Abstract
Hepatitis E virus (HEV), a single-stranded, positive-sense RNA virus, is responsible for acute hepatitis E epidemics in many developing countries, and the virus is also endemic in some industrialized countries. Hepatitis E is a recognized zoonotic disease, and several animal species, including pigs, are potential reservoirs for HEV. The genome of HEV contains three open reading frames (ORFs). ORF1 encodes the nonstructural proteins, ORF2 encodes the capsid protein, and ORF3 encodes a small multifunctional protein. The ORF2 and ORF3 proteins are translated from a single, bicistronic mRNA. The coding sequences for these two ORFs overlap each other, but neither overlaps with ORF1. Whereas the mechanisms underlying HEV replication are poorly understood, the construction of infectious viral clones, the identification of cell lines that support HEV replication, and the development of small animal models have allowed for more detailed study of the virus. As result of these advances, recently, our understanding of viral entry, genomic replication and viral egress has improved. Furthermore, the determination of the T=1 and T=3 structure of HEV virus-like particles has furthered our understanding of the replication of HEV. This article reviews the latest developments in the molecular biology of HEV with an emphasis on the genomic organization, the expression and function of genes, and the structure and replication of HEV.
Emerging Microbes & Infections (2012) 1, e17; doi:10.1038/emi.2012.7
Introduction
Hepatitis E virus (HEV) is a small, non-enveloped virus with a single-stranded, positive-sense RNA genome that is approximately 7.2 kb in size.Citation1,Citation2 HEV is transmitted mainly via a fecal–oral route and is the causative agent of hepatitis E, which is an important public health disease in many developing countries. Sporadic and cluster cases of acute hepatitis E have also been reported in many industrialized countries,Citation1,Citation3,Citation4 including Japan,Citation5 the United States,Citation6 and countries in Europe.Citation7 Unlike other forms of acute viral hepatitis, HEV infection is associated with a relatively high mortality rate (up to 30%) in infected pregnant women.Citation8,Citation9,Citation10 Four major genotypes of mammalian HEV are recognized; genotypes 1 and 2 are restricted to human infection, whereas genotypes 3 and 4 are zoonotic. Pigs are a known reservoir for HEV, and several other animal species, including deer, rabbits and mongooses, may potentially serve as HEV reservoir.Citation11,Citation12,Citation13 Although the currently available HEV cell culture systems are not robust,Citation14 they do support the full virus replication cycle. Therefore, these systems afford an opportunity to understand the mechanisms underlying cell entry, genomic replication, virion morphogenesis and viral egress in vitro.Citation9,Citation15,Citation16,Citation17 Furthermore, the construction of infectious cDNA clones and replicons of HEV has paved the way for structural and functional studies of HEV genes,Citation18,Citation19,Citation20,Citation21,Citation22 and the development of pig- and chicken-based small animal models allows for the study of HEV replication and pathogenesis in homologous animal model systems.Citation23,Citation24 Finally, the recent determination of the three-dimensional structure of HEV virus-like particles (VLPs)Citation25,Citation26,Citation27,Citation28,Citation29 has further advanced our understanding of the molecular biologyand replication of this virus.
This article provides an overview of the recent advances in the understanding of the molecular biology and replication of HEV.
Host range and heterogeneity of HEV
HEV is a member of the Hepeviridae family,Citation2,Citation30 and two major species of HEV have been recognized. Mammalian HEV causes acute hepatitis in humans and has animal reservoirs in pigs and possibly other mammals,Citation12 whereas avian HEV is associated with hepatitis-splenomegaly syndrome in chickensCitation31 (). The species in the genus of Hepevirus includes the four major genotypes of mammalian HEV;Citation12,Citation13 genotypes 1 and 2 have been identified in humans in developing countries, and genotypes 3 and 4 have been identified in humans and other animal species, including domestic and wild pigs, deer, mongooses and rabbits in both developing and industrialized countries. Recently, two putative new HEV genotypes were identified. One was identified in wild boars in JapanCitation32 and the other in rats in GermanyCitation33,Citation34 ().
Figure 1 A phylogenetic tree based on the complete genomic sequences of representative HEV strains from each genotype. The tree was constructed using the neighbor-joining method with 1000 bootstrap analyses using MEGA5. The scale bar represents 0.05 nucleotide substitutions per position. The bootstrap values are labeled at the major nodes. The GenBank accession numbers of the HEV genomes are included in parenthesis after each strain.
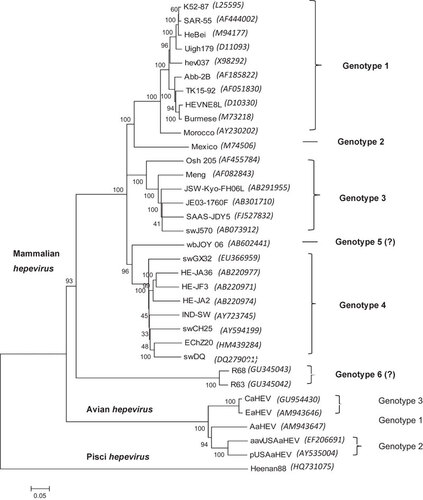
Table 1 Host range and heterogeneity of HEV
Avian HEV is currently classified as a separate, floating species in the Hepeviridae family.Citation2,Citation30 Currently, at least three genotypes of avian HEV have been identified from chickens worldwide.Citation2,Citation9,Citation35,Citation36 Because of the extensive sequence variation (approximately 50%) between avian and mammalian HEVs, it has been proposed to classify avian HEV as a new genus as opposed to its current classification as a floating species within the Hepeviridae familyCitation9 ().
Cutthroat trout virus (CTV) from fishCitation37 also appears to be a member of the Hepeviridae family. Because of the low level of sequence identity to mammalian or avian hepeviruses (13%–27%), CTV likely represents another new genus in this family of viruses ().
Genomic organization
The HEV genome is a single-stranded, positive-sense RNA molecule. Mammalian and fish HEV is approximately 7.2 kb in size, but avian HEV is approximately 6.7 kb in size.Citation38 The genome contains a short 5′ noncoding region (NCR), open reading frame (ORF) 1 that encodes the nonstructural proteins, ORF2 that encodes the capsid protein, ORF3 that encodes a small multifunctional protein, and a 3′ NCRCitation1 (). The proteins encoded by ORF2 and ORF3 are produced from a bicistronic subgenomic (SG) mRNA. A junction region (JR) that is similar to the alphavirus JR sequence is present between ORF1 and the start site of the subgenomic coding region. Therefore, the coding region for ORF2 overlaps with ORF3, but neither overlaps with ORF1.Citation19,Citation39 The 5′ end of the HEV genome contains a 7-methylguanosine cap structure, and the genome is organized as 5′ NCR-ORF1-JR-ORF3/ORF2-3′ NCR. Additionally, two cis-reactive elements (CRE) have been identified in the genome. The first CRE is essential for HEV replication and overlaps with both the 3′ end of ORF2 and the 3′ NCR.Citation40 The second CRE is located in the JR, and both the sequence and structure of the stem–loop (SL) in the JR are crucial for HEV replication.Citation22 Furthermore, the second CRE may be the promoter for the 2.0-kb SG mRNA.Citation19,Citation41
Figure 2 A schematic diagram of the genomic and subgenomic organization of the HEV genome. The three ORFs are labeled and shown as boxes with the putative ORF1 domains indicated inside the box. Modified from Cao et al. (2010).Citation22 CRE, cis-reactive element; Hel, helicase; HEV, hepatitis E virus; HVR, hypervariable region; JR, junction region; MT, methytransferase; NCR, noncoding region; ORF, open reading frame; PCP, a papain-like cysteine protease; RdRp, RNA-dependent RNA polymerase; SL, stem–loop structure; X, macro domain; Y, Y domain.
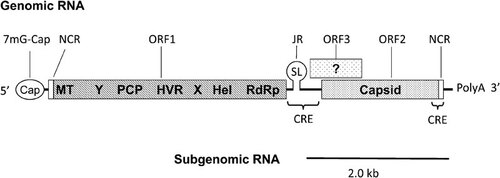
HEV proteins and their functions
Non-structural proteins (NSPs)
The HEV NSPs are encoded by ORF1. This coding region begins immediately after the 5′ NCR and extends 5082 bp (for the genotype 1 HEV Sar-55 strain). ORF1 encodes a 1693-amino acid (aa) polypeptide that is involved in viral replication and protein processing.Citation40 ORF1 contains several putative functional domainsCitation1,Citation42 (), including a methyltransferase (Met) domain for capping the 5′ end of the viral genomic RNA,Citation43 a ‘Y’ domain of unknown function, a papain-like cysteine protease (PCP) domain, a proline-rich region that contains a hypervariable region (HVR), an ‘X’ domain of unknown function that is next to the PCP domain, a helicase (Hel) domain, and an RNA-dependent RNA polymerase (RdRp) domain that is responsible for viral replication.Citation44
The Met domain is the first functional domain at the 5′ end of ORF1.Citation43 Both guaine-7-Met and guanyltransferase activities were detected in a baculovirus-expressed 110-kDa ORF1 polyprotein. Capping of the HEV genomic RNA was verified using a 5′ RNA ligase-mediated rapid amplification of cDNA ends method that can selectively amplify capped RNAs.Citation45 The cap is critical for viral infectivity, as only capped genomic RNA is infectious in non-human primate models.Citation40 A purified recombinant HEV Hel protein was shown to possess γ-phosphatase activity that may catalyze the first step in the formation of the cap,Citation46 suggesting that the Hel domain of the HEV protein is active and may be involved in the synthesis of the 5′ capped HEV RNA.
When expressed in Escherichia coli (E. Coli), the Hel domain of HEV possesses both nucleoside triphosphatase and 5′–3′ RNA duplex unwinding activities.Citation47 Mutations in the first walker A motif, including substitution of the K residue of the nucleotide-binding motif with an A residue, can lead to a 70% loss in ATPase activity. Similarly, mutating the DE residues in the Mg2+-binding motif present in the second walker B motif to aa residues caused a 50% reduction in ATPase activity. Mutations in both motifs abolished the Hel activity of the protein.
A putative PCP domain has been predicted in HEV ORF1;Citation42 however, definitive evidence for the translational and post-translational processing of the ORF1 polyprotein is still lacking. HEV ORF1 has been expressed as a 185-kDa polyprotein in E. coli, insect cells and mammalian cells.Citation48,Citation49,Citation50 When ORF1 was expressed in mammalian cells using a vaccinia virus-based expression system, two potential post-translational processing products of 107 and 78 kDa were observed only after an extended incubation time.Citation49 However, disruption of the predicted protease catalytic site in the ORF1 PCP domain did not affect the processing of the protein products. This suggests that the observed cleavage products are not generated by a viral protease and that they are likely produced by the expression system that was used in the study. Additionally, when HEV ORF1 was expressed in a baculovirus expression system, multiple smaller proteins were seen, and the presence of the putative processing products was abolished after the addition of the cell-permeable cysteine protease inhibitor E-64d.Citation51 Therefore, conclusive evidence detailing the processing of the ORF1 protein in the baculovirus system is still lacking.
More recently, the deconjugation activity of a purified recombinant protein that contained the Met and the papain-like cysteine protease domains (MetT-PCP) from ORF1 was analyzed using the following fluorogenic substrates: ubiquitin-7-amino-4-methylcoumarin (Ub-AMC), interferon-stimulated gene 15 (ISG15)-AMC, neural precursor cells expressed developmentally downregulated 8 (Nedd8)-AMC and small ubiquitin-like modifier (SUMO)-AMC. The MetT-PCP recombinant protein hydrolyzed all four substrates, and the hydrolysis of ISG15-AMC was significantly greater compared with the other substrates, suggesting that the HEV MetT-PCP recombinant protein possesses deubiquitinating activity.Citation52 However, processing of the Hel and the RdRp domains that contained the conserved LXGG site (position 1205) was not observed. Thus, it remains unclear whether the ORF1 polyprotein functions as a single protein with multiple functional domains or if the cleaved smaller proteins function independently.
The viral RdRp contains eight conserved motifs (motif I–VIII) that are similar to the RdRps from other positive-sense RNA viruses.Citation53 The GDD sequence is essential for HEV replicase activity, as demonstrated by mutation of an enhanced green fluorescent protein (EGFP)-containing HEV replicon.Citation54 The HEV RdRp can bind to the 3′ NCR of the HEV genome, and when expressed in E. coli, recombinant HEV RdRp can synthesize RNA in vitro using the 3′ polyadenylated HEV RNA as a template in a primer-independent manner.Citation44 Furthermore, the RdRp is localized to the endoplasmic reticulum (ER) of the cells that express RdRp recombinant protein,Citation55 suggesting that the ER may be involved in HEV replication.
An HVR was identified in ORF1of HEV. The HVR overlaps the proline-rich sequence that is located between the N terminus of the X domain and the C-terminal portion of the putative PCP domain.Citation42 The HVR varies both in length and sequence among different HEV strains.Citation56 It has been demonstrated that HEV can tolerate small deletions in the HVR and that the aa residues in this region are dispensable for viral infectivity.Citation56 However, the replication of HVR deletion mutants was markedly reduced in Huh7 cells, and a similar reduction in HEV replication was observed when HVR deletion mutants from avian HEV were tested in LMH chicken liver cells.Citation57 The HVR sequences are functionally interchangeable between different HEV genotypes with respect to viral replication and infectivity in vitro; however, genotype-specific HVR differences in replication efficiency have also been observed.Citation57 This suggests that while small deletions in the HVR do not have a significant impact on viral infectivity, the HVR may influence the efficiency of HEV replication by interacting with viral and/or host factors. Interestingly, a rare, recombinant HEV that contained a 174-base insertion from a human ribosomal protein gene was isolated when the Kernow-C1 strain of HEV, which was originally isolated from a human immunodeficiency virus-infected individual, was adapted to grow in human hepatocellular carcinoma cells.Citation15 The insertion in the HVR region was stable during serial passages in cell culture, and using an infectious cDNA clone, this insertion was found to be a key factor in the adaptation of the virus to growth in cell culture. Both the aa composition and the size of the insertion are important in viral replication.Citation58
Recently, it has been demonstrated that chimeric viruses that contain the ORF2 capsid gene either alone or in combination with the adjacent 5′ JR and 3′ NCR from a genotype 4 human HEV in the backbone of a genotype 3 swine HEV are replication competent in Huh7 cells, infectious in HepG2/C3A cells, and infectious in pigs. However, chimeric viruses that contain the JR, ORF2 and 3′ NCR from zoonotic genotypes 3 or 4 HEV that infect humans and pigs in the backbone of genotype 1 human HEV failed to infect pigs. This suggests that the 5′ NCR and ORF1 may be involved in cross-species HEV infection or may be related to host tropism.Citation59
The capsid protein
The HEV capsid protein is encoded by ORF2 and is 660 aa in length with a molecular weight of approximately 72 kDa. The capsid protein has a typical arginine-rich signal peptide sequence and three potential N-linked glycosylation sites.Citation1,Citation9 When expressed in vitro, capsid proteins of various sizes have been observed.Citation60,Citation61 In cells that are infected with recombinant Semliki Forest virus that contains ORF2, two larger ORF2-specific proteins were identified that were likely due to N-linked glycosylation.Citation62 However, when the HEV ORF2 protein was expressed in a baculovirus system, four capsid protein products of 72, 63, 56 and 53 kDa were observed. Sequence analyses revealed that the 53-, 56- and 63-kDa proteins were cleaved products from the full-length 72-kDa protein.Citation63
The ORF2 capsid protein is involved in the assembly of the HEV particle and its interaction with host cells. The capsid protein contains a potential ER localization signal at its N terminus, and while the protein does enters the ER, a fraction of the protein retrotranslocates to the cytoplasm.Citation64 The majority of the capsid protein expressed in mammalian cells is glycosylated, and the glycosylation is important for the formation of infectious virus particles.Citation20 The capsid also binds to HEV genomic RNACitation65 and may play a role in viral assembly. The capsid interacts with host cells and binds to heat shock protein 90 (HSP90),Citation66 glucose-regulated protein 78 (Grp78)Citation67 and heparin sulfate proteoglycans (HSPGs).Citation68 It has been demonstrated that HSPGs may serve as an attachment receptor to facilitate HEV entry into the host cells, whereas Grp78 or HSP90 may be involved in intracellular transport. Recently, it has been shown that three aa mutations (F51L, T59A and S390L) in the HEV ORF2 capsid proteinCitation21 result in viral attenuation. These three mutations significantly reduced viremia, delayed the onset time of viremia, shortened the duration of fecal virus shedding and viremia, and reduced the viral loads in the liver, bile and intestinal contents.Citation69
The HEV ORF2 capsid protein is immunogenic, and neutralizing antibodies have been shown to target it. Both linear and conformational epitopes for neutralizing antibodies have been mapped to aa residues 578–607,Citation70 452–617,Citation71 and 458–607Citation72 in the C-terminal portion of ORF2. Recent studies demonstrated that only antibodies recognizing conformational epitopes are neutralizing, and the aa residues Leu477 and Leu613 in the capsid protein are important in forming a neutralization-sensitive epitope.Citation73 Recently, the epitope of the 8C11 neutralizing antibody was identified using the complex structure of the HEV E2s domain and the 8C11 antibody. The neutralizing epitope was found to be conformational and located at aa residues 496–499, 510–514 and 573–578.Citation74 Western blot analysis showed that the recombinant avian HEV capsid protein reacted with both antisera against genotype 1 human HEV and convalescent antisera against genotype 3 swine and human HEVs. Similarly, convalescent sera from chickens that were experimentally infected with avian HEV reacted with the recombinant capsid proteins from the genotype 3 swine HEV and genotype 1 human HEV.Citation75 The convalescent serum from animals that were infected with any of the four mammalian genotypes of HEV all neutralized genotype 1 HEV.Citation76 Despite the broad antigenic cross-reactivity between the known HEV genotypes, antigenic variations have been observed among HEV strains using genotype- and strain-specific monoclonal antibodies.Citation77 Therefore, both pan-genotypic and strain-specific epitopes exist. It has been reported that aa residue 606 in the capsid is important in maintaining the antigenicity of the HEV ORF2 protein.Citation77 The E2 domain of the capsid protein, including aa residues 455–602, forms a homodimer. A strong hydrophobic cluster at the dimer interface is maintained by the side chains of residues Val503, Trp548, Thr552, Ala555, Tyr557, Tyr561, Val598 and Val600 in both subunits of the dimer. The C-terminal residues Ala597, Val598, Ala599, Leu601 and Ala602 are also involved in the formation of the dimmer.Citation26
The cytoskeleton-associated multifunctional phosphoprotein
HEV ORF3 encodes a small, 114-aa, cytoskeleton-associated phosphoprotein that is translated from the bicistronic SG RNA. At its 3′ end, ORF3 overlaps ORF2 by approximately 300 nt, but it does not overlap ORF1.Citation19,Citation39 The protein encoded by ORF3 contains two N-terminal hydrophobic domains (D1 and D2) and two C-terminal proline-rich regions (P1 and P2).Citation17 The phosphorylated ORF3 protein interacts with the non-glycosylated capsid protein via a 25-aa region in the ORF3 protein. This ORF2–ORF3 interaction depends on the phosphorylation of the ORF3 protein at the Ser71 residue and may play a regulatory role in the assembly of HEV virions.Citation78 The C-terminal region of the ORF3 protein is multifunctional and appears to be involved in virion morphogenesis and pathogenesis.Citation78,Citation79,Citation80
When expressed in mammalian cells, the ORF3 protein is approximately 13 kDa in size, it is phosphorylated at the Ser71 residue, and it is associated with the cytoskeletal and membrane fractions.Citation81 Similarly, ORF3 proteins were also detected in cells that were transfected with the HEV genomic RNA.Citation18,Citation41,Citation82 The expression of the ORF3 protein displays both filamentous and punctate distribution patterns. The filamentous distribution reflects the interaction between the ORF3 protein and microtubules,Citation83 whereas the punctate distribution is associated with both early and recycling endosomes.Citation84 Using an in vitro replicon system, it was shown that the ORF3 protein is not required for HEV replication in Huh7 cells and other cell lines,Citation18 but the ORF3 protein is essential for both infection of rhesus macaquesCitation41 and the release of virions from infected cells.Citation16,Citation85 Monoclonal antibodies against the ORF3 protein can capture HEV particles from cell culture supernatants and serum from HEV-infected patients but cannot capture fecal virus. This suggests that the ORF3 protein is present on the surface of nascent HEV virions.Citation86
It has been shown that the ORF3 protein colocalizes with the cytoskeleton via interactions through the ORF3 D1 hydrophobic domain,Citation81 and it binds to mitogen-activated protein kinase (MAPK) phosphataseCitation87 and microtubules.Citation83 The D2 hydrophobic domain interacts with hemopexin and affects cellular iron homeostasis.Citation88 The P2 region contains a proline-rich PxxP motif and binds to sarcoma(src)-homology 3 (SH3) domain-containing proteins,Citation89 which have important functions in signal transduction pathways that promote cell survival.Citation90 It has been reported that the ORF3 protein activates extracellular signal-regulated kinases (Erks)Citation89 via the binding and inactivation of an Erk-specific MAPK phosphatase, Pyst1. The ORF3 protein binds Pyst1 through its D1 domain and blocks conformational changes in Pyst1 that are required for Erk-mediated activation.Citation87 It has also been reported that the ORF3 protein upregulates the expression of enzymes in the glycolytic pathway via the stabilization of hypoxia-inducible factor 1 (HIF-1),Citation91 and it interacts with the fibrinogen (FBG) Bβ chain.Citation92
The ORF3 protein inhibits the mitochondrial apoptosis pathwayCitation93 and delays the transport of phosphorylated signal transducer and activator of transcription 3 (pSTAT3) into the cell nucleus.Citation84 The ORF3 protein also delays the trafficking and degradation of the activated hepatocyte growth factor receptor (c-Met). Because of its effects on growth factor receptor trafficking, it is believed that the ORF3 protein promotes cell survival.Citation94 The ORF3 protein has also been shown to interact with α1-microglobulin and bikunin.Citation79,Citation80 In cells that express the ORF3 protein, there is an increase in the secretion of α1-microglobulin,Citation95 which is mediated by tumor susceptibility gene 101 (Tsg101). Recent studies have revealed that the ORF3 protein binds to Tsg101 via its PSAP motif,Citation95,Citation96 and that the ORF3 protein interacts with Tsg101 and α1-microglobulin to facilitate the assembly of the endosomal sorting complex required for transport (ESCRT) complexes.Citation95 It has also been reported that Tsg101 and the vacuolar protein-sorting (Vps) proteins Vps4A and Vps4B are involved in release of HEV particles. This suggests that the multivesicular body (MVB) pathway is involved in viral release.Citation97 It appears that the ORF3 PSAP motif is a late domain involved in the release of membrane-associated HEV particles from infected cells. The interactions between the ORF3 protein and host proteins may create a favorable environment for HEV replication and pathogenesis. In Huh7 liver cells, it has been demonstrated that ORF3 increases the phosphorylation of hepatocyte nuclear factor 4 (HNF4). Phosphorylation impairs the nuclear translocation of HNF4 and downregulates HNF4-dependent gene expression.Citation98 Taken together, these results suggest that the ORF3 protein is a multifunctional protein that appears to play an important role in HEV replication and pathogenicity.
CREs in HEV genome
Single-stranded, positive-sense RNA viral genomes generally form secondary and tertiary structures, known as CREs, that maintain the stability of the viral RNA genome and participate in molecular interactions. The CREs are involved in RNA–RNA interactions and the binding of viral and cellular proteins during viral replication. CREs are generally located in the conserved 5′ and 3′ NCRs of the viral genomes. However, the 5′ NCR of the HEV genome is only 26 nt long, has a cap structure and plays a role in the initiation of HEV replication.Citation40,Citation43,Citation45 No CRE has been identified in the 5′ NCR of the HEV genome.
The first CRE that was identified in the HEV genome is important for HEV replication, is located in the 3′ NCR, and overlaps with the 3′ end of the coding region for ORF2.Citation40 The 3′ NCR and an adjacent region form two SL structures (SL1 and SL2) () that both interact with the viral RdRp.Citation44 This interaction is sequence specific, because 3′ NCR deletion mutants that lack SL1, SL2 or the polyA failed to form a complex with the viral RdRp. This finding suggests that the 3′ end of the viral genome, including the SLs and the polyA stretch, is recognized by the viral RdRp. It has also been reported that despite the sequence variation in the 3′ termini of the different HEV genotypes, the replication of chimeric HEV genomes and replicons generated from different HEV genotypes is similar to the replication of the parental virus or replicon. This result suggests that viral replication is not significantly affected by swapping the 3′ NCR between different HEV genotypes.Citation99 However, it appears that both the structure and the sequence of the SLs in the 3′ NCR are critical for viral replication. A single nucleotide change that disrupts one base pair within SL2 at position 7106 in genotype 1 HEV significantly decreased viral replication.Citation40,Citation99 Moreover, both a short hairpin RNA (shRNA)Citation100 and a ribozymeCitation101 targeting the 3′ NCR of HEV effectively inhibited viral replication, further indicating that the 3′ NCR is important in HEV replication.
Figure 3 The secondary structure of the CREs of a genotype 1 HEV (strain Sar-55) as predicted using mfold. (A) The secondary structures at the 3' end of HEV genomic region (nt 7082–7192 An) with the SL1 and SL2 labeled.Citation40,Citation44 (B) Negative-polarity complement of the JR in the HEV genome (nt 5096–5157).Citation22 The regulatory SL is labeled, and the arrows indicate the start sites of the subgenomic mRNA, ORF2 and ORF3.
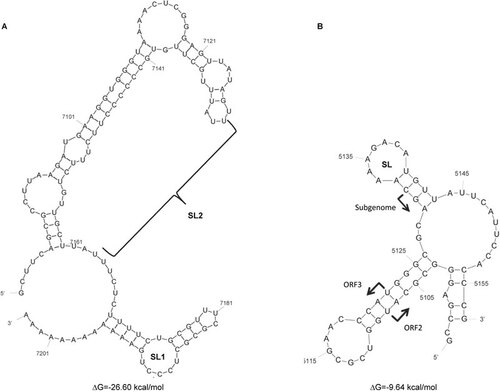
The second HEV CRE is located in the JR of the HEV genome. This CRE may serve as a promoter for the synthesis of the 2.0-kb SG mRNA.Citation22,Citation39,Citation41 It has been shown that when 6-nt or 4-nt mutations were introduced into the JR, neither ORF2 nor ORF3 protein expression was detectable in vitro,Citation41 suggesting the presence of a CRE in the JR. Subsequently, a highly conserved SL structure was identified within the HEV JR (). The JR shares nucleotide sequence identity with a JR in a Rubella virus and with the conserved alphavirus SG promoter sequence.Citation39 The role of the SL structure within the JR in HEV replication was demonstrated using Huh7 cells that were transfected with either wild-type or SL mutant replicons that contained a Renilla luciferase reporter.Citation22 Previous research has demonstrated that mutation of the AGA motif in the loop structure significantly reduced the levels of HEV replication. Additionally, mutations in the stem of the structure and in the subgenomic initiation site also significantly inhibited HEV replication. These results indicate that both the sequence and the SL structure in the JR are important for HEV replication. Delineating the exact role of the HEV RNA in viral replication will facilitate an increased understanding of the life cycle of the virus.
Virion structure and function
When expressed in insect cells, the N-terminal, truncated capsid protein can self-assemble into VLPs of two different sizes, T=1 and T=3.Citation29,Citation102,Citation103 The T=1 VLPs (270 Å in diameter) are smaller than native virions (320–340 Å) but have similar antigenicity and a similar surface structure.Citation104,Citation105 The crystal structures of T=1 VLPs of HEV genotype 1,Citation29 genotype 3Citation28 and genotype 4Citation25 have been determined, and a cryo-EM structure of T=3 VLPs has also been resolved.Citation29
Despite significant sequence differences between the capsid proteins of different HEV genotypes, the T=1 VLP structures that are derived from HEV genotypes 1, 3 and 4 are very similar.Citation25,Citation28,Citation29 The T=1 VLP structure is an icosahedral particle that consists of 60 identical capsid protein subunits.Citation29 Three distinct domains have been identified in the T=1 VLP structure, the S (Shell), M (middle) and P (protruding) domains.Citation25,Citation28,Citation29 The S domain is a jelly roll-like β-barrel fold and is conserved among many small RNA viruses.Citation25 The M domain comprises a twisted β-barrel with six antiparallel β-strands and four short α-helices. This domain binds to and interacts with the S domain.Citation25,Citation28 The P domain forms a dimeric spike that protrudes from the two-fold axis of symmetry in the T=1 VLPs.Citation25 The M and P domains are linked by a flexible, proline-rich hinge that facilitates the topological changes in the protruding spike in the T=1 VLPs.Citation25,Citation29 Dimeric, trimeric and pentameric interactions around the two-, three- and five-fold icosahedral axes are required for packaging of T=1 VLPs. The P domain forms a dimeric spikeCitation26 that functions as the viral receptor, is the site of neutralizing antibody binding,Citation25,Citation28,Citation29 and is important for antigenicity.Citation106
The Asn137 N-linked glycosylation site is partially hidden near the inner surface of the S domain, and the Asn310 N-linked glycosylation site is completely hidden by the interface of the capsid trimer. The Asn562 site is likely glycosylated in the ER.Citation28 It is likely that both the M and P domains are involved in virus entry.Citation28 A structure of T=1 VLPs in complex with the Fab224 neutralizing antibody has recently been resolved,Citation106 and this structure demonstrated that Fab224 recognizes a lateral surface of the P domain. Furthermore, it has been shown that insertion of an antibody epitope at the C terminus of the capsid protein does not interfere with the assembly of T=1 VLPs. This result indicates that T=1 VLPs may be good candidates for vaccine developmentCitation104 and may be useful as a potential oral vaccine vector.Citation106,Citation107
The HEV virion has also been predicted to have a T=3 icosahedral symmetry composed of 180 copies of the capsid protein.Citation25,Citation105 Recently, virion-sized T=3 VLPs were purified from insect cells that expressed HEV ORF2 with 13-aa residues truncation at the N terminus.Citation29 The cryo-EM-derived structure of the T=3 VLPs indicated that 180 copies of the capsid protein are assembled, thus confirming the previous prediction.Citation29 The capsid proteins of the T=3 VLP are grouped into three unique monomers, the A, B and C monomers. The A and B monomers form dimeric spikes around each of the five-fold icosahedral axes of symmetry, whereas the C monomers forma dimeric spike at each of the two-fold icosahedral axes of symmetry.Citation29 The N-terminal aa residues 14–111 are thought to prevent the ORF2 proteins from assembling into T=1 VLPs.Citation29 While T=1 structures have been described, it is believed that the native HEV capsid is a T=3 icosahedron.
Life cycle of HEV
Due to the lack of a robust cell-culture system for HEV, the life cycle of HEV is largely unknown.Citation17,Citation108,Citation109 The HEV capsid protein is believed to bind to a cellular receptor to initiate viral entry and replication. ORF2 peptide-binding experiments suggested that the C-terminal region of ORF2 may mediate virus entry by binding to heat shock cognate protein 70 (HSC70) on the cell surface.Citation110 Additionally, HSPGs have been identified as attachment receptors that are located on the cell surfaceCitation68 (). As a 23-nm particle, a truncated dimer of the HEV capsid protein (HEV239) potentially binds to the HEV receptor(s) on cells.Citation111 A potential sugar binding sequence is located in the M domain. This sequence forms a hidden pocket at the interface between the two capsid molecules around the three-fold axis of symmetry. The putative binding motif on the capsid is conserved between all four major mammalian HEV genotypes, suggesting a functional role of this domain in binding to the cellular receptor.Citation25 It has been reported that the truncated HEV 239 capsid protein also binds to Grp78, which is a cellular chaperone. This binding may play an important role in the HEV life cycle.Citation67 However, a specific cellular receptor for HEV still remains elusive. After virus entry into permissive cells, the HEV genomic RNA is uncoated by unknown mechanisms. An HSP90-specific inhibitor (geldanamycin) blocks the intracellular transport of the HEV239 capsid protein but does not affect the binding and entry of the truncated capsid protein. This suggests that HSP90 may play a role in the intracellular transport of HEV.Citation66
Figure 4 Proposed life cycle of HEV.Citation9,Citation17,Citation108,Citation109 Step a: HEV attaches to the cell surface via HSPGs, HSC70 or other putative attachment receptor(s) and then enters the cell via a unknown specific cellular receptor.Citation68,Citation110 Step b: The HEV virion penetrates the membrane and enters the cells. HSP90 and Grp78 may be involved in this transport. The virion then uncoats and releases the positive-sense genomic RNA into the cytoplasm of the cell. Step c: The positive-sense genomic viral RNA serves as the template to translate the ORF1 nonstructural polyprotein in the cytoplasm. Step d: The viral RdRp synthesizes an intermediate, replicative negative-sense RNA from the positive-sense genomic RNA that (step e) serves as the template for the production of positive-sense, progeny viral genomes. Step f: The ORF2 and ORF3 proteins are translated from the subgenomic, positive-stranded RNA, and (step g) the ORF2 capsid protein packages the genomic viral RNA and assembles new virions. Step h: The nascent virions are transported to the cell membrane. The ORF3 protein facilitates the trafficking of the virion, and (step i) the nascent virions are released from the infected cells. Modified from various studies.Citation17,Citation108,Citation109
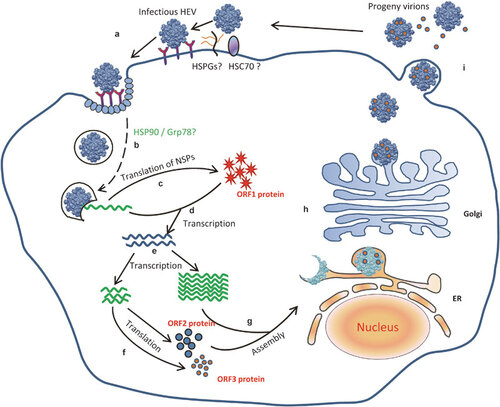
After uncoating of the viral genome and release of the viral genome into cells, the 7-methylguanosine cap structure in the 5′ NCR of the HEV genome recruits the 40S ribosomal subunit to initiate cap-dependent translation of viral proteins. The NSPs are translated from the genomic RNA. A negative-sense, replicative intermediate RNA is likely produced by the viral RdRp. This intermediate serves as the template for the production of positive-sense, progeny viral genomes. The activity of the HEV RdRp has been demonstrated in the HEV replicon systems,Citation44,Citation99 and the negative-sense intermediate viral RNA has been detected in tissues from HEV-infected animals.Citation112,Citation113,Citation114 The RdRp may bind to the CRE in the 3′ NCR of the HEV genome,Citation44 which could play an important role in HEV replication.Citation40,Citation99 The CRE in the JR of the HEV genome is important in the synthesis of the SG RNA.Citation22,Citation41 This SG RNA is translated to produce the structural capsid protein and the small ORF3 protein.
Both the assembly and release of HEV are poorly characterized. It is believed that the ORF2 protein packages the viral genome and has a role in the assembly of progeny virions. A 76-nt region at the 5′ end of the HEV genome has been identified as a binding domain for the ORF2 protein, and this region may be involved in the packaging of the HEV genome.Citation65 The ORF3 protein is believed to be involved in viral egress.Citation16,Citation115 The PSAP motif in the ORF3 protein is a late domain that is important for the release of the membrane-associated HEV particles from infected cells.Citation97
In summary, HEV is an important but grossly under studied pathogen. The HEV life cycle is poorly understood, and often, there are more questions than answers for many of the various steps in its replication. However, over the past decade, significant advances have been made in many aspects of HEV growth and replication, including the determination of the three-dimensional virion structure, the establishment of infectious cDNA clones, the identification of animal strains of HEV, and the development of small animal models. However, as most of the available molecular biology data are derived from cells over expressing individual viral proteins, the biological significance of these data in the context of virus infection is mostly unknown. Therefore, future studies that aim to delineate the structure and function of the HEV genes, to understand the molecular mechanisms of HEV replication, and to examine virus–host interactions are warranted. The recent development of a more efficient cell culture system for HEV replication will aid in the future study of HEV molecular biology.
The authors’ research on HEV is supported by grants from the US National Institutes of Health (R01 AI074667 and R01 AI050611).
- Emerson SU, Purcell RH. Hepatitis E Virus. In: Knipe DM, Howley PM, editors. Fields Virology. 5th ed. Philadelphia, PA: Lippincott-Raven Publishers; 2007. pp 3048–3059.
- Meng XJ. Hepatitis E Virus (Hepevirus). In: Mahy BWJ, Regenmortel MHV, editors. Encyclopedia of Virology. 3rd ed. Oxford: Academic Press; 2008. pp 377–383.
- Dalton HR, Bendall R, Ijaz S, Banks M. Hepatitis E: an emerging infection in developed countries. Lancet Infect Dis2008;8: 698–709.
- FitzSimons D, Hendrickx G, Vorsters A, van Damme P. Hepatitis A and E: update on prevention and epidemiology. Vaccine2010;28: 583–588.
- Mushahwar IK. Hepatitis E virus: molecular virology, clinical features, diagnosis, transmission, epidemiology, and prevention. J Med Virol2008;80: 646–658.
- Minuk GY, Sun A, Sun DF et al.Serological evidence of hepatitis E virus infection in an indigenous North American population. Can J Gastroenterol2007;21: 439–442.
- Bendall R, Ellis V, Ijaz S, Thurairajah P, Dalton HR. Serological response to hepatitis E virus genotype 3 infection: IgG quantitation, avidity, and IgM response. J Med Virol2008;80: 95–101.
- Navaneethan U, Al Mohajer M, Shata MT. Hepatitis E and pregnancy: understanding the pathogenesis. Liver Int2008;28: 1190–1199.
- Meng XJ. Recent advances in Hepatitis E virus. J Viral Hepat2010;17: 153–161.
- Naik SR, Aggarwal R, Salunke PN, Mehrotra NN. A large waterborne viral hepatitis E epidemic in Kanpur, India. Bull World Health Organ1992;70: 597–604.
- Meng XJ. Swine hepatitis E virus: cross-species infection and risk in xenotransplantation. Curr Top Microbiol Immunol2003;278: 185–216.
- Meng XJ. Hepatitis E virus: animal reservoirs and zoonotic risk. Vet Microbiol2010;140: 256–265.
- Meng XJ. From barnyard to food table: the omnipresence of hepatitis E virus and risk for zoonotic infection and food safety. Virus Res2011;161: 23–30.
- Okamoto H. Hepatitis E virus cell culture models. Virus Res2011;161: 65–77.
- Shukla P, Nguyen HT, Torian U et al.Cross-species infections of cultured cells by hepatitis E virus and discovery of an infectious virus-host recombinant. Proc Natl Acad Sci USA2011;108: 2438–2443.
- Emerson SU, Nguyen HT, Torian U, Burke D, Engle R, Purcell RH. Release of genotype 1 hepatitis E virus from cultured hepatoma and polarized intestinal cells depends on open reading frame 3 protein and requires an intact PXXP motif. J Virol2010;84: 9059–9069.
- Ahmad I, Holla RP, Jameel S. Molecular virology of hepatitis E virus. Virus Res2011;161: 47–58.
- Emerson SU, Nguyen H, Torian U, Purcell RH. ORF3 protein of hepatitis E virus is not required for replication, virion assembly, or infection of hepatoma cells in vitro. J Virol2006;80: 10457–10464.
- Graff J, Torian U, Nguyen H, Emerson SU. A bicistronic subgenomic mRNA encodes both the ORF2 and ORF3 proteins of hepatitis E virus. J Virol2006;80: 5919–5926.
- Graff J, Zhou YH, Torian U et al.Mutations within potential glycosylation sites in the capsid protein of hepatitis E virus prevent the formation of infectious virus particles. J Virol2008;82: 1185–1194.
- Huang YW, Haqshenas G, Kasorndorkbua C, Halbur PG, Emerson SU, Meng XJ. Capped RNA transcripts of full-length cDNA clones of swine hepatitis E virus are replication competent when transfected into Huh7 cells and infectious when intrahepatically inoculated into pigs. J Virol2005;79: 1552–1558.
- Cao D, Huang YW, Meng XJ. The nucleotides on the stem–loop RNA structure in the junction region of the hepatitis E virus genome are critical for virus replication. J Virol2010;84: 13040–13044.
- Halbur PG, Kasorndorkbua C, Gilbert C et al.Comparative pathogenesis of infection of pigs with hepatitis E viruses recovered from a pig and a human. J Clin Microbiol2001;39: 918–923.
- Billam P, Huang FF, Sun ZF et al.Systematic pathogenesis and replication of avian hepatitis E virus in specific-pathogen-free adult chickens. J Virol2005;79: 3429–3437.
- Guu TS, Liu Z, Ye Q et al.Structure of the hepatitis E virus-like particle suggests mechanisms for virus assembly and receptor binding. Proc Natl Acad Sci USA2009;106: 12992–12997.
- Li S, Tang X, Seetharaman J et al.Dimerization of hepatitis E virus capsid protein E2s domain is essential for virus–host interaction. PLoS Pathog2009;5: e1000537.
- Wang CY, Miyazaki N, Yamashita T et al.Crystallization and preliminary X-ray diffraction analysis of recombinant hepatitis E virus-like particle. Acta Crystallogr Sect F Struct Biol Cryst Commun2008;64: 318–322.
- Yamashita T, Mori Y, Miyazaki N et al.Biological and immunological characteristics of hepatitis E virus-like particles based on the crystal structure. Proc Natl Acad Sci USA2009;106: 12986–12991.
- Xing L, Li TC, Mayazaki N et al.Structure of hepatitis E virion-sized particle reveals an RNA-dependent viral assembly pathway. J Biol Chem2010;285: 33175–33183.
- Emerson SU, Anderson D, Arankalle A et al. Hepevirus. In: Fauquet CM, Mayo MA, Maniloff J, Desselberger U, Ball LA, editors. Virus Taxonomy VIIIth Report of the ICTV. London, United Kingdom. Elsevier Academic Press; 2004. pp 851–855.
- Payne CJ, Ellis TM, Plant SL, Gregory AR, Wilcox GE. Sequence data suggests big liver and spleen disease virus (BLSV) is genetically related to hepatitis E virus. Vet Microbiol1999;68: 119–125.
- Takahashi M, Nishizawa T, Sato H et al.Analysis of the full-length genome of a hepatitis E virus isolate obtained from a wild boar in Japan that is classifiable into a novel genotype. J Gen Virol2011;92: 902–908.
- Johne R, Plenge-Bonig A, Hess M, Ulrich RG, Reetz J, Schielke A. Detection of a novel hepatitis E-like virus in faeces of wild rats using a nested broad-spectrum RT-PCR. J Gen Virol2010;91: 750–758.
- Johne R, Heckel G, Plenge-Bonig A et al.Novel hepatitis E virus genotype in Norway rats, Germany. Emerg Infect Dis2010;16: 1452–1455.
- Bilic I, Jaskulska B, Basic A, Morrow CJ, Hess M. Sequence analysis and comparison of avian hepatitis E viruses from Australia and Europe indicate the existence of different genotypes. J Gen Virol2009;90: 863–873.
- Peralta B, Biarnes M, Ordonez G et al.Evidence of widespread infection of avian hepatitis E virus (avian HEV) in chickens from Spain. Vet Microbiol2009;137: 31–36.
- Batts W, Yun S, Hedrick R, Winton J. A novel member of the family Hepeviridae from cutthroat trout (Oncorhynchus clarkii). Virus Res2011;158: 116–123.
- Huang FF, Sun ZF, Emerson SU et al.Determination and analysis of the complete genomic sequence of avian hepatitis E virus (avian HEV) and attempts to infect rhesus monkeys with avian HEV. J Gen Virol2004;85: 1609–1618.
- Huang YW, Opriessnig T, Halbur PG, Meng XJ. Initiation at the third in-frame AUG codon of open reading frame 3 of the hepatitis E virus is essential for viral infectivity in vivo. J Virol2007;81: 3018–3026.
- Emerson SU, Zhang M, Meng XJ et al.Recombinant hepatitis E virus genomes infectious for primates: importance of capping and discovery of a cis-reactive element. Proc Natl Acad Sci USA2001;98: 15270–15275.
- Graff J, Nguyen H, Yu C et al.The open reading frame 3 gene of hepatitis E virus contains a cis-reactive element and encodes a protein required for infection of macaques. J Virol2005;79: 6680–6689.
- Koonin EV, Gorbalenya AE, Purdy MA, Rozanov MN, Reyes GR, Bradley DW. Computer-assisted assignment of functional domains in the nonstructural polyprotein of hepatitis E virus: delineation of an additional group of positive-strand RNA plant and animal viruses. Proc Natl Acad Sci USA1992;89: 8259–8263.
- Magden J, Takeda N, Li T et al.Virus-specific mRNA capping enzyme encoded by hepatitis E virus. J Virol2001;75: 6249–6255.
- Agrawal S, Gupta D, Panda SK. The 3′ end of hepatitis E virus (HEV) genome binds specifically to the viral RNA-dependent RNA polymerase (RdRp). Virology2001;282: 87–101.
- Zhang M, Purcell RH, Emerson SU. Identification of the 5′ terminal sequence of the SAR-55 and MEX-14 strains of hepatitis E virus and confirmation that the genome is capped. J Med Virol2001;65: 293–295.
- Karpe YA, Lole KS. RNA 5′-triphosphatase activity of the hepatitis E virus helicase domain. J Virol2010;84: 9637–9641.
- Karpe YA, Lole KS. NTPase and 5′ to 3′ RNA duplex-unwinding activities of the hepatitis E virus helicase domain. J Virol2010;84: 3595–3602.
- Ansari IH, Nanda SK, Durgapal H et al.Cloning, sequencing, and expression of the hepatitis E virus (HEV) nonstructural open reading frame 1 (ORF1). J Med Virol2000;60: 275–283.
- Ropp SL, Tam AW, Beames B, Purdy M, Frey TK. Expression of the hepatitis E virus ORF1. Arch Virol2000;145: 1321–1337.
- Suppiah S, Zhou Y, Frey TK. Lack of processing of the expressed ORF1 gene product of hepatitis E virus. Virol J2011;8: 245.
- Sehgal D, Thomas S, Chakraborty M, Jameel S. Expression and processing of the Hepatitis E virus ORF1 nonstructural polyprotein. Virol J2006;3: 38.
- Karpe YA, Lole KS. Deubiquitination activity associated with hepatitis E virus putative papain-like cysteine protease. J Gen Virol2011;92: 2088–2092.
- Koonin EV. The phylogeny of RNA-dependent RNA polymerases of positive-strand RNA viruses. J Gen Virol1991;72 (Pt 9): 2197–2206.
- Emerson SU, Nguyen H, Graff J, Stephany DA, Brockington A, Purcell RH. In vitro replication of hepatitis E virus (HEV) genomes and of an HEV replicon expressing green fluorescent protein. J Virol2004;78: 4838–4846.
- Rehman S, Kapur N, Durgapal H, Panda SK. Subcellular localization of hepatitis E virus (HEV) replicase. Virology2008;370: 77–92.
- Pudupakam RS, Huang YW, Opriessnig T, Halbur PG, Pierson FW, Meng XJ. Deletions of the hypervariable region (HVR) in open reading frame 1 of hepatitis E virus do not abolish virus infectivity: evidence for attenuation of HVR deletion mutants in vivo. J Virol2009;83: 384–395.
- Pudupakam RS, Kenney SP, Cordoba L et al.Mutational analysis of the hypervariable region of hepatitis E virus reveals its involvement in the efficiency of viral RNA replication. J Virol2011;85: 10031–10040.
- Shukla P, Nguyen HT, Faulk K et al.Adaptation of a genotype 3 hepatitis E virus to efficient growth in cell culture depended on an inserted human gene segment acquired by recombination. J Virol2012;86: 5697–5707.
- Feagins AR, Cordoba L, Sanford BJ et al.Intergenotypic chimeric hepatitis E viruses (HEVs) with the genotype 4 human HEV capsid gene in the backbone of genotype 3 swine HEV are infectious in pigs. Virus Res2011;156: 141–146.
- Jameel S, Zafrullah M, Ozdener MH, Panda SK. Expression in animal cells and characterization of the hepatitis E virus structural proteins. J Virol1996;70: 207–216.
- Torresi J, Meanger J, Lambert P, Li F, Locarnini SA, Anderson DA. High level expression of the capsid protein of hepatitis E virus in diverse eukaryotic cells using the Semliki Forest virus replicon. J Virol Methods1997;69: 81–91.
- Torresi J, Li F, Locarnini SA, Anderson DA. Only the non-glycosylated fraction of hepatitis E virus capsid (open reading frame 2) protein is stable in mammalian cells. J Gen Virol1999;80 (Pt 5): 1185–1188.
- Robinson RA, Burgess WH, Emerson SU et al.Structural characterization of recombinant hepatitis E virus ORF2 proteins in baculovirus-infected insect cells. Protein Expr Purif1998;12: 75–84.
- Surjit M, Jameel S, Lal SK. Cytoplasmic localization of the ORF2 protein of hepatitis E virus is dependent on its ability to undergo retrotranslocation from the endoplasmic reticulum. J Virol2007;81: 3339–3345.
- Surjit M, Jameel S, Lal SK. The ORF2 protein of hepatitis E virus binds the 5′ region of viral RNA. J Virol2004;78: 320–328.
- Zheng ZZ, Miao J, Zhao M et al.Role of heat-shock protein 90 in hepatitis E virus capsid trafficking. J Gen Virol2010;91: 1728–1736.
- Yu H, Li S, Yang C et al.Homology model and potential virus-capsid binding site of a putative HEV receptor Grp78. J Mol Model2011;17: 987–995.
- Kalia M, Chandra V, Rahman SA, Sehgal D, Jameel S. Heparan sulfate proteoglycans are required for cellular binding of the hepatitis E virus ORF2 capsid protein and for viral infection. J Virol2009;83: 12714–12724.
- Cordoba L, Huang YW, Opriessnig T et al.Three amino acid mutations (F51L, T59A, and S390L) in the capsid protein of the hepatitis E virus collectively contribute to virus attenuation. J Virol2011;85: 5338–5349.
- Schofield DJ, Glamann J, Emerson SU, Purcell RH. Identification by phage display and characterization of two neutralizing chimpanzee monoclonal antibodies to the hepatitis E virus capsid protein. J Virol2000;74: 5548–5555.
- Meng J, Dai X, Chang JC et al.Identification and characterization of the neutralization epitope(s) of the hepatitis E virus. Virology2001;288: 203–211.
- Zhou YH, Purcell RH, Emerson SU. An ELISA for putative neutralizing antibodies to hepatitis E virus detects antibodies to genotypes 1, 2, 3, and 4. Vaccine2004;22: 2578–2585.
- Zhang H, Dai X, Shan X, Meng J. The Leu477 and Leu613 of ORF2-encoded protein are critical in forming neutralization antigenic epitope of hepatitis E virus genotype 4. Cell Mol Immunol2008;5: 447–456.
- Tang X, Yang C, Gu Y et al.Structural basis for the neutralization and genotype specificity of hepatitis E virus. Proc Natl Acad Sci USA2011;108: 10266–10271.
- Haqshenas G, Huang FF, Fenaux M et al.The putative capsid protein of the newly identified avian hepatitis E virus shares antigenic epitopes with that of swine and human hepatitis E viruses and chicken big liver and spleen disease virus. J Gen Virol2002;83: 2201–2209.
- Emerson SU, Clemente-Casares P, Moiduddin N, Arankalle VA, Torian U, Purcell RH. Putative neutralization epitopes and broad cross-genotype neutralization of hepatitis E virus confirmed by a quantitative cell-culture assay. J Gen Virol2006;87: 697–704.
- Liang JH, Dai X, Dong C, Meng JH. A single amino acid substitution changes antigenicity of ORF2-encoded proteins of hepatitis E virus. Int J Mol Sci2010;11: 2962–2975.
- Tyagi S, Korkaya H, Zafrullah M, Jameel S, Lal SK. The phosphorylated form of the ORF3 protein of hepatitis E virus interacts with its non-glycosylated form of the major capsid protein, ORF2. J Biol Chem2002;277: 22759–22767.
- Tyagi S, Surjit M, Lal SK. The 41-amino-acid C-terminal region of the hepatitis E virus ORF3 protein interacts with bikunin, a kunitz-type serine protease inhibitor. J Virol2005;79: 12081–12087.
- Tyagi S, Surjit M, Roy AK, Jameel S, Lal SK. The ORF3 protein of hepatitis E virus interacts with liver-specific alpha1-microglobulin and its precursor alpha1-microglobulin/bikunin precursor (AMBP) and expedites their export from the hepatocyte. J Biol Chem2004;279: 29308–29319.
- Zafrullah M, Ozdener MH, Panda SK, Jameel S. The ORF3 protein of hepatitis E virus is a phosphoprotein that associates with the cytoskeleton. J Virol1997;71: 9045–9053.
- Panda SK, Ansari IH, Durgapal H, Agrawal S, Jameel S. The in vitro-synthesized RNA from a cDNA clone of hepatitis E virus is infectious. J Virol2000;74: 2430–2437.
- Kannan H, Fan S, Patel D, Bossis I, Zhang YJ. The hepatitis E virus open reading frame 3 product interacts with microtubules and interferes with their dynamics. J Virol2009;83: 6375–6382.
- Chandra V, Kar-Roy A, Kumari S, Mayor S, Jameel S. The hepatitis E virus ORF3 protein modulates epidermal growth factor receptor trafficking, STAT3 translocation, and the acute-phase response. J Virol2008;82: 7100–7110.
- Yamada K, Takahashi M, Hoshino Y et al.ORF3 protein of hepatitis E virus is essential for virion release from infected cells. J Gen Virol2009;90: 1880–1891.
- Takahashi M, Yamada K, Hoshino Y et al.Monoclonal antibodies raised against the ORF3 protein of hepatitis E virus (HEV) can capture HEV particles in culture supernatant and serum but not those in feces. Arch Virol2008;153: 1703–1713.
- Kar-Roy A, Korkaya H, Oberoi R, Lal SK, Jameel S. The hepatitis E virus open reading frame 3 protein activates ERK through binding and inhibition of the MAPK phosphatase. J Biol Chem2004;279: 28345–28357.
- Ratra R, Kar-Roy A, Lal SK. The ORF3 protein of hepatitis E virus interacts with hemopexin by means of its 26 amino acid N-terminal hydrophobic domain II. Biochemistry2008;47: 1957–1969.
- Korkaya H, Jameel S, Gupta D et al.The ORF3 protein of hepatitis E virus binds to Src homology 3 domains and activates MAPK. J Biol Chem2001;276: 42389–42400.
- Shimamura A, Ballif BA, Richards SA, Blenis J. Rsk1 mediates a MEK–MAP kinase cell survival signal. Curr Biol2000;10: 127–135.
- Moin SM, Chandra V, Arya R, Jameel S. The hepatitis E virus ORF3 protein stabilizes HIF-1alpha and enhances HIF-1-mediated transcriptional activity through p300/CBP. Cell Microbiol2009;11: 1409–1421.
- Ratra R, Kar-Roy A, Lal SK. ORF3 protein of hepatitis E virus interacts with the Bbeta chain of fibrinogen resulting in decreased fibrinogen secretion from HuH-7 cells. J Gen Virol2009;90: 1359–1370.
- Moin SM, Panteva M, Jameel S. The hepatitis E virus Orf3 protein protects cells from mitochondrial depolarization and death. J Biol Chem2007;282: 21124–21133.
- Chandra V, Kalia M, Hajela K, Jameel S. The ORF3 protein of hepatitis E virus delays degradation of activated growth factor receptors by interacting with CIN85 and blocking formation of the Cbl-CIN85 complex. J Virol2010;84: 3857–3867.
- Surjit M, Oberoi R, Kumar R, Lal SK. Enhanced alpha1 microglobulin secretion from hepatitis E virus ORF3-expressing human hepatoma cells is mediated by the tumor susceptibility gene 101. J Biol Chem2006;281: 8135–8142.
- Nagashima S, Takahashi M, Jirintai et al.A PSAP motif in the ORF3 protein of hepatitis E virus is necessary for virion release from infected cells. J Gen Virol2011;92: 269–278.
- Nagashima S, Takahashi M, Jirintai S et al.Tsg101 and the vacuolar protein sorting pathway are required for release of hepatitis E virions. J Gen Virol2012;92( Pt 12): 2838–248.
- Chandra V, Holla P, Ghosh D, Chakrabarti D, Padigaru M, Jameel S. The hepatitis E virus ORF3 protein regulates the expression of liver-specific genes by modulating localization of hepatocyte nuclear factor 4. PLoS One2011;6: e22412.
- Graff J, Nguyen H, Kasorndorkbua C et al.In vitro and in vivo mutational analysis of the 3′-terminal regions of hepatitis E virus genomes and replicons. J Virol2005;79: 1017–1026.
- Kumar A, Panda SK, Durgapal H, Acharya SK, Rehman S, Kar UK. Inhibition of Hepatitis E virus replication using short hairpin RNA (shRNA). Antiviral Res2010;85: 541–550.
- Sriram B, Thakral D, Panda SK. Targeted cleavage of hepatitis E virus 3′ end RNA mediated by hammerhead ribozymes inhibits viral RNA replication. Virology2003;312: 350–358.
- Li TC, Takeda N, Miyamura T et al.Essential elements of the capsid protein for self-assembly into empty virus-like particles of hepatitis E virus. J Virol2005;79: 12999–13006.
- Li TC, Yamakawa Y, Suzuki K et al.Expression and self-assembly of empty virus-like particles of hepatitis E virus. J Virol1997;71: 7207–7213.
- Li TC, Suzaki Y, Ami Y, Dhole TN, Miyamura T, Takeda N. Protection of cynomolgus monkeys against HEV infection by oral administration of recombinant hepatitis E virus-like particles. Vaccine2004;22: 370–377.
- Xing L, Kato K, Li T et al.Recombinant hepatitis E capsid protein self-assembles into a dual-domain T=1 particle presenting native virus epitopes. Virology1999;265: 35–45.
- Xing L, Wang JC, Li TC et al.Spatial configuration of hepatitis E virus antigenic domain. J Virol2011;85: 1117–1124.
- Niikura M, Takamura S, Kim G et al.Chimeric recombinant hepatitis E virus-like particles as an oral vaccine vehicle presenting foreign epitopes. Virology2002;293: 273–280.
- Chandra V, Taneja S, Kalia M, Jameel S. Molecular biology and pathogenesis of hepatitis E virus. J Biosci2008;33: 451–464.
- Jameel S. Molecular biology and pathogenesis of hepatitis E virus. Expert Rev Mol Med1999;1999: 1–16.
- Zhou Y, Emerson SU. P.302 Heat shock cognate protein 70 may mediate the entry of hepatitis E virus into host cells. J Clin Virol2006;36( Suppl 2): S155.
- He S, Miao J, Zheng Z et al.Putative receptor-binding sites of hepatitis E virus. J Gen Virol2008;89: 245–249.
- Nanda SK, Panda SK, Durgapal H, Jameel S. Detection of the negative strand of hepatitis E virus RNA in the livers of experimentally infected rhesus monkeys: evidence for viral replication. J Med Virol1994;42: 237–240.
- Meng XJ, Halbur PG, Haynes JS et al.Experimental infection of pigs with the newly identified swine hepatitis E virus (swine HEV), but not with human strains of HEV. Arch Virol1998;143: 1405–1415.
- Williams TP, Kasorndorkbua C, Halbur PG et al.Evidence of extrahepatic sites of replication of the hepatitis E virus in a swine model. J Clin Microbiol2001;39: 3040–3046.
- Yamada K, Takahashi M, Hoshino Y et al.Construction of an infectious cDNA clone of hepatitis E virus strain JE03-1760F that can propagate efficiently in cultured cells. J Gen Virol2009;90: 457–462.
- Okamoto H. Genetic variability and evolution of hepatitis E virus. Virus Res2007;127: 216–228.
- Maneerat Y, Clayson ET, Myint KS, Young GD, Innis BL. Experimental infection of the laboratory rat with the hepatitis E virus. J Med Virol1996;48: 121–128.
- Usmanov RK, Balaian MS, Dvoinikova OV et al.[An experimental infection in lambs by the hepatitis E virus.] Vopr Virusol1994;39: 165–168. Russian.
- Nicand E, Armstrong GL, Enouf V et al.Genetic heterogeneity of hepatitis E virus in Darfur, Sudan, and neighboring Chad. J Med Virol2005;77: 519–521.
- Sonoda H, Abe M, Sugimoto T et al.Prevalence of hepatitis E virus (HEV) Infection in wild boars and deer and genetic identification of a genotype 3 HEV from a boar in Japan. J Clin Microbiol2004;42: 5371–5374.
- Meng XJ, Halbur PG, Shapiro MS et al.Genetic and experimental evidence for cross-species infection by swine hepatitis E virus. J Virol1998;72: 9714–9721.
- Meng XJ, Purcell RH, Halbur PG et al.A novel virus in swine is closely related to the human hepatitis E virus. Proc Natl Acad Sci USA1997;94: 9860–9865.
- Zhao C, Ma Z, Harrison TJ et al.A novel genotype of hepatitis E virus prevalent among farmed rabbits in China. J Med Virol2009;81: 1371–1379.
- Nakamura M, Takahashi K, Taira K et al.Hepatitis E virus infection in wild mongooses of Okinawa, Japan: demonstration of anti-HEV antibodies and a full-genome nucleotide sequence. Hepatol Res2006;34: 137–140.
- Wang Y, Ling R, Erker JC et al.A divergent genotype of hepatitis E virus in Chinese patients with acute hepatitis. J Gen Virol1999;80 (Pt 1): 169–177.
- Wang Y, Ma X. [Detection and sequences analysis of sheep hepatitis E virus RNA in Xinjiang autonomous region.] Wei Sheng Wu Xue Bao2010;50: 937–941. Chinese.
- Hu GD, Ma X. [ Detection and sequences analysis of bovine hepatitis E virus RNA in Xinjiang Autonomous Region.] Bing Du Xue Bao2010;26: 27–32. Chinese.
- Feagins AR, Opriessnig T, Huang YW, Halbur PG, Meng XJ. Cross-species infection of specific-pathogen-free pigs by a genotype 4 strain of human hepatitis E virus. J Med Virol2008;80: 1379–1386.
- Huang F, Zhang W, Gong G et al.Experimental infection of Balb/c nude mice with Hepatitis E virus. BMC Infect Dis2009;9: 93.
- Haqshenas G, Shivaprasad HL, Woolcock PR, Read DH, Meng XJ. Genetic identification and characterization of a novel virus related to human hepatitis E virus from chickens with hepatitis-splenomegaly syndrome in the United States. J Gen Virol2001;82: 2449–2462.
- Zhao Q, Zhou EM, Dong SW et al.Analysis of avian hepatitis E virus from chickens, China. Emerg Infect Dis2010;16: 1469–1472.