Abstract
Zika virus (ZIKV) is a re-emerging mosquito-borne flavivirus that has recently caused extensive outbreaks in Central and South America and the Caribbean. Given its association with Guillain–Barré syndrome in adults and neurological and ocular malformities in neonates, ZIKV has become a pathogen of significant public health concern worldwide. ZIKV shares a considerable degree of genetic identity and structural homology with other flaviviruses, including dengue virus (DENV). In particular, the surface glycoprotein envelope (E), which is involved in viral fusion and entry and is therefore a chief target for neutralizing antibody responses, contains regions that are highly conserved between the two viruses. This results in immunological cross-reactivity, which in the context of prior DENV exposure, may have significant implications for the generation of immune responses to ZIKV and affect disease outcomes. Here we address the issue of humoral cross-reactivity between DENV and ZIKV, reviewing the evidence for and discussing the potential impact of this cross-recognition on the functional quality of antibody responses against ZIKV. These considerations are both timely and relevant to future vaccine design efforts, in view of the existing overlap in the distribution of ZIKV and DENV and the likely spread of ZIKV to additional DENV-naive and experienced populations.
Emerging Microbes & Infections (2017) 6, e33; doi:10.1038/emi.2017.42; published online 10 May 2017
Zika Virus Emergence and Changing Clinical Features
Zika virus (ZIKV) is a mosquito-borne flavivirus that was first discovered in 1947 in the Zika forest in Uganda.Citation1 The virus was isolated from a febrile rhesus macaque through a yellow fever surveillance network in the area. A year later, ZIKV was also isolated from Aedes mosquitoes from the same forest, pointing to a potential sylvatic transmission cycle involving non-human primates and mosquitoes.Citation1, Citation2 The first confirmed human ZIKV case was a laboratory-acquired infection reported in Uganda in 1964Citation3 following which sporadic cases of natural human infection were identified in NigeriaCitation4, Citation5 and Indonesia.Citation6 However, serological data suggests a wider geographical distribution, as seroprevalence for ZIKV antibodies has been documented in several additional countries spanning South and Southeast Asia and Africa,Citation7, Citation8 as well as in Uganda as early as 1952.Citation2
The first significant human outbreak of ZIKV outside these areas occurred on the Yap Island of Micronesia in 2007, which was marked by 49 confirmed infections, 131 probable or suspected cases and an estimated ZIKV infection rate of 73%.Citation9, Citation10 Thereafter in 2013, the virus caused a larger epidemic in French Polynesia, with estimates ranging between 8500 and 19 000 suspected infections.Citation11, Citation12 Until 2013, symptomatic ZIKV infections were primarily associated with mild illness involving fever, rash, myalgia, arthralgia and conjunctivitis.Citation7, Citation9 However, during the French Polynesia outbreak, many ZIKV patients presented with severe clinical manifestations including Guillain–Barré syndrome, which required hospitalization and medical interventions.Citation13, Citation14
In 2015, ZIKV was discovered to have spread to Brazil,Citation15, Citation16, Citation17 which initiated the largest ZIKV epidemic known to date. Since its emergence in Brazil, cases of autochthonous ZIKV transmission have been reported in nearly 50 additional countries and territories in the western hemisphere,Citation18 including the United States.Citation19 In addition, ZIKV infections in the Brazilian outbreak have been linked to complications in pregnancy, and severe ocular and neurological deformities in neonates born to ZIKV-infected mothers including microcephaly.Citation20, Citation21, Citation22 Besides the striking increase in the incidence of microcephaly reported concurrently with the ZIKV outbreak,Citation23 the presence of ZIKV in brain tissues of aborted microcephalic fetuses,Citation24, Citation25 as well as in the amniotic fluid of pregnant mothers of microcephalic fetusesCitation26 demonstrate a causal relationship between ZIKV infection and this devastating developmental defect.Citation27
Accordingly, ZIKV has now emerged as one of the most critical arboviruses and is a significant public health concern worldwide. Given the overlapping presence of DENV in a majority of ZIKV epidemic regions,Citation18, Citation28, Citation29 there is a pressing need to better understand the extent and characteristics of DENV–ZIKV immunological cross-reactivity. Further, the potential impact of this cross-reactivity on the protective efficacy of ZIKV-induced antibody responses warrants careful investigation.
Genetic and Immunological Relatedness Between Zikv and Denv
Structural similarities and sequence conservation
ZIKV is a member of the Flaviviridae virus family. Its positive sense, single-stranded RNA genome is contained within a nucleocapsid core that is surrounded by an outer envelope made up of two structural proteins: envelope (E) and pre-membrane (prM). The cryo-EM structure of ZIKV reveals that the virus has a nearly identical organizational structure to DENV, including the characteristic herringbone arrangement of E protein head-to-tail homodimers on the virus surfaceCitation30, Citation31 (). In addition to structural similarities between the viral particles, the main targets for antibody responses in dengue infections, namely E, prM and the non-structural protein NS1, share substantial amino-acid sequence identity between ZIKV and DENV.Citation30, Citation32, Citation33, Citation34 The considerable structural and genetic relatedness between ZIKV and DENV () has been hypothesized to cause immunological cross-reactivity between these closely related viruses, which may make diagnosing patients challenging as well as potentially impact protective/pathologic immune responses to these infections.
Figure 2 ZIKV and DENV E proteins share considerable sequence identity. (A) Phylogenetic tree, showing relatedness based on E protein sequence, created using MEGA7.Citation67 The evolutionary history between the viruses was inferred by using the maximum likelihood method based on the JTT matrix-based model.Citation68 The percentage of trees in which the associated viral sequences clustered together is shown next to the branches. Branches are drawn to scale, with lengths measured in the number of substitutions per site. (B) Heat map showing E protein sequence identity, generated with ggplot2 in R.Citation69 Sequences were aligned in Geneious version 6.1.Citation70 For (A) and (B), the ZIKV strains analyzed and their GenBank accession numbers are: PRVABC59: KU501215, MR766: AY632535, H/PF/2013: KJ776791, Yap/2007: EU545988 and SPH2015: KU321639. The DENV strains are DENV1 WestPac: U88535, DENV2 Tonga/72: AY744147.1, DENV3 Sleman/78: AY648961.1 and DENV4 Dominica/814669/1981: AF326573.1. In addition, the YFV strain Asibi: KF769016.1 was also included as an outgroup in the sequence analyses above.
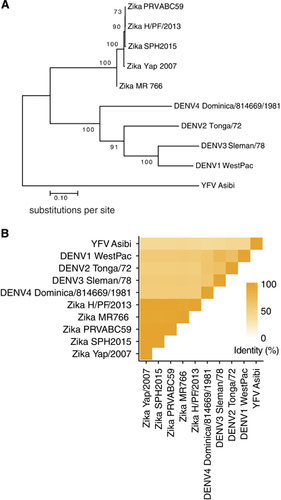
The E protein is involved in receptor binding, fusion and viral entry, and is a major target for neutralizing antibody responses in flaviviral infections. The crystal structure of ZIKV E shows that like other flaviviral E proteins, it contains three E protein domains (EDs): a central β-barrel-shaped domain I (EDI), a finger-like domain II (EDII) and a C-terminal immunoglobulin-like domain III (EDIII). The viral fusion peptide is located at the tip of EDII, and lies shielded by EDI and EDIII from the other monomeric subunit within the E protein dimerCitation35 (). In the context of DENV infection, EDIII was shown to be an important target for type-specific potently neutralizing antibodies in mice.Citation36, Citation37 However, serum depletion experiments reveal that EDIII-specific antibodies make up a small proportion of human DENV-immune serum.Citation38, Citation39 In contrast, the fusion loop region, containing the fusion loop epitope (FLE), appears to be more immunodominant and is a target for less potent but highly cross-reactive antibodies.Citation40 Another target of significant interest is termed the envelope dimer epitope (EDE).Citation40 EDE antibodies, directed toward epitopes that span across the E dimer interface, have been shown to be conformationally sensitive and broadly neutralizing. These dimer-dependent epitopes are part of a novel and growing class of complex, quaternary epitopes that are only present in the intact virion and not the monomeric form of E.Citation40, Citation41, Citation42, Citation43, Citation44 Recently, mAbs generated from memory B cells (MBCs) of ZIKV-infected patients were shown to target virus-specific EDIII as well as the highly conserved fusion loop, among other sites.Citation33, Citation45, Citation46 In addition, EDE-specific mAbs derived from DENV-infected patient MBCs potently cross-neutralized ZIKV.Citation47, Citation48 These findings demonstrate the relevance of FLE, EDE and EDIII epitopes in ZIKV antibody responses. However, additional studies are required to determine the contribution of such antibodies to the overall protective capacity of ZIKV-induced humoral immunity.
Figure 1 The ZIKV particle and E protein dimer. Cryo-EM surface structures of (A) immature (PDB 5U4W)Citation31 and (B) mature (PDB 5IRE)Citation30 ZIKV. The E protein dimer is highlighted in a yellow box. (C) The ZIKV E protein dimer colored by its domain, EDI: red, EDII: yellow and EDIII: blue.Citation30 The fusion loop is circled in orange. All structural figures in (A–C) were created using PyMol (Schrödinger LLC).
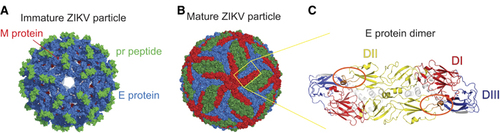
Evidence of immunological cross-reactivity
During the Yap State outbreak, suspected ZIKV cases were tested for serum binding and neutralizing (PRNT90) titers to ZIKV and other flaviviruses including DENV.Citation9, Citation10 Most patients tested were categorized as flavivirus pre-immune due to the presence of cross-reactive IgG in their acute-phase (<10 days after symptom onset) sera. A majority of these presumed secondary flavivirus cases showed measurable DENV PRNT90 serum titers.Citation10 Although ZIKV was the only detectable circulating virus during the outbreak (as stated in Lanciotti et al.,Citation10), DENV infections had been previously reported on the island.Citation49 Similarly, during the French Polynesia outbreak, cross-reactive serum titers against DENV were observed in ZIKV-probable cases.Citation14 This may have been in part due to the co-circulation of DENV1 and DENV3 in French Polynesia at the time of its ZIKV outbreak.Citation12 In addition, the country has experienced several dengue epidemics,Citation50, Citation51, Citation52 and moreover, serological surveys in 2011–2013 indicated that nearly 80% of the adult population was DENV seropositive.Citation53 Consequently, given the possibility of prior/concomitant exposure to DENV, the presence of cross-reactive antibody titers in patients from the Oceania outbreaks was not entirely unexpected.
More recently, several studies have addressed the issue of DENV–ZIKV immunological cross-reactivity by testing sera from ZIKV-infected individuals against DENV, or dengue sera against ZIKV. In one such study, sera from both DENV-naive and DENV pre-immune ZIKV patients strongly bound to ZIKV as well as DENV, with cross-reactive antibodies targeting both E and NS1 proteins.Citation33 The four patient samples in this study were from primary ZIKV cases, where infection occurred during travel to ZIKV-afflicted areas. Similarly, studies examining secondary dengue sera from endemic regions have shown cross-reactivity to ZIKV, in both binding and neutralization of the virus.Citation32, Citation47, Citation48
The studies described above illustrate ample cross-reactivity between ZIKV sera and DENV and vice versa. However, such analyses of polyclonal sera alone may not reveal the origin of cross-reactive antibody responses, or the relative proportion of type-specific versus cross-reactive antibodies. Especially in flavivirus-experienced populations, the possibility of multiple independent pools of antibodies contributing to the apparent serum cross-reactivity, rather than one common pool that recognizes both DENV and ZIKV also cannot be easily ruled out. This issue has been addressed by functional studies of mAbs generated from dengue patient plasmablasts. Our group and others have demonstrated that mAbs generated from in vivo activated, single-cell-sorted plasmablasts isolated during DENV infection can bind and neutralize ZIKV.Citation32, Citation48 As the source of the mAbs analyzed was plasmablasts specifically activated in response to DENV infection, these studies conclusively show that dengue-induced antibodies can cross-react to a heterologous virus, ZIKV. Additionally, mAb panels generated from MBCs of DENV-naive primary ZIKV patients have also been tested against DENV antigens to demonstrate ZIKV–DENV dual-reactivity at the single-cell level.Citation33
Impact of Cross-Reactive Humoral Immunity
Key targets for cross-reactive human antibody responses
The surface glycoproteins E and prM, and the non-structural protein NS1 have been identified as the main antigenic targets for human B-cell responses in DENV infections.Citation54, Citation55, Citation56, Citation57, Citation58 Whereas studies examining convalescent patients have shown the abundance of prM and NS1-specific MBCs,Citation54, Citation56, Citation57, Citation58 focused analyses of dengue plasmablast responses demonstrate that acute-phase antibodies are largely directed to the E protein.Citation40, Citation59, Citation60 Dengue-induced B-cell responses are dominated by antibodies that are cross-reactive to multiple serotypes, with a minor proportion exhibiting serotype-specific activity.Citation54, Citation56, Citation57, Citation58, Citation60 Recently, panels of mAbs from ZIKV-infected patients have been characterized to study the functional properties of ZIKV antibodies.Citation33 Antibodies generated from the MBCs of primary ZIKV patients included both ZIKV-specific as well as DENV cross-reactive mAbs. The subset of NS1 mAbs was largely ZIKV-specific despite the high cross-reactivity displayed by sera from the same donors. In addition, mAbs that bound EDIII, or whole virus but not recombinant E protein, were highly ZIKV-specific and potently neutralizing in vitro. In contrast, mAbs that were presumably EDI/EDII-specific, evident by their lack of binding to EDIII but recognition of the complete E protein, displayed cross-reactive binding to DENV but poorly neutralized ZIKV.Citation33
Dengue patient plasmablast and MBC-derived mAbs have also been tested for cross-reactive binding and neutralization to ZIKV.Citation32, Citation33, Citation47, Citation48, Citation61 Although binding to the virus was more broadly reported, the potent cross-neutralization of ZIKV by dengue-induced mAbs appears to be a relatively restricted phenotype.Citation32, Citation48, Citation61 Antibodies directed to the highly conserved and immunodominant FLE poorly neutralized ZIKV in vitro.Citation61 In contrast, the dimer-dependent EDE-specific mAbs were found to neutralize ZIKV potently.Citation47, Citation48, Citation61 The recognition and potent neutralization of ZIKV by EDE antibodies suggests that quaternary and other complex epitopes may be important antibody targets in the ZIKV immune response. Mapping the epitopes of additional ZIKV-neutralizing mAbs may reveal novel antigenic sites critical for protection and also inform future vaccine development efforts.
Antibody-dependent enhancement
Alongside their protective potential, antibody responses in dengue and other flaviviral infections have also been implicated in exacerbating disease. Studies have shown that infecting Fcγ-receptor (FcγR)—expressing cells in the presence of antibodies from flavivirus-immune donors—can significantly increase the rate of infection.Citation62 This phenomenon, termed antibody-dependent enhancement (ADE), is said to occur when cross-reactive antibodies present at sub-neutralizing concentrations facilitate the uptake of virions by permissive cells, thereby enhancing infection. Rather than inhibiting viral infection, the immune complexes formed between such antibodies and viral particles attach to cells and are internalized more efficiently via FcγR engagement.Citation62 In case of DENV infections, ADE is one of the several hypotheses proposed to explain the increase in disease severity associated with repeat heterotypic infections.Citation63, Citation64
ADE of DENV infection has been demonstrated by multiple groups using sera and mAbs from primary and secondary dengue patients. Both neutralizing and non-neutralizing mAbs have been shown to greatly enhance DENV infection in vitro.Citation54, Citation56, Citation58, Citation60 More recently, a few studies have also demonstrated that the ADE capacity of dengue-induced antibodies can also extend to ZIKV.Citation32, Citation48 These studies are important from an epidemiological perspective, as the vast majority of regions that have reported ZIKV cases also experience DENV outbreaks. While timely, the findings of these studies are not entirely surprising, given the significant biological similarities and abundant epitopes shared between the two viruses. In one of these studies, EDE mAbs, shown to potently neutralize all four DENV serotypesCitation40 as well as ZIKV,Citation48, Citation61 also enhanced ZIKV infection in vitro by ADE. However, incubating neutralizing concentrations of specific EDE mAbs with enhancing concentrations of polyclonal dengue sera reduced infection of FcγR-bearing cells. In contrast, the presence of poorly neutralizing FLE mAbs did not abrogate the enhancement of infection by serum antibodies.Citation48 These data suggest that the neutralization potential of antibodies targeting certain epitopes, such as EDE, may impede the ADE effect of enhancing antibodies, emphasizing the possible advantages of epitope-based vaccine design.
Protective potential of cross-reactive antibodies
In addition to the in vitro analyses described above, the protective capacity of several murine and human ZIKV-reactive mAbs has also been assessed in vivo. The fusion loop-specific murine mAb 2A10G6 () was found to confer in vivo protection from ZIKV infection, albeit at a suboptimal dose of 500 μg.Citation35 In another study, the EDIII lateral ridge-specific murine mAbs ZV-54 and ZV-67 protected mice from lethal challenge.Citation65 Unlike 2A10G6, the EDIII mAbs were ZIKV-specific and did not bind to DENV in vitro. Such ZIKV-specific mAbs may possess a selective advantage over broadly reactive mAb in their inability to induce ADE of DENV infection.
The human MBC-derived mAb ZIKV-117 was evaluated for its prophylactic and therapeutic efficacy in a pregnant and non-pregnant mouse model. In addition to reducing mortality in wild-type adult mice, in the fetal transmission model, administering ZIKV-117 decreased placental injury, reduced ZIKV infection of placenta and fetal tissue and improved fetal outcome overall. Epitope mapping of ZIKV-117 suggested that the mAb binds a quaternary epitope on the E protein dimer–dimer interface.Citation46 Another group generated a panel of mAbs from a convalescent ZIKV patient and of the mAbs isolated, Z23 and Z3L1 demonstrated potent ZIKV-specific in vitro neutralization and protected mice from weight loss and mortality after ZIKV infection. Although Z23 was mapped by cryo-EM to bind to EDIII, Z3L1 appeared to make contact primarily with EDI residuesCitation45 (). Unlike the above mAbs, EDE-specific mAb C10 () was isolated from a DENV-experienced but presumably ZIKV-naive donor, and was also shown to protect mice from lethal challenge.Citation47 Although these results are immensely promising, additional studies are required to dissect the mechanism of neutralization of these various mAbs. Moreover, several of these aforementioned studies were performed in immunocompromised mice, and therefore a more physiologically relevant characterization of their prophylactic and/or therapeutic potential merits further investigation in macaque models.
Figure 3 Cross-reactive and ZIKV-specific mAbs binding their E protein epitopes. (A) Murine mAb 2A10G6 binding to the conserved fusion loop on a ZIKV E monomer.Citation35 (B) Human mAb Z3L1 binding to a ZIKV-specific EDI epitope.Citation45 (C) Human mAb C10 (ribbon structure) binding to a dimer-dependent epitope on E protein dimer.Citation71 For (A–C), heavy and light chains of mAbs are colored red and pink, respectively. All residues conserved between DENV2 and ZIKV are colored orange. All figures were created using PyMol (Schrödinger LLC).
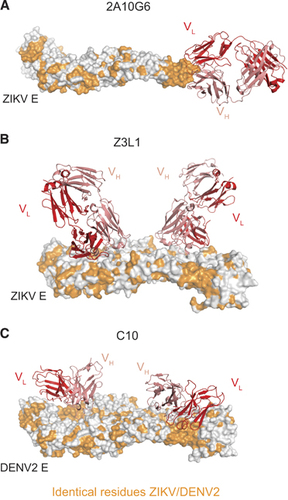
Sero-epidemiological data from historical ZIKV outbreaks as well as the findings of recent human serum-based studies may also provide added insight to the protective potential of immunological cross-reactivity against ZIKV infection. As demonstrated by in vitro studies, secondary dengue patient sera can strongly neutralize ZIKV while primary DENV sera exhibit limited cross-neutralization activity.Citation32, Citation47 This could be explained by the differences in longevity of type-specific versus cross-reactive antibodies after DENV infection. Although type-specific responses after primary infection are believed to be long-lived, cross-protective immunity can wane months after infection. Secondary heterotypic DENV exposures, on the other hand, may boost cross-reactive antibody production by reactivating cross-reactive MBCs, potentially resulting in broader neutralization capacity. Additionally, while acute or early convalescent (≤100 days after symptom onset) secondary sera were shown to potently neutralize ZIKV, other studies showed that late convalescent sera exhibited poor-to-moderate ZIKV neutralization.Citation32, Citation47, Citation48 These data suggest that while recently DENV-exposed individuals may maintain protective antibody titers against ZIKV, DENV-seropositive individuals exposed to ZIKV years after their dengue exposure may not benefit from effective cross-protection. These findings are consistent with the health outcomes of past and current ZIKV outbreaks. French Polynesia and Brazil, both countries with high DENV seroprevalence, experienced significant ZIKV epidemics with adverse clinical presentations. Cases of birth abnormalities and severe disease have also been reported in numerous DENV-endemic areasCitation28, Citation29, Citation66 suggesting that prior DENV exposure may not protect against future ZIKV infections. Future studies comparing naive versus recall human responses may help clarify the role of pre-existing cross-reactive antibodies during ZIKV disease, shedding more light on the protective/pathological potential of DENV immunity in the context of ZIKV infection.
Acknowledgments
This work was funded in part by NIH/NIAID Grant U19AI057266 and 1U01AI115651 to RA and JW. WH is a Cancer Research Institute Irvington Fellow supported by the Cancer Research Institute. All three authors are affiliated with Emory University School of Medicine, Atlanta, GA 30322, USA.
References
- Dick GW, Kitchen SF, Haddow AJ.Zika virus. I. Isolations and serological specificity. Trans R Soc Trop Med Hyg 1952;46: 509–520.
- Dick GW.Zika virus. II. Pathogenicity and physical properties. Trans R Soc Trop Med Hyg 1952;46: 521–534.
- Simpson DI.Zika virus infection in man. Trans R Soc Trop Med Hyg 1964;58: 335–338.
- Moore DL, Causey OR, Carey DEet al.Arthropod-borne viral infections of man in Nigeria, 1964–1970. Ann Trop Med Parasitol 1975;69: 49–64.
- Fagbami AH.Zika virus infections in Nigeria: virological and seroepidemiological investigations in Oyo State. J Hyg (Lond) 1979;83: 213–219.
- Olson JG, Ksiazek TG, Suhandiman, Triwibowo.Zika virus, a cause of fever in Central Java, Indonesia. Trans R Soc Trop Med Hyg 1981;75: 389–393.
- Musso D, Gubler DJ.Zika virus. Clin Microbiol Rev 2016;29: 487–524.
- Wikan N, Smith DR.Zika virus: history of a newly emerging arbovirus. Lancet Infect Dis 2016;16: e119–e126.
- Duffy MR, Chen TH, Hancock WTet al.Zika virus outbreak on Yap Island, Federated States of Micronesia. N Engl J Med 2009;360: 2536–2543.
- Lanciotti RS, Kosoy OL, Laven JJet al.Genetic and serologic properties of Zika virus associated with an epidemic, Yap State, Micronesia, 2007. Emerg Infect Dis 2008;14: 1232–1239.
- Cao-Lormeau VM, Roche C, Teissier Aet al.Zika virus, French polynesia, South Pacific, 2013. Emerg Infect Dis 2014;20: 1085–1086.
- Roth A, Mercier A, Lepers Cet al.Concurrent outbreaks of dengue, chikungunya and Zika virus infections - an unprecedented epidemic wave of mosquito-borne viruses in the Pacific 2012–2014. Euro Surveill 2014;19: 20929.
- Oehler E, Watrin L, Larre Pet al.Zika virus infection complicated by Guillain-Barre syndrome—case report, French Polynesia, December 2013. Euro Surveill 2014;19: 20720.
- Cao-Lormeau VM, Blake A, Mons Set al.Guillain-Barre Syndrome outbreak associated with Zika virus infection in French Polynesia: a case-control study. Lancet 2016;387: 531–1539.
- Campos GS, Bandeira AC, Sardi SI.Zika virus outbreak, Bahia, Brazil. Emerg Infect Dis 2015;21: 1885–1886.
- Zanluca C, Melo VC, Mosimann AL, Santos GI, Santos CN, Luz K.First report of autochthonous transmission of Zika virus in Brazil. Mem Inst Oswaldo Cruz 2015;110: 569–572.
- Cardoso CW, Paploski IA, Kikuti Met al.Outbreak of exanthematous illness associated with Zika, chikungunya, and dengue viruses, Salvador, Brazil. Emerg Infect Dis 2015;21: 2274–2276.
- PAHO/WHO. Regional Zika epidemiological update (Americas) March 10 2017. Washington, DC: PAHO/WHO, 2016. Available at http://www.paho.org/hq/index.php?option=com_docman&task=doc_view&Itemid=270&gid=38610&lang=en. (accessed 21 March 2017).
- Likos A, Griffin I, Bingham AMet al.Local mosquito-borne transmission of Zika virus - Miami-Dade and Broward Counties, Florida.2016MMWR Morb Mortal Wkly Rep 2016;65: 1032–1038.
- de Paula Freitas B, de Oliveira Dias JR, Prazeres Jet al.Ocular findings in infants with microcephaly associated with presumed Zika virus congenital infection in Salvador, Brazil. JAMA Ophthalmol 2016;134: 529–535.
- Mlakar J, Korva M, Tul Net al.Zika virus associated with microcephaly. N Engl J Med 2016;374: 951–958.
- Ventura CV, Maia M, Bravo-Filho V, Gois AL, Belfort R Jr.Zika virus in Brazil and macular atrophy in a child with microcephaly. Lancet 2016;387: 228.
- Kleber de Oliveira W, Cortez-Escalante J, De Oliveira WTet al.Increase in reported prevalence of microcephaly in infants born to women living in areas with confirmed Zika virus transmission during the first trimester of pregnancy - Brazil, 2015. MMWR Morb Mortal Wkly Rep 2016;65: 242–247.
- Martines RB, Bhatnagar J, Keating MKet al.Notes from the field: evidence of Zika virus infection in brain and placental tissues from two congenitally infected newborns and two fetal losses—Brazil, 2015. MMWR Morb Mortal Wkly Rep 2016;65: 159–160.
- Driggers RW, Ho CY, Korhonen EMet al.Zika virus infection with prolonged maternal viremia and fetal brain abnormalities. N Engl J Med 2016;374: 2142–2151.
- Calvet G, Aguiar RS, Melo ASet al.Detection and sequencing of Zika virus from amniotic fluid of fetuses with microcephaly in Brazil: a case study. Lancet Infect Dis 2016;16: 653–660.
- Rasmussen SA, Jamieson DJ, Honein MA, Petersen LR.Zika virus and birth defects - reviewing the evidence for causality. N Engl J Med 2016;374: 1981–1987.
- Bhatt S, Gething PW, Brady OJet al.The global distribution and burden of dengue. Nature 2013;496: 504–507.
- Stanaway JD, Shepard DS, Undurraga EAet al.The global burden of dengue: an analysis from the Global Burden of Disease Study 2013. Lancet Infect Dis 2016;16: 712–723.
- Sirohi D, Chen Z, Sun Let al.The 3.8A resolution cryo-EM structure of Zika virus. Science 2016;352: 467–470.
- Prasad VM, Miller AS, Klose Tet al.Structure of the immature Zika virus at 9A resolution. Nat Struct Mol Biol 2017;24: 184–186.
- Priyamvada L, Quicke KM, Hudson WHet al.Human antibody responses after dengue virus infection are highly cross-reactive to Zika virus. Proc Natl Acad Sci USA 2016;113: 7852–7857.
- Stettler K, Beltramello M, Espinosa DAet al.Specificity, cross-reactivity, and function of antibodies elicited by Zika virus infection. Science 2016;353: 823–826.
- Ye Q, Liu ZY, Han JF, Jiang T, Li XF, Qin CF.Genomic characterization and phylogenetic analysis of Zika virus circulating in the Americas. Infect Genet Evol 2016;43: 43–49.
- Dai L, Song J, Lu Xet al.Structures of the Zika virus envelope protein and its complex with a flavivirus broadly protective antibody. Cell Host Microbe 2016;19: 696–704.
- Sukupolvi-Petty S, Austin SK, Purtha WEet al.Type- and subcomplex-specific neutralizing antibodies against domain III of dengue virus type 2 envelope protein recognize adjacent epitopes. J Virol 2007;81: 12816–12826.
- Shrestha B, Brien JD, Sukupolvi-Petty Set al.The development of therapeutic antibodies that neutralize homologous and heterologous genotypes of dengue virus type 1. PLoS Pathog 2010;6: e1000823.
- Wahala WM, Kraus AA, Haymore LB, Accavitti-Loper MA, de Silva MA.Dengue virus neutralization by human immune sera: role of envelope protein domain III-reactive antibody. Virology 2009;392: 103–113.
- Midgley CM, Bajwa-Joseph M, Vasanawathana Set al.An in-depth analysis of original antigenic sin in dengue virus infection. J Virol 2011;85: 410–421.
- Dejnirattisai W, Wongwiwat W, Supasa Set al.A new class of highly potent, broadly neutralizing antibodies isolated from viremic patients infected with dengue virus. Nat Immunol 2015;16: 170–177.
- de Alwis R, Smith SA, Olivarez NPet al.Identification of human neutralizing antibodies that bind to complex epitopes on dengue virions. Proc Natl Acad Sci USA 2012;109: 7439–7444.
- Teoh EP, Kukkaro P, Teo EWet al.The structural basis for serotype-specific neutralization of dengue virus by a human antibody. Sci Transl Med 2012;4: 139ra183.
- Fibriansah G, Tan JL, Smith SAet al.A highly potent human antibody neutralizes dengue virus serotype 3 by binding across three surface proteins. Nat Commun 2015;6: 6341.
- Fibriansah G, Ibarra KD, Ng TSet al.Dengue virus. Cryo-EM structure of an antibody that neutralizes dengue virus type 2 by locking E protein dimers. Science 2015;349: 88–91.
- Wang Q, Yang H, Liu Xet al.Molecular determinants of human neutralizing antibodies isolated from a patient infected with Zika virus. Sci Transl Med 2016;8: 369ra179.
- Sapparapu G, Fernandez E, Kose Net al.Neutralizing human antibodies prevent Zika virus replication and fetal disease in mice. Nature 2016;540: 443–447.
- Swanstrom JA, Plante JA, Plante KSet al.Dengue virus envelope dimer epitope monoclonal antibodies isolated from dengue patients are protective against Zika VIrus. MBio 2016;7: e01123–16.
- Dejnirattisai W, Supasa P, Wongwiwat Wet al.Dengue virus sero-cross-reactivity drives antibody-dependent enhancement of infection with zika virus. Nat Immunol 2016;17: 1102–1108.
- Durand MA, Bel M, Ruwey I, Marfel M, Yug L, Ngaden V.An outbreak of dengue fever in Yap State. Pac Health Dialog 2005;12: 99–102.
- Cao-Lormeau VM, Roche C, Aubry Met al.Recent emergence of dengue virus serotype 4 in French Polynesia results from multiple introductions from other South Pacific Islands. PLoS One 2011;6: e29555.
- Cao-Lormeau VM, Musso D.Emerging arboviruses in the Pacific. Lancet 2014;384: 1571–1572.
- Singh N, Kiedrzynski T, Lepers C, Benyon EK.Dengue in the Pacific—an update of the current situation. Pac Health Dialog 2005;12: 111–119.
- Aubry M, Finke J, Teissier Aet al.Seroprevalence of arboviruses among blood donors in French Polynesia, 2011-2013. Int J Infect Dis 2015;41: 11–12.
- Beltramello M, Williams KL, Simmons CPet al.The human immune response to Dengue virus is dominated by highly cross-reactive antibodies endowed with neutralizing and enhancing activity. Cell Host Microbe 2010;8: 271–283.
- Vaughan K, Greenbaum J, Blythe M, Peters B, Sette A.Meta-analysis of all immune epitope data in the Flavivirus genus: inventory of current immune epitope data status in the context of virus immunity and immunopathology. Viral Immunol 2010;23: 259–284.
- Smith SA, Zhou Y, Olivarez NP, Broadwater AH, de Silva AM, Crowe JE Jr.Persistence of circulating memory B cell clones with potential for dengue virus disease enhancement for decades following infection. J Virol 2012;86: 2665–2675.
- Friberg H, Jaiswal S, West K, O'Ketch M, Rothman AL, Mathew A.Analysis of human monoclonal antibodies generated by dengue virus-specific memory B cells. Viral Immunol 2012;25: 348–359.
- Dejnirattisai W, Jumnainsong A, Onsirisakul Net al.Cross-reacting antibodies enhance dengue virus infection in humans. Science 2010;328: 745–748.
- Xu M, Hadinoto V, Appanna Ret al.Plasmablasts generated during repeated dengue infection are virus glycoprotein-specific and bind to multiple virus serotypes. J Immunol 2012;189: 5877–5885.
- Priyamvada L, Cho A, Onlamoon Net al.B cell responses during secondary dengue infection are dominated by highly cross-reactive, memory-derived plasmablasts. J Virol 2016;90: 5574–5585.
- Barba-Spaeth G, Dejnirattisai W, Rouvinski Aet al.Structural basis of potent Zika-dengue virus antibody cross-neutralization. Nature 2016;536: 48–53.
- Halstead SB.Neutralization and antibody-dependent enhancement of dengue viruses. Adv Virus Res 2003;60: 421–467.
- Vaughn DW, Green S, Kalayanarooj Set al.Dengue viremia titer, antibody response pattern, and virus serotype correlate with disease severity. J Infect Dis 2000;181: 2–9.
- Kliks SC, Nisalak A, Brandt WE, Wahl L, Burke DS.Antibody-dependent enhancement of dengue virus growth in human monocytes as a risk factor for dengue hemorrhagic fever. Am J Trop Med Hyg 1989;40: 444–451.
- Zhao H, Fernandez E, Dowd KAet al.Structural basis of Zika virus-specific antibody protection. Cell 2016;166: 1016–1027.
- PAHO/WHO Zika cases and congenital syndrome associated with Zika virus reported by countries and terriroties in the Americas, 2015-2016 Cumulative cases. Washington, DC: PAHO/WHO, 2016. Available at http://www.paho.org/hq/index.php?option=com_docman&task=doc_view&Itemid=270&gid=37582&lang=en (accessed 21 March 2017).
- Kumar S, Stecher G, Tamura K.MEGA7: molecular evolutionary genetics analysis version 7.0 for bigger datasets. Mol Biol Evol 2016;33: 1870–1874.
- Jones DT, Taylor WR, Thornton JM.The rapid generation of mutation data matrices from protein sequences. Comput Appl Biosci 1992;8: 275–282.
- Wickham H. ggplot2: Elegant Graphics For Data Analysis.New York: Springer, 2009.
- Kearse M, Moir R, Wilson Aet al.Geneious basic: an integrated and extendable desktop software platform for the organization and analysis of sequence data. Bioinformatics 2012;28: 1647–1649.
- Rouvinski A, Guardado-Calvo P, Barba-Spaeth Get al.Recognition determinants of broadly neutralizing human antibodies against dengue viruses. Nature 2015;520: 109–113.