Highlights
• | We studied how different knowledge sources were combined by farmers re-designing cropping systems. | ||||
• | Fundamental knowledge was useful to the re-design process when articulated to farmers’ actions. | ||||
• | 4 patterns linking fundamental knowledge to farmers’ action were identified. | ||||
• | A situated contextualisation process generates new knowledge about a farmer’s own agro-ecosystem. | ||||
• | The causality within systemic understanding built by farmers is generated in a situated process. |
Abstract
When they re-design their cropping systems to move towards agroecology, farmers implement practices that involve biological processes. Such practices have been qualified as knowledge-intensive, as they involve the renewal of agronomic principles and numerous interactions between the systems’ components and their regulation. Several studies recognize the value of discussing knowledge on systems’ functioning and component properties with farmers, in relation to technical change processes. This paper investigates some processes of coordination of fundamental and generic knowledge on biological processes, on the situated knowledge that farmers may use when introducing technical changes in their own cropping system, and on the integrated approach to agroecological processes. We perform an inductive inquiry, in the framework of an iterative and instrumental analysis of case studies. We chose five cases of different step-by-step cropping system re-design situations. Through our crosscutting analysis, we highlight the fundamental knowledge on biological objects that the farmers mobilized, and we describe some aspects of the processes involved in its contextualization. In particular, we describe four patterns of connection between fundamental knowledge and farmers’ actions, and distinguish three main reformulations of fundamental knowledge that participate in contextualizing it. These involve reinterpretation of individual experiences and identification of the effects of action on the situated biological processes. We conclude on research orientations for considering expert knowledge not as a specific content to integrate, but as a situated way of knowing that should be acknowledged in its processes.
1 Introduction
Re-designing cropping systems to move towards agroecology leads farmers to rely increasingly on biological processes and endogenous resources, and far less on external inputs [1–3Citation[1]Citation[2]Citation[3]]. This has several implications for the application of agricultural practices. First, farmers might have to implement practices corresponding to new agronomic approaches (such as maintaining a canopy for most of the year to cover the soil, trying to control weeds, limiting leaching and possibly increasing nitrogen fixation in the case of legumes). Thus, they may face situations in which they have little experience to guide their decisions about appropriate action. Second, managing such biological processes is made harder by the variability of their functioning according to environment-specific pedo-climatic conditions, and by the numerous and largely under-explored interactions (for example, maintaining a cover crop may lead to an increase in the slug population). This increases the uncertainty of the targeted effects or leads to unintended impacts. In view of these specificities, some authors have described the related practices as “knowledge-intensive practices” [Citation4,Citation5]. This assumes the acute need for new knowledge to apply these, particularly because they involve “the adoption of technology that requires a high level of management skills, with an emphasis on observation, monitoring and judgement” [Citation4].
Agronomists have developed three main strategies to fulfil this need. First, they have made more intensive use of the knowledge developed by farmers. It has been recognized that farmers rely on both scientific and local knowledge [Citation4,Citation6]. It has also been shown that both sources of knowledge are necessary for agronomists, either to broaden agronomic knowledge, or to design and assess agro-ecological cropping systems [e.g. 7–10Citation[7]Citation[8]Citation[9]Citation[10]]. In particular, there is an emphasis on the tacit knowledge that farmers acquire through acting in their own situation, called “experiential knowledge” [Citation11,Citation12], largely based on know-how. Second, agronomists have carried out experiments with innovative crop systems to quantify the effects of new combinations of practices enhancing biological processes, emphasizing the scope for learning [13–15Citation[13]Citation[14]Citation[15]]. Third, and this is probably the predominant strategy, agronomists have developed integrated and complex models to describe the numerous interactions within a cropping system e.g. [16–20Citation[16]Citation[17]Citation[18]Citation[19]Citation[20]]. By gathering the scientific knowledge available on soil-crop mechanisms, these models are designed to support the ex-ante evaluation of farming systems not yet applied on farms e.g. [Citation21]. The value of these models is thus argued to lie in their capacity to extensively take into account feedback loops and the unintended consequences of actions such as the quantification of water and nitrogen needs of wheat at spring when sown densely and early, which have consequences on fertilization and potential water stress induced; or the addition of new weed seeds in the soil seed bank when weed plants reach maturity, leading to harmful weed infestations in the following crops, [Citation21]. With these models, it is also possible to predict long-term trends in the system, such as soil nitrogen and carbon content dynamics under various management practices [Citation22], which are not easy to measure. The use of such quantitative and integrative models has been argued to provide helpful support to change practices e.g. [Citation23,Citation24]. However, many authors have shown that models were of little help for designing new practices, as summarized by Prost et al. [Citation25]. Moreover, the interactions between crops and practices that models simulate mostly concern the amounts of abiotic growing factors (e.g. water, nitrogen). Models rarely take into account biotic processes, while these strongly impact low-input systems (e.g. those linked to diseases, pests, soil biological activity). As a result, these integrated models may lack contextualization variables to be used successfully to design locally-adapted crop systems.
These limitations of models highlight the issue of the use of scientific knowledge in re-design situations: how can farmers mobilize general scientific knowledge in a situated action process contending with systemic interactions between biological processes? The effectiveness of knowledge-sharing between agronomists and farmers has been shown to vary, based on agronomists’ behaviour and social skills [Citation4,Citation26]. Yet, as these studies focus on social dynamics and actors’ behaviours, they provide little information on the actual content of the exchanges, and the processes of their legitimation and organization for action. Furthermore, the hybridization of scientific and local knowledge is sometimes considered difficult and partly impossible because of their differing aims regarding agrosystems: farmers’ objective is to manage ecosystems (for a crop or practice to yield satisfying results in a farmer’s situation), and scientists’ aim is to understand them (i.e. they need to know why and how something works) e.g. [Citation27,Citation28]. Based on these distinct aims, scientists have developed numerous decision support systems, as means to transfer their knowledge to farmers, with the aim of helping farmers make the right choices of practices based on their constraints. In so doing, scientists consider that farmers do not need to understand the functioning of their agrosystem to manage it and they encapsulate scientific knowledge in a usable tool. However, re-designing a cropping system does not just mean managing it, and the validity of this assumption in the context of agroecological transition is questionable. Farmers do not work with a given stable system; they gradually transform an agroecosystem while acting on productive resources, removing, adding or modifying some of its components. Furthermore, in some cases, action research has highlighted that farmers can become interested in very fundamental approaches to some parts of the system. For instance, Jordan et al. [Citation29] mentioned the use of biological knowledge on weed species as an important event in a change process.
Consequently, when the re-design of a cropping system involves biological processes, this seems to require a combination of scientific general knowledge on the corresponding system, the situated knowledge farmers acquire or develop, and an integrated approach to the cropping systems. The core focus of this article relates to this combination: how do farmers re-designing their cropping system mobilize general scientific knowledge in their particular situation? How is this knowledge contextualized? What do such processes tell agronomists seeking to provide relevant resources for re-designing cropping systems? We answer these questions by examining various cropping system re-design situations through an inductive case-study analysis. All these situations share the common feature of mobilizing specific scientific knowledge. In the next section, we briefly present the methods we used in the different cases for data collection. We then describe the five case studies. In the results section, we present four crosscutting findings.
2 Method
We selected five situations of technical change in step-by-step re-design processes, as characterized by Meynard et al. [Citation30]. Step-by-step re-design is characterized by an initial diagnosis of the practices and state of the system, followed by a range of techniques being proposed, chosen, implemented, monitored and adapted, resulting in the system experiencing new states, as well as leading to the assessment of various performances in order to start a new design loop. The five case studies concerned the implementation of new practices by farmers, in line with certain agroecological principles, as described in Wezel et al. [Citation31]. The changes were aimed at various goals (): implementing integrated crop management to reduce pesticide use (Cases 2 and 5), diversifying the cultural strategies to reduce weed pressure along the crop sequence (Case 1), and changing soil tillage to improve the soil structure and fertility (Cases 3 and 4).
Table 1 Presentation of the case-studies.
We investigated these cases through a combination of active and passive participation, and through comprehensive semi-structured interviews. The timescales of the collected data varied from one-day meetings to 5–7 year projects with regular experiments and meetings (). The number of people concerned by each case and their professions also varied from one individual farmer to a group involving several farmers, advisors and facilitators (). On the one hand, we observed (Cases 1 and 2) or interviewed (Case 5) groups of farmers in different situations considered as important stages in the step-by-step design process [Citation32]: a system experiment visit, and a one-day design workshop (). On the other hand, we carried out individual semi-structured interviews with farmers, either participating in a development group (Case 5), or not (Cases 3 and 4). These interviews were organized in the same way. After a quick description of the farming system, we first identified, with the interviewee, the main problems and the main technical changes that had been introduced. We then focused on the implementation of one specific technical change, and asked the farmer about the information sources mobilized, the successive steps taken, the observations made, and finally the changes made and kept. Finally, we opened the interview to other technical changes or aspects of the cropping system.
Our inquiry was largely inductive, as we did not base our analysis on a specific hypothesis concerning the way the farmers may mobilize scientific knowledge. We made instrumental use of the cases [Citation33]: in each case, we closely observed the moments when scientific and fundamental knowledge was mobilized, and progressively built qualitative criteria for their analysis (summed up in ), based on a grounded theory approach [Citation34]. Cases were thus used in their complementarity to inform the iteratively built criteria of analysis, as no one was fully informative on all aspects. The specific hypotheses formulated from the in-depth study of one case were tested on the other cases, and so on, back and forth. Based on the identification of the specific knowledge mobilized in each case, we tracked its transformation and its use until the implementation or design of a new practice. We identified key elements in the chronology of the event, and focused on some sticking points and steps or events through which these were overcome. We then identified the knowledge shared and used by farmers in each of these steps. We specifically focused on the knowledge that made it possible to continue with the different technical changes, and thereby unlocked the building of new action strategies. In each case, we used full transcripts of the interviews or meetings. Finally, we performed a transversal analysis of the different cases in order to highlight the main patterns involving the following processes: how specific knowledge is asserted and discussed; how generic knowledge is used in a specific context or, conversely, how localized experiences are discussed and shared in general terms; and how it allows the farmers to choose new practices or strategies they intend to implement. Five common findings, complementarily supported by various amounts of data from each case, are specifically developed. They may help agronomists to recognize or generate knowledge mobilization processes.
Table 2 Case-studies specificities according to the knowledge and experiences exchanges, the agronomic problematics, the technical strategies built.
3 Case studies
3.1 Organic farmers meeting about perennial weed control
The meeting focused on the management of perennial weeds, particularly thistle, identified as a common problematic species on the group’s farms. It started with a presentation by a facilitator on biological and physiological aspects of thistle, drawing on scientific papers, agronomic press, and expert knowledge from experimenters (, line 2). During this presentation, although the techniques were not mentioned on the slides, farmers’ comments directly linked the information given with possible changes in their actions. The same facilitator then presented two curative strategies: exhaustion and extraction (, line 2). The size of root fragments to support each strategy differs (long for extraction, and short for exhaustion) based on the soil management tools used. The results from different experiments comparing various soil tillage tools quickly prompted discussions about organizational feasibility (workload, equipment, energy use), but did not lead to the emergence of new management strategies. After this first part of the meeting, farmers discussed their own experiences, but without reaching a shared conclusion, mostly underlining the specificities of situations (e.g. the possibility of having long dry periods for an efficient extraction strategy; density and age of thistle’s spots). In the afternoon, the farmers were asked to each make propositions for a specific case. They started with opposing points of view, without consensus on the results of the techniques proposed (competitive effect of alfalfa or a lentil-triticale mixture; the use of specific machines adapted from other farmers’ experiences, e.g. the “Wenz method”). A real strategy began to emerge only when the discussion returned to the key aspect of the dynamics of thistle “reserves”: how they are built at different development stages (soil nutrient absorption, leaves’ metabolism) when, at what rate, and what processes most affect them (root fragmentation, plant growth, and vegetative part destruction). These aspects were related first to possible observations of the processes (observed plant growth and vigour, leaves’ production and senescence, roots’ multiplication), and second to the interpreted effects of practices (mowing, stubble ploughing to favour weeds’ germination) on this dynamics of “reserves”. This involved re-specifying the key moments of the dynamics, and the detailed processes of the constitution of reserves (minimum at harvest? At the end of summer? Reserves increase when a plant grows or when flowers are cut? Depth of soil at which emergence from a rhizome is most probable?). The participants identified a specific indicator of plant development stages which was directly linked to the reserves’ dynamics: the 6–8 leaves stage. Prior to this, the plant’s reserves decrease, whereas after they increase again. Only then were two different practice strategies to test proposed (, line 5).
3.2 System experiment visit with a group of farmers
During a visit to a cropping system trial, the main experimenter presented the different cropping systems implemented and their re-design objectives. One of the variables measured in the trial, which the farmers asked the most about, was the amount and diversity of worms in the different plots. The farmers commented that the simple fact of assessing the presence of worms was of value, but that they could not relate it to their practices. The measurements in the trial showed long-term trends that the experimenter linked to the past management of the plots (e.g. a plot with a historical background involving no ploughing practices differed from others). Repeated soil tillage with tine or disc tools which negatively affected worm populations was also discussed. Surprisingly, the farmers’ discussions focused primarily on the main families of worm species, as well as their most prominent life traits, about which they asked the experimenter for further details. For instance, they related the classical movements of the different species within soil to the effect of their different tools for soil tillage (, line 5). However they lacked more specific knowledge about the dynamics of development and regeneration at population level to anticipate the consequences of their actions on a scale of several years. One farmer enriched the discussion with observations from his own farm: an apparent increase in the worm population was noted, whereas he mentioned very few changes in his practices. The other farmers led him to specify that stubble ploughing was much less frequent, validating the established link between this action and the development of certain species.
3.3 Farmer's implementation of stubble ploughing and cover crops in a minimum-tillage system
This farmer participated in an eight-year project with a R&D organization to develop integrated crop management using less pesticide. At the same time, he changed his cropping system by removing all ploughing practices. At first, his knowledge about the techniques associated with no-ploughing strategies was restricted to the types of machines one can use, and the problems encountered which lead to removing ploughing (e.g. the energy cost of ploughing, hydromorphic soils). Rapidly, he had to use more pesticides. In order to continue not to plough while decreasing herbicide use, he tried to adapt the techniques used for soil preparation and covering between crops. He implemented stubble ploughing after crop harvests to bury crop residues and manage weeds. However this had varying effects and the following wheat crop showed a weaker growth dynamic. He obtained various references by comparing the number and date of applications with colleagues, but this still did not give him guidance for the specific adjustment of the practice. He began to resolve this issue when a scientist studying carabid species presented basic elements on carabids’ biology (how different species move in various environments along their lifetime and seasons, what their resources and habitat needs are, what the main mortality factors are), and namely the depth of soil at which they reproduce and how soil movements affect their reproduction. He deduced that soil tilling deeper than 10 cm prevented the development of a carabid population by disrupting its habitat, thus favouring the growth of slug populations (although no specific knowledge about the efficacy of carabids predation on slugs was discussed). With the help of an expert from a technical institute, he then confirmed that 10 cm was a sufficient soil tilling depth to grow beetroots: that is, he considered other possible actions in his own situation, handling interactions with other practices (i.e. the presence of beetroot crops in the succession). He analysed and reinterpreted the results concerning the use of stubble ploughing and the corresponding action of the machine with colleagues, comparing their respective experiences to confirm some of the technique’s effects (e.g. the soil aspect to be expected right behind the machine, the machine’s adjustment).
3.4 Farmer's implementation of a minimum-tillage cropping system
In 1998, this farmer started to look for strategies to reduce his workload. He also struggled with soil humidity in some fields. Convinced by an article based on a farmer’s experience that presented the effect of no-ploughing strategies on the soil’s bearing capacity (, line 1), he started to apply simplified cultural techniques in one difficult field (i.e. too wet in the spring). After seven years of not ploughing the plot he started to notice the expected improvements in soil bearing capacity, but a company harvesting the hemp on the plot entered after a 50 mm rainfall, which resulted in a compacted soil structure and caused him to plough again this year. He then joined an association dedicated to no ploughing and took part in various visits and training courses. Describing these, he mainly emphasized two starting points for his renewed agronomic reasoning. First, he stressed the determining role of knowledge about soil biology (, line 2). This led him to change his own view of the soil, seeing it as a compartment of the system which fulfils nutritional functions, and not only as a physical support, and reformulating his knowledge on the role of bacteria in transforming and supplying nutrients (for instance the role of denitrification and nitrification processes). He associated the soil’s nutrition ability with indicators (e.g. clod toughness, the depth of roots’ soil exploration, humus content). Second, the specialist specified that the diversity of bacteria populations is linked to the diversity of plants growing in the soil (though without specifying which diversity of plants). He applied this knowledge to his situation: he evaluated that the crop diversity in his system (4 different crops with similar rooting depth) was too low and decided to introduce a pea crop (combining the functions of crop diversity with the nitrogen management issue), and to diversify the species used as cover-crops with mixtures of species having different root systems to maximize soil exploration. Bacterial activity was also central to the concept that guided the way he interpreted the functioning of the interactions between plants and the soil and therefore between different practices, i.e. the continuity of soil bacterial activity across cash crops by sowing cover crops mixtures: “Well I keep the continuity of the main crop if you will, and apply it to the other crop.”
3.5 Co-development of weed management strategies in low-input cropping systems
After the first five years of an R&D project dedicated to the reduction of pesticide use, fungicide and insecticide uses had reduced greatly see Ref. [Citation35], but the use of herbicides had not. The project therefore began to focus on weed control, which differed from diseases in terms of the timescales that needed to be taken into account. The project actors first visited a long-term system experiment [Citation13] dedicated to decreasing herbicide use. They found the results presented unconvincing, due to the poor economic results of the systems tested, and according to them, these offered no clues as to possible technical changes to implement. Among the various techniques tested in this experiment, they nevertheless identified mechanical weeding as a possible weed control technique that they had not yet explored. For instance, chain harrows were tested on barley. The results varied widely from one situation to another: on chalky soils, they slowed down the cereal’s growth and allowed weeds to grow back even more than before the harrowing, whereas in loamy soils, it favoured the barley’s growth, with satisfying weeding results. An agronomist who had participated in designing the cropping systems tested then presented different elements of the knowledge underlying his work: the annual rate of decline, the emergence time, and the germination depth of the main weed species. Once they started to mobilize these aspects, they were better able to anticipate the results of specific actions such as applying stubble ploughing, or various frequencies of soil tillage, and could modulate them to be more efficient. As all the farmers involved used different techniques, the meetings were an opportunity for them to share their experiences, basing their interpretations on weed biology. For instance, they shared experiences about goosefoot infestation, and compared their weed pressure according to the ploughing frequency, which was linked to the annual decay rate: they advised each other not to plough after a high infestation rate because of the longevity of the seeds buried. A farmer decided to introduce a new sunflower crop, basing his reasoning on his most problematic weeds’ time of emergence, which differs from that of sunflowers (, line 5).
4 Crosscutting analysis: conditions for the mobilization of fundamental knowledge and the process of systemic contextualization
Through the cross-comparison of the case studies looking at the various forms of knowledge mobilization involved, we identified four main common results, closely interconnected. The order in which we present them makes it possible to gradually understand how specific elements of generic knowledge can be linked to a particular cropping system.
4.1 Focused, partial, fundamental, often descriptive knowledge is used and may unlock situations of change
The comparison of our case studies shows that the knowledge which appeared useful for unlocking processes of change was very specific, rather than involving the whole system in an integrated way. In fact, whereas the problems the farmers faced were highly systemic (, line 1), the knowledge that allowed them to move forward in the technical changes was very fragmentary and selective. In all cases, the knowledge concerned only some components of a system () and mainly the biology and dynamics of biological objects: particular species (thistle in Case 1; cover-crop species in Case 3; various weed species in Case 6), groups of species (worms in Case 2; carabids in Case 3), or larger groups of micro- or macro-organisms occupying the same ecological niche (soil bacterial populations in Case 4). Biological objects are to be opposed to technical objects such as physicochemical objects (the water or nitrogen pools and fluxes within the soil) or machines. These biological objects are generally not directly and intentionally manipulated by the farmers, but they are always involved in natural processes that might interact with cash crops’ growth and productivity. Also, they can be influenced by the farmers via cultural practices. Furthermore, the knowledge used was fundamental, in the sense that it described a biological or physiological process (such as the dynamics of thistle reserves’ accumulation and depletion throughout the year, or the cycle of development of a plant disease, , line 2). We call it descriptive as it focuses on the intrinsic mechanisms of the object, at its scale. Thus, this fundamental knowledge is to be opposed to more operational knowledge, for example the effectiveness of different soil tillage tools to decrease the thistle population. It concerned neither systemic interactions nor regulation. The analytical fundamental knowledge we identified was thus mostly qualitative.
In several case studies, this particular knowledge was proposed by a specialist. This was expressly mentioned in Case 3 concerning the carabid species’ biology (an entomologist specialized in carabid species), but also in Case 4 (an agronomist specialized in rhizosphere studies), and in Case 6 around weed species’ life traits (a weed researcher). These specialists belonged either to research institutes or to national technical institutes. The legitimacy of such actors in the eyes of the farmers lays in their ability to bring together a host of bits and pieces of knowledge that may also be available from other sources (websites for example) but were never organized in a synthetic form.
Whereas a large proportion of studies on knowledge exchange between scientists and practitioners discuss the relevance, accessibility and legitimacy of knowledge [Citation26], our analysis focuses on the intertwining of strands of knowledge contents and of knowledge production and legitimation processes. Although we do not deny the importance of the social aspects of knowledge sharing, we suggest that these might be determined by the technical aspects addressed by the knowledge. The prevalence of partial knowledge on a limited part of the system components might seem contradictory with the necessity to anticipate the systemic feedback effects and unintended consequences of actions. However, in the following sections we show how such knowledge content issue is related to processes of causal interpretation and contextualisation.
4.2 Farmers use the knowledge they can link to their own action
The cross-comparison of our cases highlights that the knowledge mobilized was that which farmers could use to steer their own actions. In fact, among all the functional aspects of the biological objects that farmers might manipulate, they considered as useful those for which they could establish a relationship between their actions (already implemented or potential) and the response of the objects. Through our cross-cutting analysis we identified four different types of relationships or patterns that participate in a process of contextualization.
First pattern: knowledge about a biological object can relate to an action that farmers already performed and manage, the effect of which is also partly known by the farmer. To understand the effects on the new object of an action already performed, further knowledge on this object is required (, Pattern 1). For instance, in Case 1, farmers asked for specific details about the depth at which root regrowth mechanisms occur, to be able to relate this to the depth of their soil ploughing. This gave them a better understanding of the various effects of actions on roots’ biology and physiology. In Case 4, knowledge about the depth of roots’ soil exploration was related to the no-ploughing strategy, along with knowledge about roots’ exploration dynamics and the possible causes of rooting reduction, to understand soil profiles and compaction. This pattern can be considered as a first step towards situating knowledge: farmers try to identify the conditions of action in which the effects targeted will be obtained or not, depending on the knowledge acquired on the biological object.
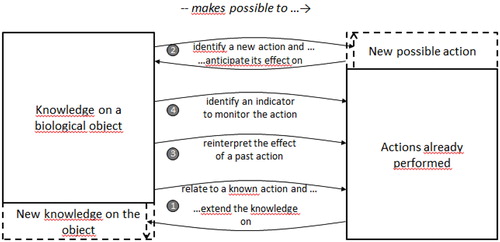
Second pattern: farmers can use fundamental knowledge on biological objects when it allows them to anticipate the effect of a new action that they have never performed (, Pattern 2). In Case 1, for instance, they asked for knowledge on thistle roots’ biology in connection with the different tools used for soil tillage. In fact, since only specific parts of the roots can regrow after being cut, they tried to select the appropriate tool for soil tillage based on the depth and width of scalping. In Case 3, the farmer built a new complete soil management strategy starting with the constraint of a 5 to 10 cm depth limit for soil tillage, so as to keep the disruption of carabids to a minimum and thus reduce the occurrence of slug attacks.
Third pattern: fundamental knowledge can be used to reinterpret previously observed effects or consequences of an action (, Pattern 3). In Case 1, the 5% spread of thistle through seeds explained the low effectiveness of topping: avoiding flowering and seed maturity blocks a low proportion of multiplication capacity, which is mostly supported by the root system. Farmers also associated past observations of thistle pressure increase within fields after repeated cutting and mechanical weeding with the regrowth mechanism of suckers remaining on short pieces of roots which may result from these cultural practices. The dynamics of thistle roots’ reserves also offered a greater understanding of a known adverse effect of alfalfa on the weed: “Then a thistle in alfalfa grows too. It rises more, it produces more vegetative material so it draws more from its reserves” (a farmer).
Fourth pattern: fundamental knowledge can guide action by enabling farmers to identify an indicator to monitor their action (, Pattern 4). In Case 1, the thistle’s development stage of 6–8 leaves, stage at which the plant’s reserves are at their lowest, was identified as an indicator for triggering the cutting, so as to efficiently weaken the weed. In Case 4, the basics of soil biology were directly related to the possible observations that farmers might use to anticipate potential biological activity in their soil, or the dynamics of disease spread, and to trigger management actions.
These four different patterns of relationships between fundamental knowledge and the farmer’s action highlight a necessary condition: it must be possible to tackle the bio-physical phenomenon with a delimited and identified action that a farmer may perform. Fundamental knowledge thus has to relate to mechanisms or biological processes on scales that farmers could directly address through delimited actions, andthis seems to be a condition for it to be interpreted in relation to specific contexts. For instance, the examples provided above referred to actions which were specific elements within crop management programs.
These patterns also suggest particularities in the mobilization of knowledge to design new actions in a cropping system. They highlight the fact that farmers gradually organize knowledge on the functioning of limited parts of the system, and do not embrace the whole system at once. Considering the functioning of a limited part of the system makes it possible to relate it to specific actions, while the assessment of a global functioning would relate to integrated actions (e.g. a complete crop management itinerary), involving a whole set of causal relations that one may not be able to grasp. In that sense, our findings converge with those of previous ergonomic studies [Citation36,Citation37], which suggest that actors tackle anticipated events and plans based on a known set of actions, that is, that knowledge on the systems’ processes is organized according to known action. Nevertheless, these studies considered situations where usual actions were to be applied. In our case, the design of a technical change may explain that we observed such organization of knowledge in both directions: new knowledge also led to the organization of new actions. Building an understanding of the functioning of parts of the system results in iterative loops between knowledge on the biological components and action.
However, this continuous iterative process still focuses on isolated actions or objects, and as such does not fully explain how the fundamental knowledge is contextualized in reference to a situation. According to Dewey [Citation38], we never experience or make judgements about isolated objects or acts, but only in connexion with a contextual whole, which is what is called situation. The connexion to the situation isexplored in the following sections.
4.3 Fundamental knowledge supports the reformulation of individual experiences and makes them useful to others
A situation is structured by the actor who redefines it throughout the action [Citation39]. Addressing the issue of how knowledge was related to specific situations thus requires us to consider how farmers actively defined what their situation of action was. Lave [Citation40] proposed to distinguish the arena, i.e. the material and institutional components of the situation, from the setting, i.e. the interactive coupling between the actor and this arena. Farmers readily shared experiences from their own situations. However, as these were situated experiences corresponding to different settings, they were not easily extrapolated through comparisons of arenas (e.g. types of soils and microclimates). In this section, we show that comparing individual experiences with fundamental knowledge allowed for some reinterpretations, which made these experiences useful to others. The term “experience” refers to the fact that these exchanges relied on the connexion of multiple isolated actions (such as those identified in the previous section) within farmers’ discourses and interpretations. Furthermore, one experience joins several actions but also the emergent aspects linked with their implementation, such as the specific variations of a machine action due to fine-tuning, or the climatic conditions at the time the action takes place. In our case studies, we observed that simple experience sharing could rapidly lead to various explanations, depending on the arena, for local characteristics were pointed to as the sole cause of these differences, which prevented more interpretation and learning from others’ experiences. Conversely, when a specific bio-physical phenomenon was used to reinterpret the various experiences, the results were not just used to deduce whether or not a technique “worked”, but mostly to validate the farmer’s existing knowledge specific to his situation, that is, to reinterpret the setting, according to Lave’s terminology. Personal experiences, when related to a specific bio-physical phenomenon, also provide an illustration of fundamental knowledge on this phenomenon, even if the variability of the results they show is not fully explained. In that sense, there is both a reinterpretation of these experiences taking into account the new understanding afforded by the fundamental knowledge, and a reformulation of this knowledge through existing experiences. Cross-comparing the different experiences allowed farmers to gradually confirm a particular aspect of the functioning of the system, based on fundamental knowledge. In other words, a generic knowledge, i.e. the understanding of a phenomenon in its functional aspects, can be developed from contextualized experiences, which may be used to design practices in new situations. Moreover, when fundamental knowledge is confirmed, the slight differences in results or observations in various experiences may call for further specification. In Case 1, the farmers successively shared their own experiences with different thistle management strategies, discussing the results, but struggling to find a common conclusion on the effects of different techniques because of the variability in soil structure and management practices, weed species and pressure intensity, crop sequences, and the climate. However, when one of them related each practice and result to the dynamics of thistle’s reserves, they found consistency in these results and deduced the possible management techniques to be applied to the situation discussed. A generic understanding of the effect of possible practices, different from the fundamental generic knowledge introduced, was built jointly from the reinterpretation of the various experiences, with the fundamental knowledge. Concerning the need to further specify the knowledge identified through experience sharing, in Case 1, these comparisons allowed farmers to reconsider the significance of their observations (thistle regrowth becomes a positive process because it signals a decrease in its reserves), but also highlighted the need to be more accurate in the description of reserve dynamics during the discussion. Furthermore, future actions planned to compare mowing and scalping effects in an exhaustion strategy were also geared towards specifying the exact type and intensity of cutting that induces the greatest regrowth.
This translation of personal experiences into useful references for re-design relates to what Stake [Citation41] called “vicarious experience”, a contextualized form of experience called into memory as “vignettes” which cannot be reduced to findings, but only be told. Sharing previous observations and results allows a collective to perform “narrative sensemaking”, which produces a combination of “if …then” rules of action, as well as an understanding of the partial system functioning underpinning these rules. This finding from our case studies is also in line with what Pålshaugen [Citation43] called “practical discourses” containing “public interpretations of personal experiences”. The social learning in such experience sharing thus corresponds less to a “shoring” relationship [Citation44], in which a more experienced practitioner participates in the development of a pair’s competencies, than to a collective “problem setting”. According to Schön [Citation45], this is a process in which we interactively name the things we are dealing with and frame the context in which we deal with them. In these exchanges, farmers collectively build a new theory of the unique case through what Schön called a “reflection on action” and “in action”. However, whereas Schön mainly described a reflective practitioner as someone being reflective in a “conversation with the situation”, we show how the collective reflective setting of the problem may extensively rely on exogenous knowledge linked with the variety of situations of action.
4.4 Farmers apply three main processes to link generic knowledge to their own system
The previous analyses focused on the fundamental knowledge used, specifying its nature, and on the different ways it is articulated in action. We now propose an analysis of the way it is mobilized in the particular situations faced by the farmers. We identified three different processes participating in the reformulation of knowledge, which the farmers applied in order to gradually form an understanding of a part of their cropping system. These processes can be summed up as (): 1) non-situated knowledge on generic aspects of the biological objects is tailored in order to situate a biological process/phenomenon in a given environment; 2) the situated biological phenomenon is related to the effects of actions which impact it; 3) other practices that can have the same effects on the phenomenon are considered. Although continuity between these processes may appear, they were rarely observed in the corresponding full sequence in our case studies. We further describe each process below.
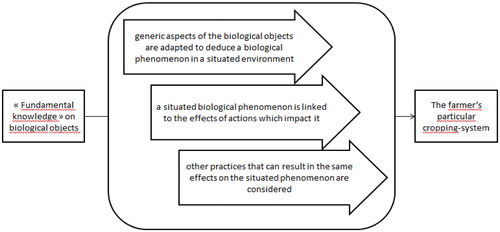
First, the non-situated knowledge concerns the biological objects, and is thus independent from the environment in which such objects are or would be manipulated (, line 2). Patterns or processes described concerning these objects may vary in intensity or accurate values in different environments, but they are stable features of the objects (e.g. the thistle increases root reserves in summer, which is true in various environments, although the rate of accumulation and quantities may vary according to the climate and soil nutrient contents). Hence, farmers try to complement this knowledge with the influence of the environment (climatic and biotic context) in a particular situation, to situate the phenomenon involving the biological objects. Relations with a specific environment were for instance specified in terms of modulated dates at which a phenomenon may occur, that take into account the local climate (Case 1: 15 October is a situating element; the date at which the root reserves is at minimum was also subject to modulation according to the local climate, which was confirmed from past observations of thistle’s development), or the presence of specific species such as wild oats and foxtail pressure in the targeted situations in Case 5.
Second, farmers related the situated biological process to the effects of their own actions. This allowed them to validate, confirm or specify the direct and indirect results of specific practices, and involved the various patterns presented in Section 4.2. Sense making in this process appeared to focus on the distinction between the description of a biological process in the environment occurring without direct human intervention and the part of the process induced by human intervention. In Case 1, a farmer asked “you say that there is only 3 to 5% of thistle plants which come from seeds, but it is because we avoid flowering? or is this the case even in a wild system?” This second process also materialized in Case 1 when farmers tried to re-draw the curve representing the amount of thistle root reserves throughout the year when different cuttings were performed. Interestingly, Walker and Sinclair [Citation46], who proposed a method to elicit and formalize local qualitative knowledge, emphasized the relevance of distinguishing the objects, processes and actions in order to establish the causal links between them. They stated that the “distinction between natural processes and human intervention was found to improve knowledge elicitation”. Thus, situating the phenomenon or process corresponds not only to answering the question “will it occur and is such a description valid in this situation?”, but also to answering the one: “what are the stable features of the phenomenon and what are the main variation factors related to actions?”.
Third, the specified influence of human action on the biological phenomenon was used as a base to broaden the range of practices that may have the same effect. This led to identifying other actions impacting the same situated phenomenon (in Case 4, the interactions between cover crops and diversified crop sequences was expressed by the farmer in terms of the impact on soil bacteria diversity), to specifying the quality or intensity of the relationship between an action and a situated mechanism, or to identifying other mechanisms of interest (Case 1: the cover-crops preventing soil tillage led to considering whether repeated topping would also deplete thistle reserves, and to tackling another mechanism − the effect of competition for light between thistle and cover-crop species on the accumulation of roots’ reserves).
In contrast with Section 4.3, which showed how particular and situated experiences were used to bring out causal relations within the cropping systems, the description of these three processes addresses the way farmers contextualize very generic knowledge on non-situated biological objects. The contextualization we analysed does not amount to simply validating the knowledge discussed in a particular situation based on various contextual elements, which would correspond to a single-step decision to mobilize knowledge in action in this situation. Rather, it involves a gradual transformation and reformulation of this knowledge, in order to build situated meaning for action, that is, to construct its meaning for a particular cropping system. By distinguishing between the different elementary processes which appeared necessary for such contextualization, we were able to unravel how specific fundamental knowledge may give farmers a “hold on reality” [Citation47]. However, as it represents a farmer’s point of view, it does not correspond to a broad definition of the contextualization of scientific knowledge, as defined by [Citation23]: “the combination of explications of values and aspirations, fitting to context and interpretation of model work in relation to other knowledge sources”. The objectivity of fundamental knowledge is somehow questioned as it acquires legitimacy from the farmers’ point of view when combined with experiential knowledge on possible actions.
4.5 The gradual linking of fundamental knowledge to interacting practices in the cropping system: an inherently situated process
Farmers successively and consistently put together different aspects of the functioning of limited parts of the system. This might be considered as the process whereby farmers constitute what Ingram et al. [Citation28] call farmers’ “broad view” (specifying that “farmers have an overview over the properties of their land and an impression of the state of the soil and their crops‘), as opposed to scientists’ “deep view’ (as they ‘gain deep insight into their topic by neglecting the broader production-related connections made by farmers’). However, we argue that farmers do benefit from insight into the functioning of the biological objects that their actions may impact. The four patterns followed to link knowledge on biological objects to farmers’ action (described in Section 4.2) showed that farmers develop knowledge, in a joint and iterative way, on the biological objects involved in their cropping system, and on the actions which are part of this system (). This leads to the situated development of a contextualized understanding of the functioning of a part of the cropping system which includes action. The causality built in such understanding directly include the situated actions rather than potential actions proposed as solutions.
The contextualisation we described does not only rely on tacit knowledge. It builds a rationality which a-priori is not entirely included in fundamental knowledge, and it includes action in a situated way. What stimulates the conceptualization are the variations in consequences observed in a set of situations. This implies building an understanding on these variations from the comparison of the interpretative logics. As such, although based on the introduction of fundamental scientific knowledge, the processes described are opposed to a “laboratorization” which adapts the situation to the implementation of a scientific rationality. They combine knowledge about action and in action, from the situation to the introduced knowledge. This produces a rationality for action within the cropping systems, which is more than what the fundamental knowledge introduced offers. The meaning and the new understanding of the phenomenon addressed in the situations are built by the farmers themselves, and are not fully provided by the scientific concept.
As a consequence, one may ask how such transformation of knowledge may be accomplished or facilitated a priori. A situated knowledge approach in terms of process and not only content then appears appropriate, as argued by Briggs [Citation48] in Indigenous Knowledge studies. Whereas most studies have focused on the integration and legitimation of indigenous knowledge through formal Western science, Briggs has argued that a focus on processes is necessary: ways of observing, discussing, questioning, analysing and making sense of information, whether it is new or received. The processes we described suggest that the situated knowledge is a situated process of new knowledge generation and not only a knowledge with specific characteristics that can become generic and integrated into scientific and local knowledge. Such contextualisation processes should be seen as a continuously evolving “genesis” that produces situated knowledge, and in which any concept acquires new meaning, depending on the situation. [Citation49] Finally, we can illuminate some particularities about how a systemic understanding is evolving. First, it engages a dialectic of various temporal scales and perspectives, namely juggling between the past and known actions, the current problem that focuses attention on specific agricultural objects and observations of the present situation, and future possible actions anticipated on the basis of the new concepts formed. This particular embeddedness of temporality in situated expert knowledge has also been underlined by Riley [Citation50]. Second, the intertwining of multiple actions and the iterative way that their interpretation is built jointly with the introduction of fundamental knowledge, can be seen as a particular system understanding: the action and its uncertainties are part of the system design, and the understanding targets an action within this system, rather than on it.
5 Conclusion
This article focused on cropping system re-design and addressed the link farmers make between generic and fundamental knowledge, their situated action on particular systems, and the systemic approach it entails. One major finding concerned the building of an understanding of the functioning of a limited part of their own system by farmers, and the role of such understanding in choosing, adapting and implementing new practices. In our study, the causality included in the situated rationality of action strategies farmers built within their systems was not included in the introduced scientific knowledge. Knowledge of the system increases in a joint dynamics, along with knowledge of action that farmers implement. Our conclusion is therefore not simply that it is necessary to further extend knowledge on biological system components in any way possible. In fact, scientists wishing to support these re-design processes should produce knowledge which might be articulated in farmers’ action on the scale of the processes and impacts of delimited techniques. The knowledge explored by agronomists around these conditions might be different from that resulting from a continuous inquiry led by scientists detached from action, and building causality systems making invisible, or non-compatible, some contextualising processes. It is worth remembering that these findings relate to re-design situations geared towards a greater mobilization of biological processes. This might explain the specific focus on fundamental knowledge about biological components of the system. Furthermore, the processes we described suggest that R&D agronomists should play a particularly significant role in identifying the possible links farmers operate between generic knowledge and their situated actions for re-design [Citation51,Citation52]. Rather than supplying sets of operational procedures and “best practices”, they should contribute to farmers’ identification and observation of the situated biological phenomenon and the way they are affected by the various actions, and to the reformulation of individual experiences regarding this phenomenon, in order to support the development of farmers’ understanding of their own cropping system functioning. In return, agronomists’ involvement in such processes might shed light on the directions which the production of scientific knowledge should follow.
Table 3: Examples and quotations from the case-studies for cross-cutting findings illustration
Download MS Word (37.7 KB)Acknowledgements
This work was supported by the Région Ile-de-France under a grant from DIM Astréa; and INRA under a grant from the meta-program SMaCH. This work was realised under the umbrella of the Initiative for Design in Agrifood Systems. We thank Nonta Libbrecht-Carey for language editing the English version of this paper.
References
- M.DuruO.TherondG.MartinR.Martin-ClouaireM.-A.MagneE.JustesE.-P.JournetJ.-N.AubertotS.SavaryJ.-E.BergezJ.P.SarthouHow to implement biodiversity-based agriculture to enhance ecosystem services: a reviewAgron. Sustain. Dev.3520151259128110.1007/s13593-015-0306-1
- R.BiggsM.SchlüterD.BiggsE.L.BohenskyS.BurnSilverG.CundillV.DakosT.M.DawL.S.EvansK.KotschyA.M.LeitchC.MeekA.QuinlanC.Raudsepp-HearneM.D.RobardsM.L.SchoonL.SchultzP.C.WestToward principles for enhancing the resilience of ecosystem servicesAnnu. Rev. Environ. Resour.37201242144810.1146/annurev-environ-051211-123836
- M.A.AltieriThe ecological role of biodiversity in agroecosystems, AgricultureEcosyst. Environment.741999193110.1016/S0167-8809(99)00028-6
- J.IngramAre farmers in England equipped to meet the knowledge challenge of sustainable soil management? An analysis of farmer and advisor viewsJ. Environ. Manage.86200821422810.1016/j.jenvman.2006.12.036
- N.G.RölingJ.L.S.JigginsPolicy paradigm for sustainable farmingEur. J. Agric. Educ. Ext.11994234310.1080/13892249485300041
- J.ClarkJ.MurdochLocal knowledge and the precarious extension of scientific networks: a reflection on three case studiesSoc. Ruralis371997386010.1111/1467-9523.00035
- T.Dorຝ.MakowskiE.MalézieuxN.Munier-JolainM.TchamitchianP.TittonellFacing up to the paradigm of ecological intensification in agronomy: revisiting methods, concepts and knowledgeEur. J. Agron.34201119721010.1016/j.eja.2011.02.006
- E.MalézieuxDesigning cropping systems from natureAgron. Sustain. Dev.322012152910.1007/s13593-011-0027-z
- D.H.WalkerP.J.ThorneF.L.SinclairB.ThapaC.D.WoodD.B.SubbaA systems approach to comparing indigenous and scientific knowledge: consistency and discriminatory power of indigenous and laboratory assessment of the nutritive value of tree fodderAgric. Syst.6219998710310.1016/S0308-521X(99)00058-X
- M.A.AltieriV.M.ToledoNatural resource management among small-scale farmers in semi-arid lands: building on traditional knowledge and agroecologyAnn. Arid Zone442005365
- T.BaarsExperiential science; towards an integration of implicit and reflected practitioner-expert knowledge in the scientific development of organic farmingJ. Agric. Environ. Ethics24201160162810.1007/s10806-010-9281-3
- I.FazeyJ.A.FazeyJ.G.SalisburyD.B.LindenmayerS.DoversThe nature and role of experiential knowledge for environmental conservationEnviron. Conserv.332006110.1017/S037689290600275X
- R.ChikowoV.FaloyaS.PetitN.M.Munier-JolainIntegrated Weed Management systems allow reduced reliance on herbicides and long-term weed controlAgric. Ecosyst. Environ.132200923724210.1016/j.agee.2009.04.009
- V.DeytieuxT.NemecekR.Freiermuth KnuchelG.GaillardN.M.Munier-JolainIs Integrated Weed Management efficient for reducing environmental impacts of cropping systems? A case study based on life cycle assessmentEur. J. Agron.362012556510.1016/j.eja.2011.08.004
- X.CoquilJ.-L.FiorelliA.BlouetC.MignoletExperiencing organic mixed crop dairy systems: a step-by-Step design centred on a long-term experimentS.BellonS.PenvernOrganic Farming, Prototype for Sustainable Agricultures2014SpringerNetherlands201217(accessed April 26 2015)http://link.springer.com/chapter/10.1007/978-94-007-7927-3_11
- R.L.McCownG.L.HammerJ.N.G.HargreavesD.P.HolzworthD.M.FreebairnAPSIM: a novel software system for model development, model testing and simulation in agricultural systems researchAgric. Syst.50199625527110.1016/0308-521X(94)00055-V
- W.A.H.RossingJ.M.MeynardM.K.Van IttersumModel-based explorations to support development of sustainable farming systems: case studies from France and the NetherlandsDev. Crop Sci.251997339351
- F.AffholderD.JourdainD.D.QuangT.P.TuongM.MorizeA.RicomeConstraints to farmers’ adoption of direct-seeding mulch-based cropping systems: a farm scale modeling approach applied to the mountainous slopes of VietnamAgric. Syst.1032010516210.1016/j.agsy.2009.09.001
- C.KollasK.C.KersebaumC.NendelK.ManevskiC.MüllerT.PalosuoC.M.Armas-HerreraN.BeaudoinM.BindiM.CharfeddineT.ConradtJ.ConstantinJ.EitzingerF.EwertR.FerriseT.GaiserI.G.de Cortazar-AtauriL.GiglioP.HlavinkaH.HoffmannM.P.HoffmannM.LaunayR.ManderscheidB.MaryW.MirschelM.MoriondoJ.E.OlesenI.ÖztürkA.PacholskiD.Ripoche-WachterP.P.RoggeroS.RoncossekR.P.RötterF.RugetB.SharifM.TrnkaD.VentrellaK.WahaM.WegehenkelH.-J.WeigelL.WuCrop rotation modelling—A European model intercomparisonEur. J. Agron.7020159811110.1016/j.eja.2015.06.007
- J.ConstantinC.Le BasE.JustesLarge-scale assessment of optimal emergence and destruction dates for cover crops to reduce nitrate leaching in temperate conditions using the STICS soil-crop modelEur. J. Agron.692015758710.1016/j.eja.2015.06.002
- N.ColbachA.CollardS.H.M.GuyotD.MézièreN.Munier-JolainAssessing innovative sowing patterns for integrated weed management with a 3D crop:weed competition modelEur. J. Agron.532014748910.1016/j.eja.2013.09.019
- J.ConstantinN.BeaudoinM.LaunayJ.DuvalB.MaryLong-term nitrogen dynamics in various catch crop scenarios: test and simulations with STICS model in a temperate climateAgric. Ecosyst. Environ.1472012364610.1016/j.agee.2011.06.006
- B.SterkC.LeeuwisM.K.van IttersumLand use models in complex societal problem solving: plug and play or networking?Environ. Modell. Software24200916517210.1016/j.envsoft.2008.07.001
- Z.HochmanJ.CouttsP.S.CarberryR.L.MccownThe FARMSCAPE Experience: Simulations Aid Participative Learning in Risky Farming Systems in Australia2000(accessed September 23, 2015)http://cat.inist.fr/?aModele=afficheN%26cpsidt=1007902
- L.ProstM.CerfM.-H.JeuffroyLack of consideration for end-users during the design of agronomic models. A reviewAgron. Sustain. Dev.32201258159410.1007/s13593-011-0059-4
- M.S.ReedL.C.StringerI.FazeyA.C.EvelyJ.H.J.KruijsenFive principles for the practice of knowledge exchange in environmental managementJ. Environ. Manage.146201433734510.1016/j.jenvman.2014.07.021
- J.FarringtonA.M.MartinFarmer participatory research: a review of concepts and recent fieldworkAgricu. Adm. Ext.29198824726410.1016/0269-7475(88)90107-9
- J.IngramP.FryA.MathieuRevealing different understandings of soil held by scientists and farmers in the context of soil protection and managementLand Use Policy272010516010.1016/j.landusepol.2008.07.005
- S.JordanJ.WhiteR.GunsolusLearning groups developing collaborative learning methods for diversified, site-specific weed management: a case study from Minnesota, USACow up a Tree: Knowing and Learning for Change in AgricultureINRA Paris(2000) 85–95.
- J.M.MeynardB.DedieuA.P.(Bram) BosRe-design and co-design of farming systems. An overview of methods and practices,)D.DarnhoferFarming Systems Research into the 21 st Century2012The New Dynamic, SpringerNetherlands40542910.1007/978-94-007-4503-2_18(accessed June 17 2013)
- A.WezelM.CasagrandeF.CeletteJ.-F.VianA.FerrerJ.Peignບgroecological practices for sustainable agriculture. A reviewAgron. Sustain. Dev.34201412010.1007/s13593-013-0180-7
- J.M. Meynard, System experiments: methodological progress, Montpellier, France, 2015.
- A.DavidEtude de cas et généralisation scientifique en sciences de gestion2003(accessed November 10, 2015)http://basepub.dauphine.fr/xmlui/handle/123456789/1444
- B.G.GlaserA.L.StraussThe Discovery of Grounded Theory: Strategies for Qualitative Research2009Transaction Publishers
- P.MischlerS.LheureuxF.DumoulinP.MenuO.SeneJ.-P.HopquinM.CariolleR.ReauN.MunierjolainV.FaloyaHuit fermes de grande culture engagées en production intégrée réduisent les pesticides sans baisse de margeLe Courrier de L Environnement de lINRA.5720097391
- R. Amalberti, Modèles d’activité en conduite de processus rapides: implications pour l’assistance à la conduite, Paris 8, 1992. http://www.theses.fr/1992PA080718 (accessed November 10, 2015).
- M.CerfLES CONNAISSANCES MOBILISÉES PAR DES AGRICULTEURS POUR LA CONCEPTION ET LA MISE EN OEUVRE DE DISPOSITIFS D’INTERVENTION CULTURALELe Travail Humain.591996305333
- J. Dewey, Logique. La théorie de l’enquête, Paris, PUF. (1993).
- P.MayenDéveloppement professionnel et formation: une théorie didactique, Université Pierre Mendes FranceGrenoble: Habilitation à Diriger des Recherches2001
- J.LaveCognition in Practice: Mind, Mathematics and Culture in Everyday Life1988Cambridge University Press(accessed October 31, 2016)http://https://books.google.fr/books?hl=fr%26lr=%26id=n6eiH3iPVKYC%26oi=fnd%26pg=PR10%26dq=arena+settings+authornbsp:lave%26ots=cbN7c8Jsoh%26sig=aCuxBrpC8qepHNkxGI7i_LplHJw
- R.E.StakeCase study: composition and performanceBull. Council Res. Music Educ.19943144
- Ø.PålshaugenHow to do things with words: towards a linguistic turn in action research?Concepts Transform.9200418120310.1075/cat.9.2.07pal
- J.BrunerPlay, thought, and languagePeabody J. Educ.6019836069
- D.A.SchönThe Reflective Practitioner: How Professionals Think in Action1983Basic books(accessed November 17, 2015)http://https://books.google.fr/books?hl=fr%26lr=%26id=ceJIWay4-jgC%26oi=fnd%26pg=PR7%26dq=authornbsp:schon%26ots=q74US-CSvj%26sig=fuvMAYnmWlryXm2Hj6rpceXI4xA
- D.H.WalkerF.L.SinclairAcquiring qualitative knowledge about complex agroecosystems Part 2: Formal representationAgric. Syst.56199836538610.1016/S0308-521X(97)00049-8
- M.MormontDes Savoirs Actionnables2007(accessed December 14, 2014)http://orbi.ulg.ac.be/handle/2268/22372
- J.BriggsIndigenous knowledge: a false dawn for development theory and practice?Prog. Dev. Stud.132013231243
- J.S.BrownA.CollinsP.DuguidSituated cognition and the culture of learningEduc. Res.1819893242
- M.RileyExperts in their fields: farmer −expert knowledges and environmentally friendly farming practicesEnviron. Plann. A0200910.1068/a39253(0-0)
- C.DelbosO.DavidA.MinasM.CerfC.FalgasC.A.GagneurJ.D.GiletV.LaudinotA.SigwaltE.WaldemeirConseil agronomique et réduction des pesticides: quelles ressources pour affronter ce nouveau challenge professionnel?Innov. Agronomiques.2014367378
- M.CerfB.OmonE.ChantreM.-N.GuillotM.Le BailC.LamineP.OlryVers des systèmes économes en intrants: quelles trajectoires et quel accompagnement pour les producteurs en grandes cultures?Innov. Agronomiques2010105119
Appendix A
Supplementary data
Supplementary data associated with this article can be found, in the online version, at http://doi:10.1016/j.njas.2016.11.004.