Abstract
Pathogenic bacteria display various levels of host specificity or tropism. While many bacteria can infect a wide range of hosts, certain bacteria have strict host selectivity for humans as obligate human pathogens. Understanding the genetic and molecular basis of host specificity in pathogenic bacteria is important for understanding pathogenic mechanisms, developing better animal models and designing new strategies and therapeutics for the control of microbial diseases. The molecular mechanisms of bacterial host specificity are much less understood than those of viral pathogens, in part due to the complexity of the molecular composition and cellular structure of bacterial cells. However, important progress has been made in identifying and characterizing molecular determinants of bacterial host specificity in the last two decades. It is now clear that the host specificity of bacterial pathogens is determined by multiple molecular interactions between the pathogens and their hosts. Furthermore, certain basic principles regarding the host specificity of bacterial pathogens have emerged from the existing literature. This review focuses on selected human pathogenic bacteria and our current understanding of their host specificity.
Introduction
Host specificity or tropism of a microbial pathogen is defined by its ability to colonize (or infect) a host organism.Citation1 Pathogenic bacteria display various levels of host specificity. Certain bacteria have extremely diverse host ranges. Bacteria in this category may infect insects, humans, rodents and many other wild/domestic animals, such as Borrelia burgdorferi and Yersinia pestis (the respective etiologic agents of Lyme disease and plague). Many bacteria possess intermediate levels of host specificity. Such pathogens infect human and other mammalian species, but they may exhibit different pathogenicity between human and other hosts. For example, Salmonella Typhimurium causes gastroenteritis in humans after oral ingestion of the bacterium, but in mice, this infection route leads to symptoms resembling typhoid fever of human.Citation2,Citation3 Listeria monocytogenes can cause listeriosis in human and a number of domestic animals after oral ingestion of the bacterium. Interestingly, oral infection of L. monocytogenes leads to listeriosis-like disease in some model animals (e.g., guinea pig) but not other (e.g., mouse).Citation4,Citation5,Citation6 These important features have made S. Typhimurium and L. monocytogenes important models for studying many important aspects of bacterial pathogenesis, host immunity, host-pathogen interactions, and host specificity.Citation2,Citation3,Citation7 In sharp contrast, a number of other bacteria are highly adapted to the human environment and display strict host selectivity for humans, including Haemophilus influenzae, Helicobacter pylori, Neisseria gonorrhoeae, Neisseria meningitidis, Mycobacterium leprae, Salmonella Typhi, Streptococcus pneumoniae, Streptococcus pyogenes, Vibrio cholerae and Treponema pallidum. For the purpose of discussion in this review, we will hereafter refer to these bacteria as human-specific pathogens.
Understanding the genetic and molecular basis of host specificity in pathogenic bacteria is of great importance. First, learning how a pathogen determines its host range at the molecular level can directly enhance our knowledge of pathogenic mechanisms. Second, identifying the molecular determinants of host tropism for a pathogen provides insights that are valuable for the improvement of animal models used to simulate the human diseases caused by natural infection. Finally, defining the molecular mechanisms of bacterial host specificity can provide potential molecular targets and other clues to aid in the design of new strategies and therapeutics for the control of microbial diseases.
Host specificity of microbial pathogens is determined by elaborate molecular interactions between the pathogens and hosts. This principle is best demonstrated in viral pathogens due to the relative simplicity of their genomes and structures. The host specificity of viruses is predominantly defined by the interactions of viral proteins with their cognate cellular receptors.Citation8 The molecular mechanisms of host specificity are less understood for bacterial pathogens due to the complexity of their molecular compositions and cellular structures. However, important progress has been made toward the complete understanding of the host specificity of pathogenic bacteria over the last two decades. This review focuses on the host specificity of selected human-specific pathogens. Due to space constraints, we will not provide exhaustive coverage of the discoveries in the area of bacterial host specificity. Instead, we will attempt to uncover emerging principles in this area by focusing on several bacterial pathogens for which the host specificity has been extensively characterized.
HUMAN-SPECIFIC PATHOGENS
Pathogenic Neisseria
N. gonorrhoeae and N. meningitidis are two human pathogens within the genus Neisseria. N. gonorrhoeae is the causative agent of gonorrhea (pelvic inflammation), a sexually transmitted disease. N. meningitidis causes invasive infections, such as septicemia and meningitis. Both pathogens have strict host tropism for humans. Like many other human-specific pathogens, the narrow host specificity of these pathogenic Neisseria has made it difficult to study the pathogenic mechanisms and develop therapeutics due to the lack of appropriate animal models that simulate the clinical presentations of the human diseases.Citation9,Citation10,Citation11
The molecular basis for the host specificity of pathogenic Neisseria is not fully understood, but the existing evidence indicates that multiple factors are involved. On the pathogen side, a number of surface-exposed proteins appear to be associated with the human specificity of pathogenic Neisseria, including immunoglobulin A1 (IgA1) protease,Citation12,Citation13 type IV pili,Citation14,Citation15,Citation16 complement factor H binding proteins (FHBP),Citation17,Citation18 gonococcal porin,Citation19 transferrin-binding proteinsCitation20,Citation21 and lactoferrin-binding proteins.Citation21,Citation22 On the host side, several human proteins have been implicated as host specificity determinants, including IgA1,Citation12,Citation13 cell surface complement regulator CD46,Citation10 complement regulator factor H (FH),Citation17,Citation18,Citation23 complement regulator C4b-binding protein (C4BP),Citation19 transferrinCitation9,Citation24,Citation25 and lactoferrin. Citation24,Citation25
As illustrated in Figure , N. gonorrhoeae and N. meningitidis produce an extracellular serine-type protease that specifically cleaves human IgA1, a subclass of the dominant mucosal antibody, IgA.Citation12,Citation13 IgA1 is the most abundant antibody in upper respiratory secretions.Citation26 IgA serves as a ‘non-inflammatory mucosal protector’ at mucosal surfaces, performing multiple important functions, such as inhibition of microbial adherence, neutralization of microorganisms and inhibition of antigen penetration.Citation27 S. pneumoniae and H. influenzae, two additional obligate human pathogens and colonizers of the human nasopharynx, possess similar capabilities to cleave human IgA1 (Figure ).Citation13,Citation28,Citation29 These IgA1 proteases specifically cleave the hinge region of IgA1, but not that of IgA2 due to the sequence differences of the two IgA subclasses in this region, with 26 amino acids in IgA1 and 13 in IgA2.Citation26 Mouse and many other mammals possess only one IgA antibody with significant sequence differences from human IgA1, which explains why the IgA1 proteases of pathogenic Neisseria cannot digest the IgA produced by many nonhuman species.Citation30 Cleavage of IgA1 in the hinge region leads to the separation of the Fc region from the antigen-binding Fab fragment, abolishing the crosslinking activity and all secondary effector functions mediated by the Fc region.Citation27 The loss of IgA-mediated crosslinking and microbial agglutination may result in higher levels of bacterial adherence to mucosal surfaces and colonization.Citation13
Figure 1 Cleavage of human IgA1 by bacterial IgA1 proteases. (A) Diagrammatic illustration of human IgA1. Indicated are the hinge region (target for bacterial IgA1 proteases) and the variable and constant regions of the IgA1 light (VL and CL) and heavy (VH and CH) chains. (B) Amino-acid sequence of the human IgA1 hinge region. The cleavage site for each of the bacterial IgA1 proteases is marked by the species name of the corresponding bacterium.
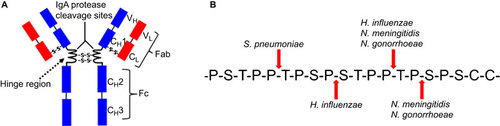
Type IV pili on the cell surface of pathogenic Neisseria mediate the initial adhesion to the mucosal epithelium through binding to human membrane cofactor protein (MCP or CD46).Citation14,Citation15,Citation16 CD46 is a widely distributed cell membrane protein, which protects the host cells from accidental damage by complement through inactivating the alternative pathway of the complement system.Citation31,Citation32 The complement system is a key component of host immunity against N. meningitidis, and complement-deficient individuals have a significantly elevated risk of developing meningococcal disease.Citation33 Previous studies have also identified CD46 as a host receptor for multiple viral and bacterial pathogens, such as measles virus,Citation34,Citation35,Citation36 herpes virus 6,Citation37 adenovirusCitation38,Citation39,Citation40,Citation41 and S. pyogenes.Citation42 The extracellular segment of CD46 consists of four tandem complement control protein (CCP) modules and one or two heavily O-glycosylated serine/threonine/proline-rich domains.Citation43 While measles virus and human herpesvirus 6 recognize the CCP1–2 and CCP2–3 modules, respectively,Citation44,Citation45,Citation46 pathogenic Neisseria and S. pyogenes bind to the serine/threonine/proline-rich and CCP3–4 regions of CD46, respectively.Citation15,Citation47
Interaction of N. meningitidis and N. gonorrhoeae with CD46 triggers signaling events in the host cells, leading to intimate attachment and bacterial entry into host cells in cell culture models.Citation14,Citation48,Citation49,Citation50 The significance of this molecular interaction in the pathogenesis of N. meningitidis has been further implicated in transgenic mice expressing human CD46.Citation10 When infected intraperitoneally with piliated N. meningitidis, CD46 transgenic mice displayed higher levels of bacteria in the bloodstream and cerebrospinal fluid; similar infection via intranasal inoculation with N. meningitidis also led to bacteremia and mortality only in CD46 transgenic mice, but not in the control mice. These in vitro and in vivo studies strongly suggest that CD46 is a host factor that contributes to the host specificity of N. meningitidis. Because the type IV pili of N. gonorrhoeae and N. meningitidis interact with CD46 in a similar manner,Citation14,Citation48,Citation49,Citation50 it is likely that this pathogen–host interaction also contributes to the host tropism of N. gonorrhoeae for humans. Transgenic mice expressing human CD46 are more susceptible to S. pyogenes infection (see below).Citation51,Citation52
Both N. gonorrhoeae and N. meningitidis are able to recruit FH to the cell surface in a human-specific fashion.Citation53,Citation54,Citation55 FH is one of the most abundant proteins in the blood (commonly present in human plasma at concentrations of 300 to 500 µg/mL). FH is composed of 20 short consensus repeats (SCRs), each containing approximately 60 amino acids.Citation56 FH inhibits the alternative pathway of the complement system by preventing the binding of factor B to C3b, enhancing the decay of the C3-convertase (C3bBb) and acting as a cofactor for the cleavage of C3b by complement factor I.Citation57 FH deposition on host tissue and cell surfaces prevents non-specific damage and avoids the wasteful consumption of complement components.Citation57 Many microbial pathogens have been shown to bind to FH as a common mechanism for the evasion of complement-mediated host immunity (Figure ).Citation58,Citation59 This is exemplified by the binding of FH to pathogenic Neisseria,Citation17,Citation18,Citation23,Citation71 Candida albicans,Citation70,Citation72 Borrelia burgdorferi,Citation68,Citation69,Citation73 H. influenzae,Citation62 S. pneumoniae,Citation63,Citation74 S. pyogenes,Citation75 S. agalactiaeCitation76,Citation77,Citation78 and Streptococcus suis.Citation79 Notably, S. pneumoniae, another resident of the human upper airway, also interacts with FH in a human-specific manner (see below).Citation80
Figure 2 Schematic illustration of the molecular interactions between microbial pathogens and negative regulator protein factor H of the alternative complement pathway. The 20 FH SCR domains are illustrated in the context of their binding specificities for N. gonorrhoeae,Citation60,Citation61 N. meningitidis,Citation55,Citation60 H. influenzae,Citation62 S. pneumoniae,Citation63,Citation64,Citation65 S. pyogenes,Citation66,Citation67 S. agalactiae,Citation63 B. burgdorferiCitation68,Citation69 and C. albicans.Citation70 The information on the FH-binding activities was derived from the relevant references cited here for the corresponding microorganisms.
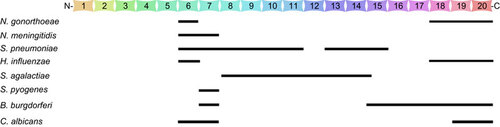
While N. gonorrhoeae binds to FH via its porin protein, a dominant component of the outer membrane,Citation17 the FH binding activity of N. meningitidis has been localized to a ∼29-kD FHBP. FHBP is expressed as a lipoprotein in the meningococcal outer membrane of all strains of N. meningitidis.Citation18,Citation81,Citation82 The recruitment of FH helps both pathogens evade complement-mediated lysis in human plasma.Citation17,Citation18,Citation23 Moreover, FH-binding activity has been shown to mediate gonococcal attachment to human complement receptor 3 on the surface of epithelial cells, suggesting that this interaction may facilitate gonococcal entry into nonprofessional phagocytes and thereby aid the evasion of host immunity.Citation83 The molecular preference of N. gonorrhoeae and N. meningitidis for human FH leads to a species-specific resistance to the bactericidal activity of human serum.Citation18,Citation23,Citation53,Citation54 Finally, the expression of human FH leads to significantly enhanced N. meningitidis bacteremia in transgenic rats.Citation84 These findings are consistent with a clinical observation that polymorphisms in the promoter of the human FH gene are associated with increased risk for meningococcal disease in UK Caucasian patients.Citation85
Prior to the discovery of its FH binding activity, the FHBP of N. meningitidis was identified as a vaccine candidate.Citation81,Citation82 Two FHBP-based vaccines have since advanced to clinical trials.Citation86 The immunization of transgenic mice expressing human FH with the natural form of FHBP led to significantly decreased protective immune responses, as assessed by serum bactericidal activity; a mutant FHBP lacking the FH-binding activity showed the opposite vaccination result.Citation87,Citation88 This finding suggests that engineering FH binding-negative FHBP vaccine antigens may enhance the immunoprotective efficacy against meningococcal disease in humans.
N. gonorrhoeae and N. meningitidis bind to C4BP via the allelic variants of the porin protein.Citation19,Citation89 C4BP is a plasma glycoprotein that downregulates the classical complement pathway.Citation32 It has been shown that N. gonorrhoeae binds only to human C4BP but not the counterparts of rodent, lagomorph and primate species.Citation53 The species-specific binding pattern with human C4BP is consistent with gonococcal resistance to human serum complement.Citation53 A number of other pathogens also recruit C4BP to evade complement-mediated immune responses, such as Bordetella pertussis,Citation90 Escherichia coli K1,Citation91 H. influenzae,Citation92 Moraxella catarrhalis,Citation93 S. pneumoniaeCitation94,Citation95 and S. pyogenes.Citation96 Interestingly, S. pneumoniae, also interacts with C4BP in a human-specific manner (see below).Citation94
Iron acquisition appears to contribute to the host specificity of N. gonorrhoeae and N. meningitidis for humans Citation25. Iron is an essential nutrient for survival for virtually all bacteria. Pathogenic Neisseria species are able to extract iron from host iron-containing proteins through specific receptors, such as transferrin, lactoferrin and hemoglobin.Citation97 N. gonorrhoeae and N. meningitidis preferentially bind to human transferrin and lactoferrin and utilize them as iron sources when compared with the transferrins and lactoferrins of other hosts.Citation25,Citation98 While the majority of transferrin circulates in human serum to deliver iron into cells and sequester free iron, lactoferrin is mostly present in phagocytic cells and in secretions such as milk, mucus and tears.Citation97 Exogenous administration of human transferrin and lactoferrin through intravenous or intraperitoneal inoculation significantly enhances meningococcal bacteremia and mortality in mice.Citation24 In addition, transgenic mice expressing human transferrin are significantly more susceptible to systemic infection by N. meningitidis.Citation9 N. meningitidis utilizes human and bovine hemoglobins at a similar level in vitro and in mice,Citation24 suggesting that the utilization of hemoglobin does not contribute significantly to the host specificity of pathogenic Neisseria for humans. In sharp contrast, the preferential binding of Staphylococcus aureus to human hemoglobin does contribute to its host specificity.Citation99 Another interesting observation is that the human-specific binding of lactoferrin by pneumococci is not a means of iron acquisition Citation100. These lines of evidence indicate that different pathogens interact with host-containing proteins for various purposes.
Streptococcus pneumoniae
S. pneumoniae is an obligate human pathogen and causes numerous infections, such as pneumonia, otitis media, meningitis and sinusitis in humans.Citation101 The molecular mechanisms behind this strict host specificity are unclear, but several human-specific pathogen–host interactions have been recently revealed using biochemical approaches.
The metallo-type IgA1 protease of S. pneumoniae cleaves the hinge region of human IgA1 but not those of the IgA molecules from many other mammalian species (Figure ).Citation28,Citation29,Citation30 As discussed above, the digestion of IgA1 by bacterial IgA1 proteases leads to the loss of the crosslinking activity and all secondary effector functions mediated by the Fc region.Citation27 S. pneumoniae IgA1 protease has been shown to enhance bacterial adherence to epithelial cells by digesting IgA1,Citation102 suggesting that this human-specific activity is a host specificity determinant of S. pneumoniae.
S. pneumoniae interacts with a list of host factors in a human-specific manner through choline-binding protein A (CbpA). CbpA, also called PspC,Citation103 is a major surface-exposed protein of S. pneumoniae.Citation104 CbpA binds to the polymeric immunoglobulin receptor (pIgR),Citation105 secretory component (SC),Citation106 secretory IgA (SIgA),Citation106 FH,Citation107,Citation108 C4BP,Citation95 sialic acid,Citation104 complement C3 proteinCitation109 and vitronectin.Citation110 The middle repeat region of CbpA is responsible for binding to domains 3 and 4 of human pIgR and the same region in human SC and SIgA.Citation105,Citation111,Citation112,Citation113 While the FH binding activity has been mapped to the amino terminal region of CbpA and its allelic variants,Citation77,Citation107,Citation114 multiple regions of human FH have been reported to bind to CbpA, including SCR 6–10,Citation65 8–14Citation63 and 13–15.Citation64 The CbpA–pIgR interaction mediates the epithelial adhesion and transmigration of S. pneumoniae;Citation105,Citation115,Citation116,Citation117 the recruitment of FH to the pneumococcal surface enhances the evasion of complement-mediated phagocytosisCitation74,Citation80,Citation107 and epithelial invasion.Citation118 Studies from our laboratory and others have demonstrated that CbpA exclusively binds to the pIgR, SC, SIgA and FH of humans but not their counterparts in common model animals (e.g., mouse, rat and rabbit).Citation80,Citation105,Citation112,Citation119 These species-specific interactions strongly suggest that CbpA is a major molecular determinant of pneumococcal host specificity.
The enolase of S. pneumoniae binds to C4BP, a negative regulator of the classical complement pathway.Citation94 This interaction leads to decreased C3b deposition on pneumococci. Interestingly, this binding occurs only with human C4BP, not mouse C4BP.Citation94 This observation is reminiscent of the human-specific recruitment of C4BP by N. gonorrhoeae, a colonizer of mucosal surfaces in humans.Citation53 Thus, evasion of complement-mediated immunity appears to be a common mechanism underlying the host specificity of many pathogenic bacteria that colonize the mucosal surfaces of humans.
Pneumococcal surface protein A (PspA) is another protein that may contribute to the host tropism of S. pneumoniae. PspA is a major surface-exposed protein and a protective antigen of S. pneumoniae.Citation120 Previous biochemical studies have shown that the C-terminal region of PspA binds to human lactoferrin, an iron-sequestering glycoprotein (predominately in mucosal secretions).Citation121 Unlike pathogenic Neisseria, S. pneumoniae cannot obtain iron from lactoferrin.Citation122 Instead, PspA binding to lactoferrin protects pneumococci from killing by apolactoferrin, a form of lactoferrin that does not carry iron.Citation123,Citation124 Interestingly, this interaction is human specific because PspA does not bind to bovine lactoferrin,Citation100 suggesting that the PspA–lactoferrin interaction contributes to the host specificity of S. pneumoniae.
These species-specific pathogen–host interactions correlate very well with the host specificity of S. pneumoniae. It is thus tempting to conclude that host specificity of S. pneumoniae is defined by multiple molecular determinants. Among the bacterial factors, CbpA mediates multiple molecular species-specific interactions with human factors (e.g., pIgR, SC, SIgA and FH). The lack of appropriate animal models has been a major challenge for determining the biological impacts of these pathogen–host interactions on the host specificity and pathogenesis of S. pneumoniae. However, as exemplified in L. monocytogenes,Citation125,Citation126 these biochemical findings have provided critical molecular targets to establish genetically modified (humanized) animals and/or (murinized) pneumococci. These tools will be important for further elucidating the mechanisms of pneumococcal host specificity and evaluating new therapeutics and vaccines for the control of pneumococcal disease.
Streptococcus pyogenes
S. pyogenes (group A streptococcus) is an obligate human pathogen that causes pharyngitis (strep throat), localized skin infections, scarlet fever, streptococcal toxic shock syndrome and autoimmune-mediated complications.Citation127 Although the molecular basis for the human specificity of S. pyogenes remains unclear, a study reported by Sun et al.Citation128 indicates that the streptokinase secreted by this bacterium is an important determinant of its host specificity. Streptokinase is able to bind to and activate human plasminogen, a blood clot-dissolving protein, but not the plasminogens from other mammalian species.Citation129 The plasminogen-activating activity of streptokinase has been implicated in the accelerated clearance of host fibrin, which may promote the dissemination of S. pyogenes in host tissues.Citation128,Citation130 Mice typically possess high resistance to skin infection by S. pyogenes, but Sun et al.Citation128 showed that the expression of human plasminogen in mice led to markedly increased mortality in the transgenic mice when infected with streptococci.
The molecular interaction of M protein with CD46 also contributes to the host specificity of S. pyogenes.Citation42 The M protein of S. pyogenes is a major surface-exposed protein and one of its most important virulence factors.Citation131 The specific interaction between M protein and human CD46 mediates streptococcal binding to keratinocytes Citation42,Citation132 and the bacterial invasion of epithelial cells.Citation133 Transgenic mice expressing human CD46 are more susceptible to streptococcal disease.Citation51,Citation52 In a systemic infection model, transgenic mice expressing human CD46 displayed higher levels of bacteremia, arthritis and mortality compared with the non-transgenic mice.Citation51 Matsui et al.Citation52 corroborated this finding in a subcutaneous infection model, in which the CD46 transgenic mice exhibited more severe forms of bacterial growth in deep tissues, necrotizing fasciitis at the infection sites (footpads) and mortality. These studies indicate that M protein is a molecular determinant of the host specificity of S. pyogenes through the targeting of human CD46.
S. pyogenes M protein also interacts with a wide range of other host factors, such as the complement regulator proteins C4BPCitation134 and FH,Citation75 as well as fibrinogenCitation135,Citation136 and IgG.Citation137,Citation138 S. pyogenes interactions with C4BP and FH are associated with bacterial evasion of complement-mediated immunity.Citation130 It remains to be determined whether these pathogen–host interactions also contribute to the host specificity of this pathogen.
OTHER PATHOGENIC BACTERIA
Gram-positive bacteria
Host specificity has been studied to various extents in other pathogenic bacteria. Among them, L. monocytogenes is the best-characterized bacterial pathogen in terms of our knowledge of the molecular mechanisms of its host specificity. L. monocytogenes is a facultative intracellular bacterium that causes foodborne diseases in humans and domestic animals. Infection occurs when ingested bacteria cross the intestinal barrier and disseminate to various organs and the bloodstream, leading to listeriosis. However, many laboratory animals do not develop typical listeriosis through the oral ingestion of L. monocytogenes, such as mouse, rat, rabbit and guinea pig.Citation4,Citation5,Citation6 Previous studies have revealed multiple molecular interactions that can now explain the host restriction of L. monocytogenes, as discussed in several excellent reviews.Citation125,Citation139,Citation140
The host selectivity of L. monocytogenes depends on two surface-exposed bacterial proteins, internalin A (InlA) and internalin B (InlB). InlA and InlB promote the entry of L. monocytogenes into nonphagocytic cells by interacting with their cognate cellular receptors. InlA binds to E-cadherin, a major cell–cell adhesion glycoprotein;Citation141,Citation142 this interaction occurs with human E-cadherin but not the mouse counterpart due to a single amino-acid difference between the human and mouse E-cadherin proteins.Citation143 The importance of the InlA–E-cadherin interaction to the host specificity of L. monocytogenes has been demonstrated in mice expressing human E-cadherin,Citation144 ‘humanized’ mouse E-cadherinCitation6 and the ‘murinized’ InlA of L. monocytogenes.Citation126 The local expression of human E-cadherin in the small intestine of mice was shown to lead to InlA-dependent bacterial invasion of enterocytes, dissemination across the intestinal barrier and significantly higher mortality following oral infection of the transgenic mice with L. monocytogenes.Citation144 Based on the structural knowledge of the InlA–human E-cadherin complex,Citation141 Wollert et al.Citation126 recently constructed a recombinant InlA that could interact with mouse E-cadherin by introducing two sequence substitutions in InlA, leading to dramatic enhancement in its binding affinity for mouse E-cadherin. The ‘murinized’ L. monocytogenes expressing the engineered InlA was able to induce typical listeriosis in wild-type mice by oral infection.Citation126
InlB is also a host specificity determinant of L. monocytogenes.Citation5 It is essential for the entry of L. monocytogenes into hepatocytes and some epithelial and fibroblast cell lines.Citation145,Citation146,Citation147 Three different host receptors have been reported to interact with InlB: C1q-binding protein gC1q-R/p32,Citation148 Met (the receptor for hepatocyte growth factor)Citation149 and glycosaminoglycans.Citation150 InlB interacts with Met in a species-specific manner because it binds to the Met proteins of human and mouse but not those of rabbit and guinea pig.Citation5 Consistently, only the Met proteins from human and mouse support the InlB-dependent entry of L. monocytogenes into mammalian cells; the InlB-deficient mutant does not show attenuated virulence in guinea pigs and rabbits. Among the common model animals, the gerbil is the only species in which both InlA/E–cadherin and InlB–Met interactions are functional.Citation6 In agreement, the oral infection of gerbils with L. monocytogenes leads to typical listeriosis.Citation6,Citation151 These advancements have not only explained the pathogenic mechanisms of L. monocytogenes, but have also laid a new foundation for the rational design of better small animal models in studying human infections caused by this pathogen.Citation151
S. aureus captures hemoglobin for heme-iron acquisition through the hemoglobin-binding protein IsdB.Citation152 Pishchany et al.Citation99 showed preferential binding of S. aureus to human hemoglobin when compared with murine hemoglobin. Consistently, human hemoglobin-expressing transgenic mice were significantly more susceptible to systemic infection by S. aureus, suggesting that iron availability in human contributes to the host specificity of S. aureus.Citation99 Preferential binding to human hemoglobin is also observed with other primarily human-associated bacteria, including Staphylococcus lugdunensis, Staphylococcus simulans and Corynebacterium diphtheriae.Citation99
Gram-negative bacteria
Salmonella enterica can cause a wide spectrum of diseases in many hosts. Different serovars of S. enterica display either a restricted host range (e.g., S. Typhi for human, S. Dublin for cattle, swine and human) or a broad host range (e.g., S. Enteritidis and S. Typhimurium for human and many other hosts). The mechanisms of Salmonella host adaptation and specificity have been exclusively discussed in two recent excellent reviews.Citation2,Citation3 The major factors that affect the host specificity of Salmonella serovars include differences in the bacteria themselves (e.g., the ability to survive host immunity, to grow in a given host environment, and to transmit to other individuals of the same host species) and host environments (e.g., pH, temperature, immune recognition and response, and the microbiota of a given host).Citation153,Citation154
Pathogenic E. coli strains can cause numerous intestinal or extraintestinal infections. E. coli strains from human and domestic animals/birds often display host specificity.Citation155 The host specificity of pathogenic E. coli can be influenced by the molecular interactions of bacterial surface-exposed proteins with host cellular receptors.Citation155,Citation156,Citation157,Citation158 Septicemic E. coli strains from chickens and lambs express host-specific adhesins, such as avian-specific AC/I pili and lamb-specific K99 fimbriae.Citation155,Citation156,Citation157 Stromberg et al.Citation158 have demonstrated that the distinct recognition of Galα 1-4Gal-containing glycolipid receptors on host cells contributes to the host specificity of uropathogenic E. coli.
EMERGING PRINCIPLES
In contrast to viruses, bacteria clearly depend on much more complex molecular interactions with their natural hosts to determine host specificity. For this reason, the existing information is not sufficient to paint a full picture for the molecular mechanisms of bacterial host specificity, even for the most extensively studied bacterial pathogens (e.g., L. monocytogenes and pathogenic Neisseria). However, several principles have emerged from previous studies. As illustrated in Figure , the strong selectivity for humans of bacterial pathogens can be explained by the following: (i) specific recognition of a human receptor by pathogens for colonization and/or dissemination; (ii) specialized ability to evade or overcome immune mechanism(s) for bacterial survival; and (iii) availability of an essential nutrient for bacterial growth in humans.
Figure 3 Major requirements for successful infection of human hosts by pathogenic bacteria. (A) Once bacteria enter the host, they need to adhere to mucosal surfaces by recognizing specific receptors on host cells. (B) The bacteria must be able to evade host immune mechanisms for survival (e.g., complement system, antibody) and acquire the necessary nutrients for the growth and expansion of the population (e.g., iron). (C) Once the population size has expanded to a certain level, bacteria can disseminate to other tissues/organs from the initial infection site by intracellular invasion through molecular interactions with host receptors or other means. (D) Some bacteria may be released into the surrounding environments and transmitted to another human host.
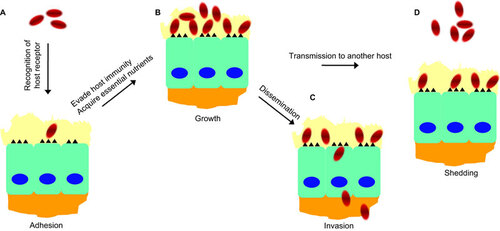
Colonization and dissemination
Certain surface-associated or secreted molecules contribute to host specificity by promoting bacterial colonization and/or dissemination. As well-characterized examples for bacterial adhesion and colonization, the pili of pathogenic NeisseriaCitation14,Citation15,Citation16 and E. coliCitation155,Citation156,Citation157,Citation158 mediate bacterial adherence to mucosal epithelia in a host-specific manner. The surface proteins of S. pneumoniae (CbpA) and S. pyogenes (M protein) bind to their human receptors for mucosal adhesion and dissemination.Citation42,Citation105 The surface-exposed proteins InlA and InlB of L. monocytogenes promote bacterial invasion across cellular/tissue barriers for dissemination into remote sites ().Citation5 The streptokinase secreted by S. pyogenes promotes bacterial dissemination by fibrinolysis through interacting with human plasminogen.Citation129
Table 1 Species-specific molecular interactions between bacteria and hosts
Immune evasion
Evasion of host immune mechanisms, particularly complement- and IgA1-mediated immunity, is a common mechanism underlying the host specificity of many mucosal colonizers in humans. This is exemplified by the host-specific interactions with human C4BP, a negative regulator of the classical complement pathway, of many bacteria such as N. gonorrhoeae,Citation19 N. meningitidis,Citation89 S. pyogenesCitation134 and S. pneumoniae.Citation94,Citation95 Similarly, many pathogens interact with negative regulators of the alternative complement pathway CD46 Citation14,Citation42 and FHCitation53,Citation54,Citation80 in a human-specific manner. Overcoming human IgA1-mediated immunity by bacterial proteases is a common mechanism underlying the host specificity of bacteria that naturally colonize the mucosal surfaces of the reproductive tract (e.g., N. gonorrhoeae) or the upper airway (e.g., S. pneumoniae, N. meningitidis and H. influenzae) ().Citation13 These lines of evidence imply that complement- and IgA-mediated immunity is crucial for host defense against these pathogens in non-permissive hosts other than humans.
Nutrient acquisition
Bacterial preference for host-specific nutrients is another common mechanism underlying host specificity. Although the precise nutrient requirements of individual bacteria in their hosts are complex, iron is one essential nutrient for bacterial growth in vivo.Citation97 Many bacteria acquire iron from host iron-containing proteins by expressing specific receptors for these host proteins.Citation97 Thus, preferential interactions of pathogenic bacteria with human iron-containing proteins have been demonstrated to contribute to the host specificity of N. meningitidis (transferrin and lactoferrin),Citation159 N. gonorrhoeae (transferrin)Citation159 and S. aureus (hemoglobin) ().Citation99,Citation152 Similarly, PspA-mediated binding to lactoferrin contributes to the human tropism of S. pneumoniae, although this notion remains to be validated by in vivo studies.
PERSPECTIVES
Recent technologic advancements in the transgenic expression of human genes in model animals have led to the generation of many ‘humanized’ mouse strains for the study of human-specific infectious diseases.Citation160 As discussed above, some of the transgenic animals have been used to evaluate the contributions of species-specific biochemical interactions to the host specificity of individual bacterial pathogens. These include mouse strains expressing human E-cadherin for L. monocytogenes,Citation144 human plasminogen for S. pyogenes,Citation128 human CD46 for N. meningitidisCitation10 and S. pyogenes,Citation51,Citation52 human transferrin for N. meningitidis,Citation9 and rats expressing human FH for N. meningitidis.Citation84 Furthermore, as exemplified in L. monocytogenes,Citation126 the increasing availability of structural information on many pathogen–host molecular interactions has made it possible to engineer murinized bacterial strains that can simulate human disease in mice. Therefore, the revelations regarding species-specific pathogen–host interactions in previous studies have already established a foundation for the establishment of genetically engineered animals and bacterial strains for studying the host specificity and pathogenesis of obligate human pathogens in the future.
Finally, insightful information on the molecular mechanisms underlying bacterial host specificity may be applied to the control of human infections caused by bacterial pathogens. For example, the host specificity determinants of bacterial pathogens may be targeted for therapeutic and vaccine development in the future because these proteins tend to be highly conserved within individual bacteria. This is exemplified by the recent development of meningococcal vaccines based on the FH-binding protein of N. meningitidis. In this case, selectively inactivating the FH-binding activity of the FH-binding protein led to enhanced immunoprotective efficacy of the vaccine.Citation86,Citation87,Citation88 Because the CbpA, PspA and IgA1 proteases are among the protein antigens that are immunoprotective against S. pneumoniae infections,Citation161,Citation162 selective mutations in the functional sites of these proteins may be used to improve the protective efficacies of these proteins as vaccine candidates.
Jing-Ren Zhang is supported by grants from the Ministry of Science and Technology of China (973, grant NO 2012CB518702), the Tsinghua University Collaborative Research Program (grant NO 2011Z23153), the Center for Marine Medicine and Rescue of Tsinghua University (grant NO 20124812029) and the Grand Challenges Exploration of the Bill and Melinda Gates Foundation (grant NO OPP1021992).
- Kirzinger MW, Stavrinides J.Host specificity determinants as a genetic continuum. Trends Microbiol2012;20: 88–93.
- Baumler A, Fang FC.Host specificity of bacterial pathogens. Cold Spring Harb Perspect Med2013;3: a010041.
- Foley SL, Johnson TJ, Ricke SC, Nayak R, Danzeisen J.Salmonella pathogenicity and host adaptation in chicken-associated serovars. Microbiol Mol Biol Rev2013;77: 582–607.
- Lecuit M, Cossart P.Genetically-modified-animal models for human infections: the Listeria paradigm. Trends Mol Med2002;8: 537–542.
- Khelef N, Lecuit M, Bierne H, Cossart P.Species specificity of the Listeria monocytogenes InlB protein. Cell Microbiol2006;8: 457–470.
- Disson O, Grayo S, Huillet E et al.Conjugated action of two species-specific invasion proteins for fetoplacental listeriosis. Nature2008;455: 1114–1118.
- Pizarro-Cerdá J, Kühbacher A, Cossart P.Entry of Listeria monocytogenes in mammalian epithelial cells: an updated view. Cold Spring Harb Perspect Med2012;2.
- Medina RA, Garcia-Sastre A.Influenza A viruses: new research developments. Nat Rev Microbiol2011;9: 590–603.
- Zarantonelli ML, Szatanik M, Giorgini D et al.Transgenic mice expressing human transferrin as a model for meningococcal infection. Infect Immun2007;75: 5609–5614.
- Johansson L, Rytkonen A, Bergman P et al.CD46 in meningococcal disease. Science2003;301: 373–375.
- Arko RJ.Animal models for pathogenic Neisseria species. Clin Microbiol Rev1989;2 Suppl: S56–S59.
- Plaut AG, Gilbert JV, Artenstein MS, Capra JD.Neisseria gonorrhoeae and Neisseria meningitidis: extracellular enzyme cleaves human immunoglobulin A. Science1975;190: 1103–1105.
- Kilian M, Russell MW.Microbial evasion of IgA functions.In: Mestecky J, LammME, Strober W, Bienenstock J, McGhee J R, Mayer L (ed.) Mucosal immunology.San Diego, CA: Academic Press, 2005: 291–303.
- Kallstrom H, Liszewski MK, Atkinson JP, Jonsson AB.Membrane cofactor protein (MCP or CD46) is a cellular pilus receptor for pathogenic Neisseria. Mol Microbiol1997;25: 639–647.
- Kallstrom H, Blackmer Gill D, Albiger B, Liszewski MK, Atkinson JP, Jonsson AB.Attachment of Neisseria gonorrhoeae to the cellular pilus receptor CD46: identification of domains important for bacterial adherence. Cell Microbiol2001;3: 133–143.
- Cattaneo R.Four viruses, two bacteria, and one receptor: membrane cofactor protein (CD46) as pathogens’ magnet. J Virol2004;78: 4385–4388.
- Ram S, McQuillen DP, Gulati S, Elkins C, Pangburn MK, Rice PA.Binding of complement factor H to loop 5 of porin protein 1A: a molecular mechanism of serum resistance of nonsialylated Neisseria gonorrhoeae. J Exp Med1998;188: 671–680.
- Madico G, Welsch JA, Lewis LA et al.The meningococcal vaccine candidate GNA1870 binds the complement regulatory protein factor H and enhances serum resistance. J Immunol2006;177: 501–510.
- Ram S, Cullinane M, Blom AM et al.Binding of C4b-binding protein to porin: a molecular mechanism of serum resistance of Neisseria gonorrhoeae. J Exp Med2001;193: 281–295.
- Schryvers AB, Morris LJ.Identification and characterization of the transferrin receptor from Neisseria meningitidis. Mol Microbiol1988;2: 281–288.
- Cornelissen CN, Hollander A.TonB-dependent transporters expressed by Neisseria gonorrhoeae. Front Microbiol2011;2: 117.
- Schryvers AB, Morris LJ.Identification and characterization of the human lactoferrin-binding protein from Neisseria meningitidis. Infect Immun1988;56: 1144–1149.
- Schneider MC, Exley RM, Chan H et al.Functional significance of factor H binding to Neisseria meningitidis. J Immunol2006;176: 7566–7575.
- Schryvers AB, Gonzalez GC.Comparison of the abilities of different protein sources of iron to enhance Neisseria meningitidis infection in mice. Infect Immun1989;57: 2425–2429.
- Lee BC, Schryvers AB.Specificity of the lactoferrin and transferrin receptors in Neisseria gonorrhoeae. Mol Microbiol1988;2: 827–829.
- Mestecky J, Moro I, Kerr MA, Woof JM.Mucosal immunoglobulins.In: Mestecky J, LammME, Strober W, Bienenstock J, McGhee JR, Mayer L (ed.) Mucosal immunology.San Diego, CA: Academic Press, 2005: 153–181.
- Russell MW, Kilian M.Biological activities of IgA.In: Mestecky J, Lamm ME, Strober W, Bienenstock J, McGhee JR, Mayer L (ed.) Mucosal immunology.San Diego, CA: Academic Press, 2005: 267–289.
- Kilian M, Mestecky J, Schrohenloher RE.Pathogenic species of the genus Haemophilus and Streptococcus pneumoniae produce immunoglobulin A1 protease. Infect Immun1979;26: 143–149.
- Male CJ.Immunoglobulin A1 protease production by Haemophilus influenzae and Streptococcus pneumoniae. Infect Immun1979;26: 254–261.
- Reinholdt J, Kilian M.Lack of cleavage of immunoglobulin A (IgA) from rhesus monkeys by bacterial IgA1 proteases. Infect Immun1991;59: 2219–2221.
- Riley-Vargas RC, Gill DB, Kemper C, Liszewski MK, Atkinson JP.CD46: expanding beyond complement regulation. Trends Immunol2004;25: 496–503.
- Prodinger WM, Wurzner R, Stoiber H, Dierich MP.Complement.In: Paul WE (ed.) Fundamental immunology.6th ed.Philadephia, PA: Lippincott-Raven Publishers, 2012: 1077–1104.
- Apicella MA. Neisseria meningitidis.In: Mandell GL, Bennett JE. Dolin RD (ed.) Principles and practice of infectious diseases.7th ed.New York: Elsevier Churchill Livingstone, 2010: 2737–2752.
- Manchester M, Liszewski MK, Atkinson JP, Oldstone MB.Multiple isoforms of CD46 (membrane cofactor protein) serve as receptors for measles virus. Proc Natl Acad Sci USA1994;91: 2161–2165.
- Naniche D, Varior-Krishnan G, Cervoni F et al.Human membrane cofactor protein (CD46) acts as a cellular receptor for measles virus. J Virol1993;67: 6025–6032.
- Dorig RE, Marcil A, Chopra A, Richardson CD.The human CD46 molecule is a receptor for measles virus (Edmonston strain). Cell1993;75: 295–305.
- Santoro F, Kennedy PE, Locatelli G, Malnati MS, Berger EA, Lusso P.CD46 is a cellular receptor for human herpesvirus 6. Cell1999;99: 817–827.
- Sirena D, Lilienfeld B, Eisenhut M et al.The human membrane cofactor CD46 is a receptor for species B adenovirus serotype 3. J Virol2004;78: 4454–4462.
- Roelvink PW, Lizonova A, Lee JG et al.The coxsackievirus-adenovirus receptor protein can function as a cellular attachment protein for adenovirus serotypes from subgroups A, C, D, E, and F. J Virol1998;72: 7909–7915.
- Wu E, Trauger SA, Pache L et al.Membrane cofactor protein is a receptor for adenoviruses associated with epidemic keratoconjunctivitis. J Virol2004;78: 3897–3905.
- Gaggar A, Shayakhmetov DM, Lieber A.CD46 is a cellular receptor for group B adenoviruses. Nat Med2003;9: 1408–1412.
- Okada N, Liszewski MK, Atkinson JP, Caparon M.Membrane cofactor protein (CD46) is a keratinocyte receptor for the M protein of the group A streptococcus. Proc Natl Acad Sci USA1995;92: 2489–2493.
- Kirkitadze MD, Barlow PN.Structure and flexibility of the multiple domain proteins that regulate complement activation. Immunol Rev2001;180: 146–161.
- Buchholz CJ, Koller D, Devaux P et al.Mapping of the primary binding site of measles virus to its receptor CD46. J Biol Chem1997;272: 22072–22079.
- Mori Y, Seya T, Huang HL, Akkapaiboon P, Dhepakson P, Yamanishi K.Human herpesvirus 6 variant A but not variant B induces fusion from without in a variety of human cells through a human herpesvirus 6 entry receptor, CD46. J Virol2002;76: 6750–6761.
- Greenstone HL, Santoro F, Lusso P, Berger EA.Human herpesvirus 6 and measles virus employ distinct CD46 domains for receptor function. J Biol Chem2002;277: 39112–39118.
- Giannakis E, Jokiranta TS, Ormsby RJ et al.Identification of the streptococcal M protein binding site on membrane cofactor protein (CD46). J Immunol2002;168: 4585–4592.
- Lee SW, Bonnah RA, Higashi DL, Atkinson JP, Milgram SL, So M.CD46 is phosphorylated at tyrosine 354 upon infection of epithelial cells by Neisseria gonorrhoeae. J Cell Biol2002;156: 951–957.
- Ayala BP, Vasquez B, Clary S, Tainer JA, Rodland K, So M.The pilus-induced Ca2+ flux triggers lysosome exocytosis and increases the amount of Lamp1 accessible to Neisseria IgA1 protease. Cell Microbiol2001;3: 265–275.
- Merz AJ, So M.Interactions of pathogenic Neisseriae with epithelial cell membranes. Annu Rev Cell Dev Biol2000;16: 423–457.
- Lovkvist L, Sjolinder H, Wehelie R et al.CD46 contributes to the severity of group A streptococcal infection. Infect Immun2008;76: 3951–3958.
- Matsui H, Sekiya Y, Nakamura M et al.CD46 transgenic mouse model of necrotizing fasciitis caused by Streptococcus pyogenes infection. Infect Immun2009;77: 4806–4814.
- Ngampasutadol J, Ram S, Blom AM et al.Human C4b-binding protein selectively interacts with Neisseria gonorrhoeae and results in species-specific infection. Proc Natl Acad Sci USA2005;102: 17142–17147.
- Granoff DM, Welsch JA, Ram S.Binding of complement factor H (fH) to Neisseria meningitidis is specific for human fH and inhibits complement activation by rat and rabbit sera. Infect Immun2009;77: 764–769.
- Schneider MC, Prosser BE, Caesar JJ et al.Neisseria meningitidis recruits factor H using protein mimicry of host carbohydrates. Nature2009;458: 890–893.
- Rodriguez de Cordoba S, Esparza-Gordillo J, Goicoechea de Jorge E, Lopez-Trascasa M, Sanchez-Corral P.The human complement factor H: functional roles, genetic variations and disease associations. Mol Immunol2004;41: 355–367.
- Prodinger WM, Wurzner R, Erdei A, Dierich MP.Complement.In: Paul WE (ed.) Fundamental immunology.4th ed.Philadephia, PA: Lippincott-Raven Publishers, 1999: 967–995.
- Jozsi M, Zipfel PF.Factor H family proteins and human diseases. Trends Immunol2008;29: 380–387.
- Meri T, Amdahl H, Lehtinen MJ et al.Microbes bind complement inhibitor factor H via a common site. PLoS Pathog2013;9: e1003308.
- Shaughnessy J, Lewis LA, Jarva H, Ram S.Functional comparison of the binding of factor H short consensus repeat 6 (SCR 6) to factor H binding protein from Neisseria meningitidis and the binding of factor H SCR 18 to 20 to Neisseria gonorrhoeae porin. Infect Immun2009;77: 2094–2103.
- Ngampasutadol J, Ram S, Gulati S, Agarwal S, Li C, Visintin A, et al.Human factor H interacts selectively with Neisseria gonorrhoeae and results in species-specific complement evasion. J Immunol2008;180: 3426–3435.
- Hallstrom T, Zipfel PF, Blom AM, Lauer N, Forsgren A, Riesbeck K.Haemophilus influenzae interacts with the human complement inhibitor factor H. J Immunol2008;181: 537–545.
- Jarva H, Janulczyk R, Hellwage J, Zipfel PF, Bjorck L, Meri S.Streptococcus pneumoniae evades complement attack and opsonophagocytosis by expressing the pspC locus-encoded Hic protein that binds to short consensus repeats 8–11 of factor H. J Immunol2002;168: 1886–1894.
- Duthy TG, Ormsby RJ, Giannakis E et al.The human complement regulator factor H binds pneumococcal surface protein PspC via short consensus repeats 13 to 15. Infection and immunity2002;70: 5604–5611.
- Dave S, Carmicle S, Hammerschmidt S, Pangburn MK, McDaniel LS.Dual roles of PspC, a surface protein of Streptococcus pneumoniae, in binding human secretory IgA and factor H. J Immunol2004;173: 471–477.
- Kotarsky H, Hellwage J, Johnsson E et al.Identification of a domain in human factor H and factor H-like protein-1 required for the interaction with streptococcal M proteins. J Immunol1998;160: 3349–3354.
- Blackmore TK, Fischetti VA, Sadlon TA, Ward HM, Gordon DL.M protein of the group A Streptococcus binds to the seventh short consensus repeat of human complement factor H. Infect Immun1998;66: 1427–1431.
- Hartmann K, Corvey C, Skerka C et al.Functional characterization of BbCRASP-2, a distinct outer membrane protein of Borrelia burgdorferi that binds host complement regulators factor H and FHL-1. Mol Microbiol2006;61: 1220–1236.
- Hellwage J, Meri T, Heikkila T et al.The complement regulator factor H binds to the surface protein OspE of Borrelia burgdorferi. J Biol Chem2001;276: 8427–8435.
- Meri T, Hartmann A, Lenk D et al.The yeast Candida albicans binds complement regulators factor H and FHL-1. Infect Immun2002;70: 5185–5192.
- Ram S, Sharma AK, Simpson SD et al.A novel sialic acid binding site on factor H mediates serum resistance of sialylated Neisseria gonorrhoeae. J Exp Med1998;187: 743–752.
- Luo S, Hoffmann R, Skerka C, Zipfel PF.Glycerol-3-phosphate dehydrogenase 2 is a novel factor H-, factor H-like protein 1-, and plasminogen-binding surface protein of Candida albicans. J Infect Dis2013;207: 594–603.
- Kraiczy P, Skerka C, Kirschfink M, Brade V, Zipfel PF.Immune evasion of Borrelia burgdorferi by acquisition of human complement regulators FHL-1/reconectin and factor H. Eur J Immunol2001;31: 1674–1684.
- Neeleman C, Geelen SP, Aerts PC et al.Resistance to both complement activation and phagocytosis in type 3 pneumococci is mediated by the binding of complement regulatory protein factor H. Infect Immun1999;67: 4517–4524.
- Horstmann RD, Sievertsen HJ, Knobloch J, Fischetti VA.Antiphagocytic activity of streptococcal M protein: selective binding of complement control protein factor H. Proc Natl Acad Sci USA1988;85: 1657–1661.
- Areschoug T, Stalhammar-Carlemalm M, Karlsson I, Lindahl G.Streptococcal beta protein has separate binding sites for human factor H and IgA-Fc. J Biol Chem2002;277: 12642–12648.
- Jarva H, Hellwage J, Jokiranta TS, Lehtinen MJ, Zipfel PF, Meri S.The group B streptococcal beta and pneumococcal Hic proteins are structurally related immune evasion molecules that bind the complement inhibitor factor H in an analogous fashion. J Immunol2004;172: 3111–3118.
- Maruvada R, Prasadarao NV, Rubens CE.Acquisition of factor H by a novel surface protein on group B Streptococcus promotes complement degradation. FASEB J2009;23: 3967–3977.
- Pian Y, Gan S, Wang S et al.Fhb, a novel factor H-binding surface protein, contributes to the antiphagocytic ability and virulence of Streptococcus suis. Infect Immun2012;80: 2402–2413.
- Lu L, Ma Z, Jokiranta TS, Whitney AR, DeLeo FR, Zhang JR.Species-specific interaction of Streptococcus pneumoniae with human complement factor H. J Immunol2008;181: 7138–7146.
- Fletcher LD, Bernfield L, Barniak V et al.Vaccine potential of the Neisseria meningitidis 2086 lipoprotein. Infect Immun2004;72: 2088–2100.
- Masignani V, Comanducci M, Giuliani MM et al.Vaccination against Neisseria meningitidis using three variants of the lipoprotein GNA1870. J Exp Med2003;197: 789–799.
- Agarwal S, Ram S, Ngampasutadol J, Gulati S, Zipfel PF, Rice PA.Factor H facilitates adherence of Neisseria gonorrhoeae to complement receptor 3 on eukaryotic cells. J Immunol2010;185: 4344–4353.
- Vu DM, Shaughnessy J, Lewis LA, Ram S, Rice PA, Granoff DM.Enhanced bacteremia in human factor H transgenic rats infected by Neisseria meningitidis. Infect Immun2012;80: 643–650.
- Haralambous E, Dolly SO, Hibberd ML et al.Factor H, a regulator of complement activity, is a major determinant of meningococcal disease susceptibility in UK Caucasian patients. Scand J Infect Dis2006;38: 764–771.
- McNeil LK, Zagursky RJ, Lin SL et al.Role of factor H binding protein in Neisseria meningitidis virulence and its potential as a vaccine candidate to broadly protect against meningococcal disease. Microbiol Mol Biol Rev2013;77: 234–252.
- Beernink PT, Shaughnessy J, Braga EM et al.A meningococcal factor H binding protein mutant that eliminates factor H binding enhances protective antibody responses to vaccination. J Immunol2011;186: 3606–3614.
- Beernink PT, Shaughnessy J, Pajon R, Braga EM, Ram S, Granoff DM.The effect of human factor H on immunogenicity of meningococcal native outer membrane vesicle vaccines with over-expressed factor H binding protein. PLoS Pathog2012;8: e1002688.
- Jarva H, Ram S, Vogel U, Blom AM, Meri S.Binding of the complement inhibitor C4bp to serogroup B Neisseria meningitidis. J Immunol2005;174: 6299–6307.
- Berggard K, Lindahl G, Dahlback B, Blom AM.Bordetella pertussis binds to human C4b-binding protein (C4BP) at a site similar to that used by the natural ligand C4b. Eur J Immunol2001;31: 2771–2780.
- Prasadarao NV, Blom AM, Villoutreix BO, Linsangan LC.A novel interaction of outer membrane protein A with C4b binding protein mediates serum resistance of Escherichia coli K1. The Journal of Immunology2002;169: 6352–6360.
- Hallstrom T, Jarva H, Riesbeck K, Blom AM.Interaction with C4b-binding protein contributes to nontypeable Haemophilus influenzae serum resistance. J Immunol2007;178: 6359–6366.
- Nordström T, Blom AM, Forsgren A, Riesbeck K.The emerging pathogen moraxella catarrhalis interacts with complement inhibitor C4b binding protein through ubiquitous surface proteins A1 and A2. J Immunol2004;173: 4598–4606.
- Agarwal S, Ferreira VP, Cortes C, Pangburn MK, Rice PA, Ram S.An evaluation of the role of properdin in alternative pathway activation on Neisseria meningitidis and Neisseria gonorrhoeae. J Immunol2010;185: 507–516.
- Dieudonne-Vatran A, Krentz S, Blom AM et al.Clinical isolates of Streptococcus pneumoniae bind the complement inhibitor C4b-binding protein in a PspC allele-dependent fashion. J Immunol2009;182: 7865–7877.
- Berggard K, Johnsson E, Morfeldt E, Persson J, Stalhammar-Carlemalm M, Lindahl G.Binding of human C4BP to the hypervariable region of M protein: a molecular mechanism of phagocytosis resistance in Streptococcus pyogenes. Mol Microbiol2001;42: 539–551.
- Parrow NL, Fleming RE, Minnick MF.Sequestration and scavenging of iron in infection. Infect Immun2013;81: 3503–3514.
- Schryvers AB, Gonzalez GC.Receptors for transferrin in pathogenic bacteria are specific for the host’s protein. Can J Microbiol1990;36: 145–147.
- Pishchany G, McCoy AL, Torres VJ et al.Specificity for human hemoglobin enhances Staphylococcus aureus infection. Cell Host Microbe2010;8: 544–550.
- Hakansson A, Roche H, Mirza S, McDaniel LS, Brooks-Walter A, Briles DE.Characterization of binding of human lactoferrin to pneumococcal surface protein A. Infect Immun2001;69: 3372–3381.
- Musher DM. Streptococcus pneumoniae.In: Mandell GL, Bennett JE, Dolin RD (ed.) Principles and practice of infectious diseases.New York: Churchill Livingstone, 2000: 2128–2147.
- Weiser JN, Bae D, Fasching C, Scamurra RW, Ratner AJ, Janoff EN.Antibody-enhanced pneumococcal adherence requires IgA1 protease. Proc Natl Acad Sci USA2003;100: 4215–4220.
- Briles DE, Hollingshead SK, Swiatlo E et al.PspA and PspC: their potential for use as pneumococcal vaccines. Microb Drug Resist1997;3:401–408.
- Rosenow C, Ryan P, Weiser JN et al.Contribution of novel choline-binding proteins to adherence, colonization and immunogenicity of Streptococcus pneumoniae. Mol Microbiol1997;25: 819–829.
- Zhang JR, Mostov KE, Lamm ME et al.The polymeric immunoglobulin receptor translocates pneumococci across human nasopharyngeal epithelial cells. Cell2000;102: 827–837.
- Hammerschmidt S, Tillig MP, Wolff S, Vaerman JP, Chhatwal GS.Species-specific binding of human secretory component to SpsA protein of Streptococcus pneumoniae via a hexapeptide motif. Mol Microbiol2000;36: 726–736.
- Janulczyk R, Iannelli F, Sjoholm AG, Pozzi G, Bjorck L.Hic, a novel surface protein of Streptococcus pneumoniae that interferes with complement function. J Biol Chem2000;275: 37257–37263.
- Dave S, Brooks-Walter A, Pangburn MK, McDaniel LS.PspC, a pneumococcal surface protein, binds human factor H. Infect Immun2001;69: 3435–3437.
- Smith BL, Hostetter MK.C3 as substrate for adhesion of Streptococcus pneumoniae. J Infect Dis2000;182: 497–508.
- Voss S, Hallstrom T, Saleh M et al.The choline-binding protein PspC of Streptococcus pneumoniae interacts with the C-terminal heparin-binding domain of vitronectin. J Biol Chem2013;288: 15614–15627.
- Elm C, Braathen R, Bergmann S et al.Ectodomains 3 and 4 of human polymeric immunoglobulin receptor (hpIgR) mediate invasion of Streptococcus pneumoniae into the epithelium. J Biol Chem2004;279: 6296–6304.
- Lu L, Lamm ME, Li H, Corthesy B, Zhang JR.The human poly-Ig receptor binds to Streptococcus pneumoniae via domains 3 and 4. J Biol Chem2003;278: 48178–48187.
- Hammerschmidt S, Talay SR, Brandtzaeg P, Chhatwal GS.SpsA, a novel pneumococcal surface protein with specific binding to secretory immunoglobulin A and secretory component. Mol Microbiol1997;25: 1113–1124.
- Lu L, Ma Y, Zhang JR.Streptococcus pneumoniae recruits complement factor H through the amino terminus of CbpA. J Biol Chem2006;281: 15464–15474.
- Asmat TM, Klingbeil K, Jensch I, Burchhardt G, Hammerschmidt S.Heterologous expression of pneumococcal virulence factor PspC on the surface of Lactococcus lactis confers adhesive properties. Microbiology2012;158: 771–780.
- Asmat TM, Agarwal V, Rath S, Hildebrandt JP, Hammerschmidt S.Streptococcus pneumoniae infection of host epithelial cells via polymeric immunoglobulin receptor transiently induces calcium release from intracellular stores. J Biol Chem2011;286: 17861–17869.
- Agarwal V, Asmat TM, Dierdorf NI, Hauck CR, Hammerschmidt S.Polymeric immunoglobulin receptor-mediated invasion of Streptococcus pneumoniae into host cells requires a coordinate signaling of SRC family of protein-tyrosine kinases, ERK, and c-Jun N-terminal kinase. J Biol Chem2010;285: 35615–35623.
- Agarwal V, Asmat TM, Luo S, Jensch I, Zipfel PF, Hammerschmidt S.Complement regulator Factor H mediates a two-step uptake of Streptococcus pneumoniae by human cells. J Biol Chem2010;285: 23486–23495.
- Hammerschmidt S, Tillig MP, Wolff S, Vaerman JP, Chhatwal GS.Species-specific binding of human secretory component to SpsA protein of Streptococcus pneumoniae via a hexapeptide motif. Mol Microbiol2000;36: 726–736.
- Briles DE, Tart RC, Swiatlo E et al.Pneumococcal diversity: considerations for new vaccine strategies with emphasis on pneumococcal surface protein A (PspA). Clin Microbiol Rev1998;11: 645–657.
- Hammerschmidt S, Bethe G, Remane PH, Chhatwal GS.Identification of pneumococcal surface protein A as a lactoferrin-binding protein of Streptococcus pneumoniae. Infect Immun1999;67: 1683–1687.
- Tai SS, Lee CJ, Winter RE.Hemin utilization is related to virulence of Streptococcus pneumoniae. Infect Immun1993;61: 5401–5405.
- Shaper M, Hollingshead SK, Benjamin WH Jr, Briles DE.PspA protects Streptococcus pneumoniae from killing by apolactoferrin, and antibody to PspA enhances killing of pneumococci by apolactoferrin [corrected]. Infect Immun2004;72: 5031–5040.
- Mirza S, Wilson L, Benjamin WH Jr et al.Serine protease PrtA from Streptococcus pneumoniae plays a role in the killing of S. pneumoniae by apolactoferrin. Infect Immun2011;79: 2440–2450.
- Cossart P.Illuminating the landscape of host–pathogen interactions with the bacterium Listeria monocytogenes. Proc Natl Acad Sci USA2011;108: 19484–19491.
- Wollert T, Pasche B, Rochon M, Deppenmeier S, van den Heuvel J, Gruber AD, et al.Extending the host range of Listeria monocytogenes by rational protein design. Cell2007;129: 891–902.
- Bison AL, Stevens DL. Streptococcus pyogenes.In: Mandell GL, Bennett JE, Dolin RD (ed.) Principles and practice of infectious diseases.7th ed.New York: Elsevier Churchill Livingstone, 2010: 2593–2610.
- Sun H, Ringdahl U, Homeister JW, Fay WP et al.Plasminogen is a critical host pathogenicity factor for group A streptococcal infection. Science2004;305: 1283–1286.
- Boyle MD, Lottenberg R.Plasminogen activation by invasive human pathogens. Thromb Haemost1997;77: 1–10.
- Cunningham MW.Pathogenesis of group A streptococcal infections. Clin Microbiol Rev2000;13: 470–511.
- Fischetti VA.Streptococcal M protein: molecular design and biological behavior. Clin Microbiol Rev1989;2: 285–314.
- Darmstadt GL, Mentele L, Podbielski A, Rubens CE.Role of group A streptococcal virulence factors in adherence to keratinocytes. Infect Immun2000;68: 1215–1221.
- Rezcallah MS, Hodges K, Gill DB, Atkinson JP, Wang B, Cleary PP.Engagement of CD46 and alpha5beta1 integrin by group A streptococci is required for efficient invasion of epithelial cells. Cell Microbiol2005;7: 645–653.
- Thern A, Stenberg L, Dahlback B, Lindahl G.Ig-binding surface proteins of Streptococcus pyogenes also bind human C4b-binding protein (C4BP), a regulatory component of the complement system. J Immunol1995;154: 375–386.
- Kantor FS.Fibrinogen precipitation by streptococcal M protein. I. Identity of the reactants, and stoichiometry of the reaction. J Exp Med1965;121: 849–859.
- Whitnack E, Beachey EH.Antiopsonic activity of fibrinogen bound to M protein on the surface of group A streptococci. J Clin Invest1982;69: 1042–1045.
- Heath DG, Cleary PP.Fc-receptor and M-protein genes of group A streptococci are products of gene duplication. Proc Natl Acad Sci USA1989;86: 4741–4745.
- Berge A, Kihlberg BM, Sjoholm AG, Bjorck L.Streptococcal protein H forms soluble complement-activating complexes with IgG, but inhibits complement activation by IgG-coated targets. J Biol Chem1997;272: 20774–20781.
- Pizarro-Cerda J, Cossart P.Listeria monocytogenes membrane trafficking and lifestyle: the exception or the rule? Annu Rev Cell Dev Biol2009;25: 649–670.
- Stavru F, Archambaud C, Cossart P.Cell biology and immunology of Listeria monocytogenes infections: novel insights. Immunol Rev2011;240: 160–184.
- Schubert WD, Urbanke C, Ziehm T et al.Structure of internalin, a major invasion protein of Listeria monocytogenes, in complex with its human receptor E-cadherin. Cell2002;111: 825–836.
- Mengaud J, Ohayon H, Gounon P, Mege RM, Cossart P.E-cadherin is the receptor for internalin, a surface protein required for entry of L. monocytogenes into epithelial cells. Cell1996;84: 923–932.
- Lecuit M, Dramsi S, Gottardi C, Fedor-Chaiken M, Gumbiner B, Cossart P.A single amino acid in E-cadherin responsible for host specificity towards the human pathogen Listeria monocytogenes. EMBO J1999;18: 3956–3963.
- Lecuit M, Vandormael-Pournin S, Lefort J et al.A transgenic model for listeriosis: role of internalin in crossing the intestinal barrier. Science2001;292: 1722–1725.
- Dramsi S, Biswas I, Maguin E, Braun L, Mastroeni P, Cossart P.Entry of Listeria monocytogenes into hepatocytes requires expression of inIB, a surface protein of the internalin multigene family. Mol Microbiol1995;16: 251–261.
- Ireton K, Payrastre B, Chap H et al.A role for phosphoinositide 3-kinase in bacterial invasion. Science1996;274: 780–782.
- Braun L, Ohayon H, Cossart P.The InIB protein of Listeria monocytogenes is sufficient to promote entry into mammalian cells. Mol Microbiol1998;27: 1077–1087.
- Braun L, Ghebrehiwet B, Cossart P.gC1q-R/p32, a C1q-binding protein, is a receptor for the InlB invasion protein of Listeria monocytogenes. EMBO J2000;19: 1458–1466.
- Shen Y, Naujokas M, Park M, Ireton K.InIB-dependent internalization of Listeria is mediated by the Met receptor tyrosine kinase. Cell2000;103: 501–510.
- Jonquieres R, Pizarro-Cerda J, Cossart P.Synergy between the N- and C-terminal domains of InlB for efficient invasion of non-phagocytic cells by Listeria monocytogenes. Mol Microbiol2001;42: 955–965.
- Disson O, Nikitas G, Grayo S, Dussurget O, Cossart P, Lecuit M.Modeling human listeriosis in natural and genetically engineered animals. Nat Protoc2009;4:799–810.
- Torres VJ, Pishchany G, Humayun M, Schneewind O, Skaar EP.Staphylococcus aureus IsdB is a hemoglobin receptor required for heme iron utilization. J Bacteriol2006;188: 8421–8429.
- Foley SL, Nayak R, Hanning IB, Johnson TJ, Han J, Ricke SC.Population dynamics of Salmonella enterica serotypes in commercial egg and poultry production. Appl Environ Microbiol2011;77: 4273–4279.
- Goto Y, Kiyono H.Epithelial cell microRNAs in gut immunity. Nat Immunol2011;12: 195–197.
- Ron EZ.Host specificity of septicemic Escherichia coli: human and avian pathogens. Curr Opin Microbiol2006;9: 28–32.
- Babai R, Stern BE, Hacker J, Ron EZ.New fimbrial gene cluster of S-fimbrial adhesin family. Infect Immun2000;68: 5901–5907.
- Yerushalmi Z, Smorodinsky NI, Naveh MW, Ron EZ.Adherence pili of avian strains of Escherichia coli O78. Infect Immun1990;58: 1129–1131.
- Stromberg N, Marklund BI, Lund B et al.Host-specificity of uropathogenic Escherichia coli depends on differences in binding specificity to Gal alpha 1-4Gal-containing isoreceptors. EMBO J1990;9: 2001–2010.
- Noinaj N, Buchanan SK, Cornelissen CN.The transferrin-iron import system from pathogenic Neisseria species. Mol Microbiol2012;86: 246–257.
- Brehm MA, Jouvet N, Greiner DL, Shultz LD.Humanized mice for the study of infectious diseases. Curr Opin Immunol2013;25: 428–435.
- Adrian PV, Bogaert D, Oprins M et al.Development of antibodies against pneumococcal proteins alpha-enolase, immunoglobulin A1 protease, streptococcal lipoprotein rotamase A, and putative proteinase maturation protein A in relation to pneumococcal carriage and Otitis Media. Vaccine2004;22: 2737–2742.
- Paton JC, Boslego JW.Protein vaccines.In: Siber GR, Klugman KP, Makela PH (ed.) Pneumococcal vaccines—the impact of conjugate vaccine.6th ed.Washington, DC: ASM Press, 2008: 421–435.