactions across diverse rainfed lowland rice environmentsField Crops Research6419993550
- S.O.P.SamonteL.T.WilsonA.M.McClungJ.C.MedleyTargeting cultivars onto rice growing environments using AMMI and SREG GGE biplot analysesCrop Science45200524142424
- T.L.B.AcuñaH.R.LafitteL.J.WadeGenotype × environment interactions for grain yield of upland rice backcross lines in diverse hydrological environmentsField Crops Research1082008117125
- M.J.KropffK.G.CassmanH.H.van LaarS.PengNitrogen and yield potential of irrigated ricePlant and Soil1561993391394
- P.JacksonM.RobertsonM.CooperG.L.HammerThe role of physiological understanding in plant breeding: from a breeding perspectiveField Crops Research4919961137
- W.YanL.A.HuntGenotype-by-environment interaction and crop yieldPlant Breeding Reviews161998135178
- R.WassmannS.V.K.JagadishS.HeuerA.IsmailE.RedonaR.SerrajR.K.SinghG.HowellH.PathakK.SumflethL.S.DonaldClimate Change Affecting Rice Production: The Physiological and Agronomic Basis for Possible Adaptation Strategies, Advances in Agronomy, Vol. 1012009Academic Presspp. 59–122.
- H.MorishimaH.OkaPhylogenetic differentiation of cultivated rice. II Numerical evaluation of the indica-japonicaJapanese Journal of Breeding311981402413
- J.C.GlaszmannIsozymes and classification of Asian rice varietiesTheoretical and Applied Genetics7419872130
- T.HorieH.YoshidaT.ShiraiwaH.NakagawaE.KurodaT.SasakiM.HagiwaraT.KobataM.OhnishiK.KobayashiAnalysis of genotype by environment interaction in yield formation processes of rice grown under a wide environmental range in Asia. 1. Asian Rice Network (ARICENET) research and preliminary resultsJapanese Journal of Crop Science7220038889
- G.S.KhushOrigin, dispersal, cultivation and variation of ricePlant Molecular Biology3519972534
- B.O.JulianoC.M.PerezA.B.BlakeneyD.T.CastilloN.KongsereeB.LaigneletE.T.LapisV.V.S.MurtyC.M.PauleB.D.WebbInternational cooperative testing on the amylose content of milled riceStarch331981157162
- S.J.WelhamR.ThompsonLikelihood ratio tests for fixed model terms using residual maximum likelihoodJournal of the Royal Statistical Society: Series B (Statistical Methodology)591997701714
- B.A.M.BoumanM.J.KropffT.P.TuongM.C.S.WopereisH.F.M.ten BergeH.H.van LaarORYZA2000: Modeling Lowland Rice2001International Rice Research InstituteLos Baños, Philippines235 pp.
- H.A.NixClimate and crop productivity in AustraliaS.YoshidaClimate and Rice1976International Rice Research InstituteLos Baños, Philippines495507
- M.J.KropffK.G.CassmanS.PengR.B.MatthewsT.L.SetterQuantitative understanding of yield potentialK.G.CassmanBreaking the Yield Barrier1994International Rice Research InstituteLos Baños, Philippines2138
- M.S.IslamJ.I.L.MorisonInfluence of solar-radiation and temperature on irrigated rice grain-yield in BangladeshField Crops Research3019921328
- J.GoudriaanUsing the expolinear growth equation to analyse resource captureJ.L.MonteithResource Capture by Crops1994Nottingham University PressNottingham99110
- S.WonprasaidS.KhunthasuvonP.SittisuangS.FukaiPerformance of contrasting rice cultivars selected for rainfed lowland conditions in relation to soil fertility and water availabilityField Crops Research471996267275
- A.WhitbreadG.BlairY.KonboonR.LefroyK.NaklangManaging crop residues, fertilizers and leaf litters to improve soil C, nutrient balances, and the grain yield of rice and wheat cropping systems in Thailand and AustraliaAgriculture, Ecosystems & Environment1002003251263
- M.CooperS.RajatasereekulS.ImmarkS.FukaiJ.BasnayakeRainfed lowland rice breeding strategies for Northeast Thailand. I. Genotypic variation and genotype × environment interactions for grain yieldField Crops Research641999131151
- H.YoshidaT.HorieT.ShiraiwaA model explaining genotypic and environmental variation of rice spikelet number per unit area measured by cross-locational experiments in AsiaField Crops Research972006337343
- L.R.OldemanD.V.SeshuF.B.CadyResponse of Rice to Weather Variables, Climate and Rice1987The International Rice Research InstituteManila, Philippinespp. 159–185
- R.J.SummerfieldS.T.CollinsonR.H.EllisE.H.RobertsF.W.T.Penning de VriesPhotothermal responses of flowering in rice (Oryza sativa)Annals of Botany691992101112
- X.YinM.J.KropffR.H.EllisRice flowering in response to diurnal temperature amplitudeField Crops Research48199619
- C.S.CampbellJ.L.HeilmanK.J.McInnesL.T.WilsonJ.C.MedleyG.WuD.R.CobosSeasonal variation in radiation use efficiency of irrigated riceAgricultural and Forest Meteorology11020014554
- T.R.SinclairR.C.MuchowRadiation use efficiencyAdvances in Agronomy651999215265
- T.J.GerikW.D.RosenthalR.L.VanderlipL.J.WadeSimulating seed number in grain sorghum from increases in plant dry weightAgronomy Journal96200412221230
- M.K.van IttersumR.RabbingeConcepts in production ecology for analysis and quantification of agricultural input-output combinationsField Crops Research521997197208
- Q.JingB.A.M.BoumanH.HengsdijkH.van KeulenW.CaoExploring options to combine high yields with high nitrogen use efficiencies in irrigated rice in ChinaEuropean Journal of Agronomy262007166177
- P.BelderJ.H.J.SpiertzB.A.M.BoumanG.LuT.P.TuongNitrogen economy and water productivity of lowland rice under water-saving irrigationField Crops Research932005169185
- K.NaklangD.HarnpichitvitayaS.AmaranteL.WadeS.HaefeleInternal efficiency, nutrient uptake, and the relation to field water resources in rainfed lowland rice of northeast ThailandPlant and Soil2862006193208
- S.M.HaefeleK.NaklangD.HarnpichitvitayaS.JearakongmanE.SkulkhuP.RomyenS.PhasopaS.TabtimD.Suriya-arunrojS.KhunthasuvonD.KraisorakulP.YoungsukS.T.AmaranteL.J.WadeFactors affecting rice yield and fertilizer response in rainfed lowlands of northeast ThailandField Crops Research9820063951
- T.UmemotoY.NakamuraN.IshikuraActivity of starch synthase and the amylose content in rice endospermPhytochemistry40199516131616
- C.PerezO.B.JulianoS.P.LiboonJ.M.AlcantaraK.G.CassmanEffects of late nitrogen fertilizer application on head rice yield, protein content, and grain quality of riceCereal Chemistry731996556560
- A.K.BorrellA.L.GarsideS.FukaiD.J.ReidGrain quality of flooded rice is affected by season, nitrogen rate, and plant typeAustralian Journal of Agricultural Research50199913991408
- L.YangY.WangG.DongH.GuJ.HuangJ.ZhuH.YangG.LiuY.HanThe impact of free-air CO2 enrichment (FACE) and nitrogen supply on grain quality of riceField Crops Research1022007128140
- S.M.HowdenJ.-F.SoussanaF.N.TubielloN.ChhetriM.DunlopH.MeinkeAdapting agriculture to climate changeProceedings of the National Academy of Sciences10420071969119696