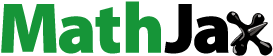
Abstract
Denitrification and leaching of nitrogen (N) from agriculture are a loss of nutrients to farmers and sources of pollution to water and air, and should therefore be minimized. In a field experiment on loamy soil, denitrification and N leaching were measured after late summer incorporation of fodder radish residues with or without paper pulp as N-immobilizing organic material. A set of relatively simple methods were used to measure and calculate denitrification and N leaching during the first two weeks after application and during the rest of the winter period. The methods were acetylene inhibition of nitrification, anion-exchange resin, the mineralization model MINIP, and inorganic-N balance calculations. Paper pulp increased N immobilization after the first day of application throughout the winter. This led to a 63–70% reduction in N losses compared with the sole fodder radish field and with the control. Denitrification was highest in the sole fodder radish treatment, at 65% of its total N losses. N leaching during the winter period was highest in the control, at 70% of its total N losses. This N was mainly liberated by mineralization of soil organic matter after ploughing in late summer. The application of paper pulp plus fodder radish did not affect sugar beet yields in the next year. The methodology for determining leaching and denitrification enabled the assessment of differences among treatments. It showed clearly that paper pulp strongly reduced N losses on this type of soil. The anion resin method that was used to measure leaching during the winter period showed clear and consistent differences between treatments, but may need additional calibration before fully relying on the absolute amounts of N leached.
1 Introduction
1.1 Nitrogen losses in the Netherlands; risks and strategies
Nitrogen (N) losses from agriculture are a source of water and air pollution and a direct loss of farming inputs. Leaching of N from agricultural soils is a major source of groundwater pollution in the Netherlands [Citation1]. The nitrate concentration in groundwater, however, cannot be attributed to a single indicator such as N surplus. De Ruijter et al. [Citation2] concluded that next to N surplus also denitrification and fertilization practices are important. Denitrification can potentially lower the risk of nitrate leaching, but is also a nutrient loss. Moreover, it can contribute to the emission of the harmful greenhouse gas N2O. European rules on water quality are translated into tight rules about allowable N application rates in the Netherlands, thus diminishing the N-fertilization space for farmers. Therefore, N losses from leaching and denitrification are not in the interest of farmers either. Lost fertilizers do not help obtaining the optimum production needed to keep the farmer's business economically sound in a competitive world.
The main strategy of EU and Dutch policy makers has been to control N pollution by forcing farmers to apply less N than they were used to before environmental issues became part of the political agenda in Europe. This strategy puts farmers in a position in which they compete against government. Alternatively, strategies to diminish N losses may be in the interest of both farmers and policy makers and may therefore lead to co-operation between farmers and policy makers.
Some N losses may hardly be avoidable in soil-grown crops, e.g., N leaching and denitrification after a heavy rain, during growth of a crop with a high N demand and low root density. N losses during fallow periods may be easier to avoid than N losses during crop growth. A well known and practised strategy to diminish nitrogen losses in autumn and winter is to grow a catch crop [Citation3]. However, an effective catch crop needs a period with sufficiently high temperatures and light intensities to germinate and grow [Citation4], but even if it grows properly, N from plant residues of a preceding crop may be lost during the initial weeks after the preceding crop's harvest.
1.2 Preventing losses by nitrogen immobilization in soil
Rather than immobilizing N in a crop, a less well known and hardly practised strategy in the Netherlands is to prevent N losses by immobilizing inorganic N in the soil. Inorganic soil-N can be temporarily immobilized by applying easily decomposable organic carbon. The microbes (with a low C:N ratio) active in the decomposition of organic material with a high C:N ratio use the inorganic N and with it immobilize temporarily the inorganic N. To be easily decomposable and to reach highest N immobilization, the organic material needs to have a low N content, and a high C:N ratio with easily decomposable organic C [Citation5]. The material needs to have a low level of lignin, which hampers decomposition of the organic C [Citation6]. High levels of polyphenols may fix proteins and nitrite and thus immobilize N in a non-microbial way [Citation7].
Several researchers [Citation8–Citation23] () have tested N immobilization of organic materials, aiming at (1) maximum N mineralization especially of mulches and cover crops in N-deficient tropical soils, (2) minimum N immobilization in potting materials in horticulture, and (3) maximum/optimum N immobilization reducing N losses to groundwater and air, especially in high-input agriculture. For our study we chose to use paper pulp, because together with straw it is abundantly available, whereas the range of immobilization capacity of woody materials seems to be much higher than of straw. Moreover, small additions of paper pulp are already used by flower-bulb farmers in the Netherlands to reduce wind erosion. So farmers are already familiar with the use of paper pulp in agriculture. Therefore, they will probably not experience problems with existing rules and laws, which often hamper the use of ‘new’ waste-materials. Paper pulp may also increase organic matter build-up in soils [Citation14], which may neutralize the fear of farmers that the soil impoverishes due to precision fertilization using inorganic fertilizers rather than organic manure.
Table 1 Literature data on organic materials (om), their application rates on dry matter (dm) basis and N-immobilizing characteristics.
1.3 Simultaneous field assessment of nitrogen leaching and denitrification
N losses in the field caused by denitrification and leaching are often not measured at the same time due to methodological difficulties. Many methods that are used to measure N leaching need to calculate point-in-time measurements of nitrate concentrations in the soil solution into sums over periods using water balances, which may be a source of considerable error when drawing up N balances [Citation24,Citation25]. Moreover, if only N leaching is measured, the resulting N management strategies promoted afterwards may reduce N leaching, but increase denitrification. On the other hand, if only denitrification is measured and managed, N leaching may increase. This is only swapping pollution from water to air or vice versa [Citation14,Citation26]. We therefore chose and modified the method of Hatch et al. [Citation27], which measures and calculates both denitrification and N leaching at the same time, in the field. Our objectives were to assess quantity and type of N losses (through denitrification and leaching) from fodder radish and the effect of paper pulp on these losses, by (1) quantifying denitrification and N leaching in the soil during the first two weeks after incorporation of fodder radish with or without paper pulp, (2) quantifying N leaching during the winter period, assuming that the major part of gaseous N losses occur shortly after incorporation of the organic material, whereas N leaching occurs more gradually [Citation14,Citation28], and (3) assessing whether the application of paper pulp has a negative effect on the growth of a succeeding crop.
2 Materials and methods
A field experiment was laid out in August/September 2005 on a level field at the experimental farm of Applied Plant Research of Wageningen University and Research Centre at Lelystad, the Netherlands (52°33′N, 5°33′E; 4 m below sea level). Annual rainfall in the area is about 750 mm, well distributed over the year.
The soil is a young marine loam, with a pHKCl of 7.5 in the plough layer and an organic matter content of 32 g kg−1. After a crop of spring barley harvested on 2 August 2004, the land remained fallow, which resulted in relatively low and homogeneous inorganic-N content in the soil as N inputs were limited to wet and dry deposition over the past years’ fallow.
2.1 Experimental design
The field experiment was of the split-plot design with two replications. Treatments (main plots) were (1) application of cut fodder radish (Raphanus sativus L.) (B), (2) application of cut fodder radish + paper pulp (BP), and (3) a control, consisting of bare fallow and no organic material applied (C). The main plots were split into two subplots, which were used consecutively for one week for measuring denitrification and N leaching.
Cut fodder radish was applied at a rate of 50 Mg ha−1 fresh material (equivalent to 6.6 Mg ha−1 dry material), containing 89 kg N ha−1 and 2.6 Mg ha−1 organic C. Paper pulp was applied at a rate of 43 Mg ha−1 moist material (equivalent to 20.5 Mg ha−1 dry material), containing 33 kg N ha−1 and 6.4 Mg ha−1 organic C. The material was incorporated mechanically into the upper 0.2 m of the soil profile one day before starting the measurements. Control plots were treated mechanically in the same way. For chemical composition of the incorporated materials see .
Table 2 Contents of C, N, cellulose, lignin, lime and heavy metals in fodder radish and paper pulp.
After winter the experiment was sown to sugar beet on 19 April 2006 at a density of 110,000 plants per ha and harvested on 25 October 2006. N-fertilization was only 60 kg N ha−1, broadcast before sowing. An area of 30 m2 was harvested from each plot, and (dry matter) yield and N content were assessed in the aboveground biomass and in the sugar beets.
2.2 Measurements
2.2.1 Methods
Denitrification and N leaching were determined over two consecutive one-week periods (23 August–8 September 2005) immediately following incorporation of the organic materials. A modified version of the method of Hatch et al. [Citation27] was used. The modifications consisted of (1) a construction as depicted in , (2) a daily addition of 25 ml acetylene (C2H2) (except on the first day when 2 × 25 ml was added, and on rainy days when 15 ml was used) resulting in a concentration of 0.01–5% acetylene in the soil air, as established in earlier tests, and (3) inserting the tubes from the surface downward and inserting the anion resin ring and gypsum disc under the outer tube via a pit next to it and a slit under it so as not to disturb the outer tube. Acetylene at a concentration of >0.01% and <5% of the soil air served to inhibit nitrification without affecting mineralization. The pvc resin rings had a diameter of 0.08 m, were 12 mm thick and contained a mixture of 22.5 g anion-exchange resin (ASB-1P, Sybron Chemicals, Birmingham, USA) and 22.5 g cation-exchange resin (C-267, Sybron Chemicals, Birmingham, USA). The gypsum discs placed under the resin rings had about the same dimensions. The exchange resin served to catch the N leached over the week of observation. The gypsum discs served to minimize N diffusion into the resin discs from below.
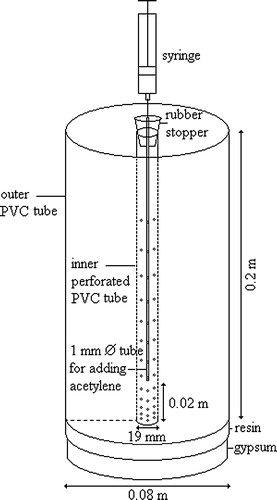
Measurements were taken in triplicate. To this end three pairs of measuring units were placed in each subplot, such that the soil was not disturbed. Only one tube of each pair received acetylene.
Leaching over the rest of the winter season was assessed using 18 mm thick pvc rings with a diameter of 0.1 m, containing 90 g anion-exchange resin (ASB-1P, Sybron Chemicals, Birmingham, USA). Three rings were placed per subplot at a depth of 0.4 m (about 0.1 m below the plough layer to minimize N diffusion from below and around the rings). The rings were placed immediately after the two weeks of measurement and remained in the soil until 14 April 2006.
2.3 Soil sampling and analysis
The soil in the measuring units was sampled twice: at the start and at the end of each week. Samples taken at the start of each week consisted of a mixture of soil taken from the hole needed to place the inner (perforated) tube (see ) and from three points directly around the outer tube. Soil samples at the end of each week were taken from within the outer tube.
NO3− and NH4+ were determined in moist soil extracted with 1 M KCl and analysed using segmented flow analysis (SFA) [NEN-5709, 2006; ISO/TS-14256-1, 2003]. The moist exchange resin rings were desorbed with 100 ml 2 M KCl, and NO3− and NH4+ were determined in the extract, using SFA.
To determine the effects of all soil-N processes on soil-N fractions in spring (28 March 2006) before sowing sugar beet, soil samples were taken to a depth of 0.3 m. The samples were extracted with 0.01 M CaCl2 and analysed for NO3− + NO2−, NH4+, total dissolved N and soluble organic C [Citation29].
2.4 Calculations and statistical analyses
Denitrification (D), nitrification (N), net mineralization (M), change in total inorganic N (m) and leaching (L) were calculated as follows:where Δ = value at the end of the week − value at the beginning of the week (=increase or decrease) [Citation27].
Analyses of variance were conducted on D, M, L and on N with 2 replicates (averages of the three measurements per replicate), for each of the two weeks separately. Using the model MINIP [Citation30], N immobilization and N mineralization of the fodder radish + paper pulp treatment were calculated at weekly intervals in the period between incorporation of the materials and harvest of next seasons’ sugar beet crop in October 2006. The model input ‘initial age’ was assessed by expert judgment to be 1.05 (years) for fodder radish and 1.10 (years) for the mixture of fodder radish and paper pulp (initial age for cellulose 1.13 (years)). Amounts of N and C added and C:N ratio were as mentioned above.
To assess denitrification losses over the winter, an inorganic-N balance was drawn up as follows:where Ni_1Apr = inorganic N measured on 23 March 2006; Ni_init = inorganic N at the start of the experiment (23 August 2005); ΔNi_minC = N mineralization of SOM during the winter, calculated from the N balance in the control treatment (C); ΔNi_MINIP = mineralization of the fodder radish (B) or fodder radish + paper pulp (BP) up to 1 April according to MINIP; Ni_leach_tot = amount of inorganic N leached over the two weeks of measurement plus the winter, as assessed by the resins described above; Ni_denit_m = denitrification measured over the first two weeks; Ni_denit_winter = denitrification over the winter (to be calculated by this balance).
Because there are three equations (for the three treatments) with four unknowns, different scenarios were considered in which Ni_denit_winter in treatment BP was assumed to be either 0 or (0.5ΔNi_minC), and in which either all or half of the measured Ni_leach_winter came from the layer 0–0.2 m (because this layer contained most N plus the added materials, but the resins during the winter period were inserted at 0.4 m depth).
3 Results
Rainfall in the first week of the denitrification measurements was 12 mm. In the second week, without precipitation, 10 mm irrigation water was applied. Average daily air temperature was 16 °C and 19 °C in the first and second week, respectively.
3.1 Nitrate, ammonium and soil-water contents
shows the average NO3− and NH4+ levels at the start (field) and at the end (tubes without C2H2) of the two test weeks. At the end of the first week (t1) total inorganic N (NO3− + NH4+) had increased compared with the start of the week (t0) in treatments C and B, and had decreased strongly in the BP treatment. At t1 there were differences between measurements within the tubes without acetylene (end of the first weeks’ series) and measurements in the open field (start of the second weeks’ series) in the tubes NH4+ contents were higher whereas in the open field NO3− contents were higher. This difference was very pronounced in the fodder radish treatment (B).
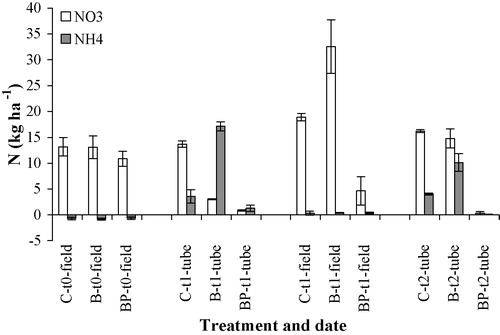
shows the average soil-water content in the open field and in the tubes of the two test weeks. The soil-water contents differed significantly between treatments at the three dates of measurement, with lowest soil-water content for C and highest soil-water content for BP (mean difference 0.01–0.02 g g−1). Moreover, at t1, soil-water content in the tubes (end of the first weeks’ series) was significantly higher (about 0.01 g g−1) than in the open field (start of the second weeks’ series).
3.2 Denitrification, N leaching, net N mineralization and nitrification
shows the calculated denitrification, N leaching, net N mineralization (gross mineralization minus immobilization) and nitrification. In the first week, denitrification was significantly higher in BP (10 kg N ha−1) than in C (2 kg N ha−1). Denitrification of B was intermediate without a statistically significant difference from either BP or C. In the second week, denitrification of B increased strongly up to 22 kg N ha−1 and denitrification of BP was strongly reduced compared with the first week, remaining at the level of the control treatment.
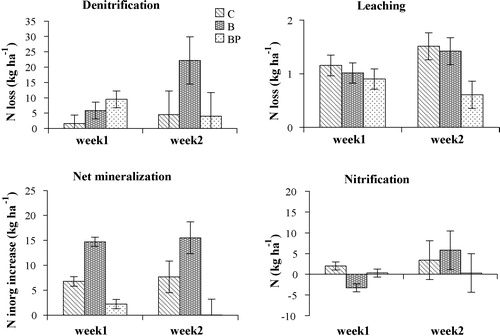
Total N leached did not exceed 3 kg N ha−1 in the first two weeks and was highest in C and lowest in BP, with statistically significant differences between BP and the two other treatments in the second week.
Net mineralization in both weeks was highest in B (around 15 kg N ha−1), and half this amount for C. Net mineralization in BP decreased from 2 kg N ha−1 in the first week to zero in the second week.
Nitrification in all treatments was less than 6 kg N ha−1 in both weeks, with even a negative value for B in the first week.
3.3 Inorganic-N balance during the winter period
presents the N balance during the winter period in the 0–0.2 m soil layer. The N balance variables were either measured (numbers) or calculated (letters) according to four scenarios in which different assumptions were used about denitrification during the winter period in BP (0 or 0.5 times background N mineralization) and about N leaching (all N leached came from the 0–0.2 m layer or only half of the total N leaching came from the 0–0.2 m layerFootnote1).
Table 3 Measured and calculated factors of the inorganic-N balance between 23 August 2005 and 1 April 2006, in four scenariosFootnotea (combinations of 2 × 2 assumptions) for all treatments.
N leaching during the winter according to the resin measurements was 54 kg ha−1 (se 7.2) for C, 30 kg N ha−1 (se 4.7) for B and 13 kg N ha−1 (se 1.6) for BP.
N mineralization of the organic amendments during winter according to MINIP was 26 kg N ha−1 for B and −47 kg N ha−1 for BP.
Background SOM-N mineralization during the winter was about 60–70 kg N ha−1 according to the N-balance scenarios 2 and 3. These scenarios, in which denitrification in BP during the winter was zero (Ni_denit_winter in BP = 0), were assumed to be most realistic, because of the strong N immobilization likely including native soil-N (see results MINIP above) and low temperatures during the winter period, hampering denitrification.
3.4 Total N losses between application and spring
presents the N losses (denitrification + leaching) during the first two weeks after incorporation of the materials, the N-leaching losses during the following winter measured by anion resins, and the denitrification losses during the following winter as calculated using the inorganic-N balance (using scenario 2 in ). This shows that N losses in the control (C) were 75 kg ha−1, which was predominantly due to leaching. N losses in treatment B (87 kg ha−1) were only slightly higher, but less due to leaching (about 40%) and more due to denitrification (about 60%). N losses were much lower for treatment BP (27 kg N ha−1) as a result of less denitrification (13.5 kg N ha−1) and less leaching (13.0 kg N ha−1).
3.5 Residual effect of organic amendments in the next cropping season
left panel shows the course of N mineralization (negative values are N immobilization) in the B and BP treatments during the year after incorporation of the organic materials, according to the MINIP model. In treatment B, N mineralization of the amendments took place continuously until late November 2006, slowing down from late November 2005 onwards, and recovering from early March 2006. One year after application, 53 kg of the added 89 kg N ha−1 should have been mineralized. In treatment BP, substantial N immobilization took place until November 2005. At that moment the difference between B and BP was more than 70 kg N ha−1. In treatment BP, N re-mineralization started mainly from April. The model results suggest that 1 year after incorporation of fodder radish plus paper pulp only 9 kg N ha−1 of the added 122 kg N ha−1 had been mineralized. The remainder was still stored in the soil organic matter (SOM) pool. The difference between the 36 kg N ha−1 still stored in SOM in treatment B and the 113 kg N ha−1 still stored in SOM in treatment BP was not visible in differences in soluble organic N () or soluble organic C (data not shown). Soluble organic N and C were similar in these two treatments (B and BP), but were higher than in the control treatment, though not statistically.

Table 4 Inorganic N contents and soluble organic N (kg ha−1) in the topsoil (0–0.3 m) at different dates during the following cropping season and total N uptake by sugar beet crop (sed = standard error of difference).
right panel combines the inorganic soil-N measurements at the start of the growing season (, inorganic N: 23 March) with the N mineralization of the organic materials for B and BP as calculated by MINIP. This shows that in treatment B about 30 kg N ha−1 became available to the crop during the growing season. At the start of the growing season (23 March 2006) soil-N was lower in BP, but through mineralization of N from fodder radish and paper pulp about 60 kg N ha−1 becomes available during the growing season. This N mineralized from the applied organic materials is hardly visible in inorganic soil-N measurements during the growing season (), but total N uptake by the sugar beet crop seemed to be somewhat higher in B and BP than in C (). Dry beet yield was similar (around 20 Mg ha−1) in all three treatments.
4 Discussion
4.1 Nitrogen losses as affected by organic amendments
During the first two weeks after fodder radish had been incorporated, denitrification was the major source of N losses. About one-third of the N applied by the fodder radish (27 kg N ha−1) was lost through denitrification. The amount of N leached in this period was only one tenth of denitrification. Incorporating paper pulp together with fodder radish reduced N losses over these two weeks by about 50%, due to immediate N immobilization. N immobilization was shown by decreased soil-N levels and a low mineralization of 2 and 0 kg N ha−1 in the first and second week, respectively. So adding paper pulp to the fodder radish led to a reduction in N losses of 15 kg N ha−1. A catch crop would not have been able to take up this amount of N over the first two weeks after planting/sowing [Citation31].
During the following winter, the reduction in N losses due to paper pulp being added to the fodder radish increased to 60–70 kg N ha−1. During this period, N leaching was unexpectedly high (54 kg N ha−1) for the control treatment (C), which had only been ploughed without incorporating organic materials. This was twice the amount of N leached in the sole fodder radish treatment and four times the amount of N leached in the fodder radish + paper pulp treatment. This N is likely to have mineralized from SOM. N mineralized from SOM likely mainly leached in the control (C), was mostly immobilized by the paper pulp in the BP treatment and partly leached and partly denitrified in the sole fodder radish treatment (B). A shift from N leaching to denitrification in the sole fodder radish treatment compared with the control is likely to have occurred because of (locally) more severe anaerobic conditions, which favour denitrification [Citation28]. Anaerobic conditions are (locally) stronger after incorporating large amounts of organic materials, due to increased microbial respiration [Citation28,Citation32]. Higher water contents also enhance anaerobiosis.
There was no residual effect of the treatments on the dry matter yield of the sugar beet crop grown in 2006. However, total N uptake appeared to be higher for the B and BP treatments (160 kg N ha−1) compared with the control (140 kg N ha−1). Only the sole fodder radish treatment had higher inorganic N at the start of the growing season. However, soluble organic-N was consistently 15–20% higher for the B and BP treatments compared with the control, probably indicating (re)mineralization of N [Citation33]. The absence of differences in soluble organic-N between B and BP might indicate that not as much N was mineralized from the fodder radish + paper pulp as expected by the MINIP calculations, probably due to a transfer of microbially immobilized N to more stable SOM-N [Citation34]. Also Devêvre and Horwáth [Citation22] noticed that incorporating straw significantly increased the amount of fertilizer-N remaining in recalcitrant forms. Other N immobilization processes than those covered by the MINIP model, which may play a role, are (1) fixation of NH4+ in interlayers of expanding lattice clay minerals, (2) chemical fixation of nitrite, ammonia or amino acids to phenolic compounds, which can remain soluble (dissolved organic-N or soluble organic-N) or can be adsorbed to soil surfaces, and (3) fixation of humic N in interlayers of expanding lattice clay minerals [Citation32,Citation35,Citation36].
According to the MINIP calculations, maximum N immobilization by the 20.5 Mg ha−1 paper pulp added to the fodder radish occurred between late October 2005 and late March 2006 and ranged from 70 to 75 kg N ha−1. This is an N immobilization of 3.4–3.6 kg N per Mg paper pulp, which is similar to the N immobilization of paper mill waste found by Vinten et al. [Citation14], and slightly higher than the N immobilization of paper mill sludge found by Aitken et al. [Citation11] or by tree clippings as found by Beauchemin et al. [Citation9]. However, it was lower than the N immobilization found for paper sludge by Simard et al. [Citation13] or the N immobilization found for cellulose and sawdust by Török et al. [Citation18] (). The considerable differences in N immobilization found in literature depend only partly on the type of material. Other factors determining the effectivity of N immobilization are (1) soil type, (2) availability of inorganic N, (3) particle size of the material, its placement and its contact surface with the mineral soil, and (4) prevalence of N immobilization to other processes involving N [Citation8,Citation20,Citation37]. Prevalence of N immobilization to other soil processes involving N may be important in the decision on the application rate of N-immobilizing material: in our experiment, a lower application rate might have increased denitrification in the first weeks due to less N immobilization and higher soil-nitrate levels, whereas a higher application rate might have caused continued N immobilization during the next cropping season. About placement of materials in soil, Baggs et al. [Citation38] mentioned that incorporating paper pulp deep into the soil increased N leaching, whereas incorporating it superficially decreased leaching.
4.2 Perspectives for farming
The high N leaching due to land preparation of a soil profile with a rather low level of inorganic N is generally overlooked in farming practice. It indicates that land preparation in autumn should be strongly discouraged in order to prevent severe N leaching during winter. On clayey soils, which cannot be tilled in spring due to low carrying capacity, additions of N-immobilizing materials before ploughing may prevent high N leaching after tillage in autumn.
Trabada-Crende and Vinten [Citation39] compared the environmental and economic effects of using paper pulp applications in existing vegetable production systems with a shift to other environmentally acceptable types of land use in a water-catchment area in England. They found that at similar levels of borehole-water quality, the vegetable + paper pulp scenario would be more economically viable than an alternative cereal–vegetable scenario.
The availability and transport of paper pulp may prevent its use on a large scale. However, applying paper pulp may be an option in situations where denitrification and N leaching are particularly high, or where the environmental conditions are particularly vulnerable, especially if long-term effects are positive. In the Netherlands, small surface applications of paper pulp are used to decrease wind erosion in flower-bulb cropping systems on sandy soils. These systems are highly susceptible to N leaching, because bulbs are harvested in July and crop residues are left in the field. Increasing the paper pulp additions and (partly) incorporating them may not only deal with wind erosion but also reduce N losses through denitrification and leaching. Applying paper pulp might also counteract the fear of farmers in the Netherlands that under the increasingly stringent nutrient management legislation, soils will deteriorate due to decreasing soil organic matter levels. Silgram and Chambers [Citation40] observed a 23% increase in soil organic matter after 10 years of straw incorporation compared with burning, whereas Aitken et al. [Citation11] reported an increase in SOM after 3.5 years of applying paper pulp.
In our experiment, the rate of paper pulp applied added less heavy metals and other potentially harmful substances than the levels allowed in annual compost applications in the Netherlands.
4.3 Methodological artifacts and their implications
The tube method that was used to measure net mineralization, nitrification, denitrification and leaching of N showed some artifacts. After inserting the tubes into the soil, the soil surface within the tubes was 2–3 cm lower than around the tubes in the open field, indicating some compaction. This may have caused more anaerobic conditions, especially in the treatments with organic amendments. Under more anaerobic conditions, NO3− may have been reduced to NH4+ [Citation32,Citation41]. This may have caused the negative nitrification in the first week in treatment B, and the higher NH4+ contents and lower NO3− contents within the tubes compared with the open field. The determination of denitrification, however, was not related to this artifact because in the equation calculating denitrification (D = M−(m + L)), ‘M’ uses NH4+ changes from tubes with C2H2, which should prevent nitrification anyway and ‘m’ uses the sum of NH4+ and NO3−, thus avoiding that an artificial shift from NO3− to NH4+ would lead to different results.
Resins accumulate N by diffusion of N from water passing around the resin rather than from water passing through the resin. This was shown by a test in which vertically placed resins without gypsum discs underneath accumulated more N during the winter period than horizontally placed resins (H.F.M. ten Berge, unpublished data). Water is likely to move mainly around the resins due to the coarser structure of the resin compared with the surrounding soil. However, resin acts as a zero sink for nitrate, and N diffusion from the soil solution towards the resins occurs. Whether our resins overestimated or underestimated N leaching depends on whether or not the rate of N diffusion into the resin could just exhaust the water moving around the resins. A rough estimate of water movement and N diffusion in our type of soil showed that rates were of a similar magnitude. Therefore, the absolute amount of N leaching measured may have been somewhat over- or underestimated. However, this did not change the differences between treatments.
4.4 Conclusions
Adding paper pulp led to a strong decrease in denitrification and N leaching from the fodder radish and additionally in a very strong decrease in N leaching from the N released from SOM after ploughing in early autumn.
A problem with using paper pulp for N immobilization at farm level may be to determine the appropriate application rate: sufficiently high to prevent high levels of denitrification after application, without being too high and causing extended N immobilization in the next cropping season.
The methods used in this study were able to supply interesting insight into the type and relative size of N leaching and denitrification in the field, as a result of application of organic amendments and ploughing.
Notes
1 Because during winter, resins had been placed at a depth of about 0.4 m under the plough layer to minimize N diffusion from around the resins into the resins.
References
- D.FratersL.J.M.BoumansG.van DrechtT.de HaanW.D.de HoopNitrogen monitoring in groundwater in the sandy regions of the NetherlandsEnvironmental Pollution1021998479485
- F.J.de RuijterL.J.M.BoumansA.L.SmitM.van den BergNitrate in upper groundwater on farms under tillage as affected by fertilizer use, soil type and groundwater tableNutrient Cycling in Agroecosystems772007155167
- A.M. van Dam, 2006. Understanding the reduction of nitrogen leaching by catch crops. PhD Thesis, Wageningen University, Wageningen.
- J.LehmannH.U.BrinerE.RosenbergCatch crops—what can and can’t you do?Landwirtschaft Schweiz41991151158
- S.GunnarssonH.MarstorpCarbohydrate composition of plant materials determines N mineralizationNutrient Cycling in Agroecosystems622002175183
- V.MeentemeyerMacroclimate and lignin control of litter decompositionEcology631978405472
- C.A.PalmP.A.SanchezNitrogen release from the leaves of some tropical legumes as affected by their lignin and polyphenolic contentsSoil Biology and Biochemistry2319918388
- B.T.ChristensenBarley straw decomposition under field conditions: effect of placement and initial nitrogen content on weight loss and nitrogen dynamicsSoil Biology and Biochemistry181986523529
- S.BeaucheminA.N’dayegamiyeM.R.LaverdièreEffects of fresh and humified wood residues on potato yields and N availability in a sandy soilCanadian Journal of Soil Science701990555564
- G.TianB.T.KangL.BrussaardBiological effects of plant residues with contrasting chemical compositions under humid tropical conditions—decomposition and nutrient releaseSoil Biology and Biochemistry24199210511060
- M.N.AitkenB.EvansJ.G.LewisEffect of applying paper mill sludge to arable land on soil fertility and crop yieldsSoil Use and Management141998215222
- T.MuellerL.S.JensenN.E.NielsenJ.MagidTurnover of carbon and nitrogen in a sandy loam soil following incorporation of chopped maize plants, barley straw and blue grass in the fieldSoil Biology and Biochemistry301998561571
- R.R.SimardR.BaziramakengaS.YelleJ.CoulombeEffects of de-inking paper sludges on soil properties and crop yieldsCanadian Journal of Soil Science781998689697
- A.J.A.VintenR.DaviesK.CastleE.M.BaggsControl of nitrate leaching from a nitrate vulnerable zone using paper mill wasteSoil Use and Management1419984451
- P.P.MotavalliH.DiscekiciUilization of waste office paper to reduce nitrate leaching into the Northern Guam aquiferBiology and Fertility of Soils312000478483
- P.NogueraM.AbadV.NogueraCoconut coir waste, a new and viable ecologically-friendly peat substituteActa Horticulturae5172000279286
- W.D.SakalaG.CadischK.E.GillerInteractions between residues of maize and pigeonpea and mineral fertilizers during decomposition and N mineralizationSoil Biology and Biochemistry322000679688
- K.TörökT.Szili-KovácsM.HalssyZs.HayekM.W.PaschkeL.J.WardellImmobilization of soil nitrogen as a possible method for the restoration of sandy grasslandApplied Vegetation Science32000714
- P.VityakonS.MeepechG.CadischB.ToomsanSoil organic matter and nitrogen transformation mediated by plant residues of different qualities in sandy acid upland and paddy soilsNetherlands Journal of Agricultural Science4820007590
- P.AmbusE.S.JensenCrop residue management strategies to reduce N-losses—interaction with crop N supplyCommunications in Soil Science and Plant Analysis322001981996
- A.-M.De CockborneL.BrucklerG.SevenierM.GascNutrient leaching from soil amended with apple wasteAgronomie212001215226
- O.C.DevêvreW.R.HorwáthStabilization of fertilizer nitrogen-15 into humic substances in aerobic vs waterlogged soil following straw incorporationSoil Science Society of America Journal652001499510
- K.KumarC.J.RosenS.C.GuptaKinetics of nitrogen mineralization in soils amended with sugar beet processing by-productsCommunications in Soil Science and Plant Analysis33200236353651
- T.M.AddiscottMeasurement of nitrate leaching: a review of methodsR.CalvetNitrates, Agriculture, Water1990INRA editionsParis157168
- C.RamosM.KuckeA review of methods for nitrate leaching measurementActa Horticulturae5632001259266
- B.ChambersO.OenemaPollution problems: mitigation or are we swapping one form of pollution for anotherD.J.HatchD.R.ChadwickS.C.JarvisJ.A.RokerControlling Nitrogen Flows and Losses.2004Wageningen Academic PublishersWageningen606608
- D.J.HatchS.C.JarvisR.J.ParkinsonConcurrent measurements of net mineralization, nitrification, denitrification and leaching from field incubated soil coresBiology and Fertility of Soils261998323330
- E.M.BaggsR.M.ReesK.A.SmithA.J.A.VintenNitrous oxide emission from soils after incorporating crop residuesSoil Use and Management1620008287
- V.J.G.HoubaE.J.M.TemminghoffG.A.GaihorstW.van VarkSoil analysis procedures using 0. 01 M calcium chloride as extraction reagentCommunications in Soil Science and Plant Analysis319&10200012991396
- B.H.JansenA simple method for calculating decomposition of ‘young’ soil organic carbonPlant and Soil761984297304
- C.FellerM.FinkA.MayncP.J.PascholdH.C.ScharpfJ.SchlagheckenK.StrohmeyerU.WeierJ.ZieglerDüngung im FreilandgemüsebauGartenbauliche Berichte42001196 pp
- E.A.DavidsonJ.ChoroverD.B.DailA mechanism of abiotic immobilization of nitrate in forest ecosystems: the ferrous wheel hypothesisGlobal Change Biology92003228236
- M.M.BreglianiE.J.M.TemminghoffW.H.van RiemsdijkE.S.HaggiNitrogen fractions in arable soils in relation to nitrogen mineralization and plant uptakeCommunications in Soil Science and Plant Analysis37200615711586
- R.M.SjöbergT.PerssonTurnover of carbon and nitrogen in coniferous forest soils of different N-status and under different 15NH4-N application rateEnvironmental Pollution1021998385393
- D.W.JohnsonW.ChengI.C.BurkeBiotic and abiotic nitrogen retention in a variery of forest soilsSoil Science Society of America Journal64200015031514
- J.KayeJ.BarrettI.BurkeStable nitrogen and carbon pools in grassland soils of variable texture and carbon contentEcosystems52002461471
- E.D.VanceF.S.ChapinSubstrate limitations to microbial activity in taiga forest floorsSoil Biology and Biochemistry332001173188
- E.M.BaggsR.M.ReesK.CastleA.ScottK.A.SmithA.J.A.VintenNitrous oxide release from soils receiving N-rich crop residues and paper mill sludge in eastern Scotland. AgricultureEcosystems and Environment902002109123
- F.Trabada-CrendeA.J.A.VintenAssessing the effects of land management and catchment hydrology on well water quality in a designed nitrate vulnerable zoneAgricultural Systems571998523540
- M.SilgramB.J.ChambersEffects of long-term straw management and fertilizer nitrogen additions on soil nitrogen supply and crop yields at two sites in eastern EnglandJournal of Agricultural Science1392002115127
- L.SchipperM.Vojvodič-VukovičNitrate removal from groundwater using a denitrification wall amended with sawdust: field trialJournal of Environmental Quality271998664668