Abstract
This study investigated the triazole phenotype and genotypic of clinical Aspergillus fumigatus isolates from China. We determined the triazole susceptibility profiles of 159 A. fumigatus isolates collected between 2011 and 2015 from four different areas in China tested against 10 antifungal drugs using the Clinical Laboratory Standard Institute M38-A2 method. For the seven itraconazole-resistant A. fumigatus isolates identified in the study, the cyp51A gene, including its promoter region, was sequenced and the mutation patterns were characterized. The resistant isolates were genotyped by microsatellite typing to determine the genetic relatedness to isolates from China and other countries. The frequency of itraconazole resistance in A. fumigatus isolates in our study was 4.4% (7/159). Six of the seven triazole-resistant isolates were recovered from the east and southeast of China, and one from was recovered from the west of China. No resistant isolates were found in the north. Three triazole-resistant isolates exhibited the TR34/L98H mutation, two carried the TR34/L98H/S297T/F495I mutation and one harbored a G54V mutation in the cyp51A gene. Analysis of the microsatellite markers from seven non-wild-type isolates indicated the presence of five unique genotypes, which clustered into two major genetic groups. The cyp51A gene mutations TR34/L98H and TR34/L98H/S297T were the most frequently found mutations, and the G54V mutation was reported for the first time in China. The geographic origin of the triazole-resistant isolates appeared to concentrate in eastern and south-eastern areas, which suggests that routine antifungal susceptibility testing in these areas should be performed for all clinically relevant A. fumigatus isolates to guide antifungal therapy and for epidemiological purposes.
Emerging Microbes & Infections (2017) 6, e109; doi:10.1038/emi.2017.97; published online 6 December
Introduction
Invasive aspergillosis (IA) in immunocompromised patients results in substantial morbidity and mortality.Citation1, Citation2 More than 40 Aspergillus species have been reported as causal agents of IA, and Aspergillus fumigatus is the leading pathogen in humans in most regions of the world.Citation2, Citation3 Antifungal agents such as the triazoles (itraconazole, posaconazole, voriconazole), the polyenes (e.g., amphotericin B) and the echinocandins are commonly prescribed drugs for patients diagnosed with IA.Citation4, Citation5 Recently, the antifungal azole isavuconazole was licensed for primary therapy for IA.Citation6 The key to successful treatment of IA includes early and accurate diagnosis and appropriate antifungal therapy at an adequate dosage. However, rapid, accurate and sensitive diagnosis is often a challenge in clinical laboratories,Citation7 and antifungal therapy is further complicated by the emergence of triazole resistance in A. fumigatus.Citation8, Citation9 It has been suggested that triazole resistance among Aspergillus species is more common than currently recognized.Citation9 Recently, an expert panel recommended that initial treatment regimens for IA should take into account the local drug resistance frequency of A. fumigatus.Citation10 Although triazole resistance has been reported in Asia,Citation11, Citation12, Citation13, Citation14 only a few Chinese surveillance reports on the antifungal susceptibility of clinical A. fumigatus isolates are available. Most reports come from restricted geographic areas and consider only a modest number of isolates or relatively few antifungal agents.Citation14, Citation15, Citation16, Citation17, Citation18 Given the lack of comprehensive information on the triazole resistance of isolates causing aspergillosis in China, the objectives of this study were to investigate the following: (1) the susceptibility of clinical A. fumigatus isolates from different areas in China to 10 antifungal drugs; (2) the triazole phenotypes and the mutation patterns in the cyp51A gene of resistant isolates; and (3) the genotypic relationships among azole-resistant isolates using microsatellite typing.Citation19
MATERIALS AND METHODS
Isolates
A total of 159 clinical isolates, including 37 from eastern areas, 39 from the south-eastern areas, 61 from northern areas and 22 from western areas, were collected between 2011 and 2015 in various medical centers from different geographic areas of China. Ethical approval was obtained, and all patients involved understood and agreed to the usage of these clinical specimens in the present study. All isolates were identified to the species level by sequencing the partial β-tubulin gene (benA) as described previously.Citation16 The obtained sequences were compared with the NCBI nucleotide database and the internal sequence database of the Westerdijk Fungal Biodiversity Institute containing verified benA sequences of Aspergillus section Fumigati. The geographical origin, clinical data and GenBank accession numbers for the generated benA sequences are listed in Supplementary Table S1.
Antifungal susceptibility testing
All isolates were tested for antifungal susceptibility under conditions described in the Clinical Laboratory Standard Institute M38-A2 reference method.Citation20 The antifungals amphotericin B, caspofungin, itraconazole, posaconazole, terbinafine and voriconazole were obtained from Sigma-Aldrich (Basingstoke, UK), and anidulafungin, micafungin, isavuconazole and ravuconazole were obtained from Toronto Research Chemicals Inc. (Toronto, Canada). The tested concentrations ranged from 0.008 to 4 mg/L for the echinocandins (anidulafungin, caspofungin and micafungin) and from 0.031 to 16 mg/L for the other compounds. All isolates were cultured on potato dextrose agar at 35 °C for 3–5 days and subcultured at least twice to ensure viability and purity. Conidia were harvested using sterile saline with Tween 20, and the final inoculum concentration of the suspension was adjusted to 0.4–5 × 104 colony-forming units (CFU)/mL in RPMI 1640 buffered with morpholinepropanesulfonic acid. Plates were incubated for 48 hours at 35 °C.Citation20 Both minimum inhibitory concentrations (MIC) and minimum effective concentrations (MEC) were determined microscopically (Primo Star Zeiss, Jena, Germany) at × 40 magnification. Epidemiological cutoff values (ECVs) were used to classify triazole susceptibility and to detect non-wild-type isolates.Citation20, Citation21, Citation22 Isolates were considered wild type when the MIC was equal to or lower than the ECV and non-wild type when the MIC was higher than the ECV. Isolates with MIC values >2 mg/L for amphotericin B,Citation23 >1 mg/L for isavuconazole, itraconazole and voriconazole and MIC values >0.5 mg/L for posaconazole were considered non-wild type (potentially resistant or less susceptible isolates).Citation24 There are no ECVs currently available for the echinocandins, ravuconazole or terbinafine. Quality control was performed as recommended in Clinical Laboratory Standard Institute document M38-A2 using strains A. fumigatus ATCC MYA-3627 and C. parapsilosis ATCC 22019.Citation25 All experiments for each isolate were performed using three independent replicates on different days.
Sequencing of A. fumigatus cyp51A gene
Non-wild-type A. fumigatus isolates were selected for detection of cyp51A mutations. Genomic DNA was extracted, and the full sequences of the cyp51A gene with the promoter region were amplified and sequenced (the primers used are listed in Supplementary Table S2).Citation26 The sequences obtained were aligned with the sequence from a triazole-susceptible isolate (GenBank accession AF338659) using ClustalW.Citation27 After the removal of the non-coding intron region, the predicted cyp51A amino-acid sequence was screened for substitutions, particularly those linked to triazole resistance.
Microsatellite genotyping
Microsatellite typing was used to determine the genetic relationships among the triazole-resistant A. fumigatus isolates. Nine loci were amplified in three multiplex-PCR assays, and subsequent fragment analysis was performed using the methods described previously.Citation28 Data were analyzed using Bionumericsv7.5 (Applied Maths, Sint-Martens-Latem, Belgium), and the dendrogram was generated using the categorical similarity coefficient followed by UPGMA cluster analysis implemented in Bionumerics. Additional microsatellite data from 18 clinical A. fumigatus isolates from China and 14 isolates from other countries such as Australia, Netherlands, India, Japan and Germany were included to provide additional insight into the genetic relationships among the triazole-resistant isolates.Citation13, Citation14, Citation29, Citation30, Citation31, Citation32
Statistical analysis
The geometric means, MIC/MEC, modal MIC/MEC, MIC /MEC ranges and MIC90 (MIC/MEC at which 90% of the isolates tested were inhibited) were measured for all isolates. Kruskal–Wallis testing was performed to test for significant differences between the MIC/MEC for each drug among four geographical areas using SPSS package v 20.0 (IBM Corp., Armonk, NY, USA). The differences were considered statistically significant at a P-value≤0.05 (two-tailed).
RESULTS
The MIC/MEC ranges, modal MIC/MEC, distribution of MICs/MECs of the 10 antifungal agents and the percentage of triazole-resistant isolates among the 159 isolates of A. fumigatus are presented in Table . Anidulafungin and micafungin were the most active drugs against A. fumigatus in vitro as they had the lowest modal MICs/MECs (mg/L) (0.016 (n=61) and 0.031 (n=64), respectively), followed by posaconazole (0.125 (n=72)), caspofungin (0.25 (n=119)), ravuconazole (0.25 (n=112)), voriconazole (0.25 (n=103)), itraconazole (0.5 (n=93)), amphotericin B (1 (n=119)), isavuconazole (1 (n=88)) and terbinafine (2 (n=79)).
Table 1 MIC/MEC ranges, modal of MICs/MECs, distribution of MICs/MECs (mg/L) obtained by testing the susceptibility of 159 A. fumigatus isolates to 10 antifungal agents and the percentage of non-WT isolates for the 159 isolates of A. fumigatus
The MIC values of the triazoles (except voriconazole) varied significantly among the four geographic areas (). The activity of itraconazole against western isolates was the most potent, whereas eastern isolates were less susceptible. In contrast, for posaconazole and ravuconazole, most A. fumigatus isolates from the western area had higher GM MICs than isolates in the other three areas; for isavuconazole, isolates from the east and the southeast had higher MICs than isolates from the north and the west. However, all isolates of A. fumigatus were particularly susceptible to the three echinocandins, although isolates from the west had lower MECs compared with isolates from the other areas ().
Table 2 Comparisons of activities of eight antifungal drugs tested against A. fumigatus isolates in four geographic areas
Seven isolates with MIC values above the established ECV for isavuconazole, itraconazole, posaconazole and voriconazole were identified, and the corresponding mutations in the cyp51A gene region and their geographical origins are shown in .
Table 3 MICs/MECs of seven triazole-resistant A. fumigatus isolates and their corresponding mutation type in the cyp51A gene region and geographical origin
The triazole-resistance rates for clinical isolates of A. fumigatus in the four geographic areas were variable, with 10.8% in the east, 5.1% in the southeast, 4.5% in the west and 0% in the north.
Analysis of microsatellite markers of the seven itraconazole-resistant isolates indicated the presence of five unique genotypes that clustered into two major independent genetic groups (Figure ). The genetic profiles of isolates STJ0119, STJ0140 and XYZ10138 were unique and were different from other isolates in the tree. They were distantly related to many Chinese isolates reported in previous studies.Citation14, Citation16 Three isolates (STJ0049, STJ0107 and STJ0048) were identical in their microsatellite profiles, and they were also genetically identical to four clinical isolates from China from previous studies (Figure ). These seven isolates appeared to be highly clonal based on the microsatellites.
Figure 1 Genotypic analysis of triazole-resistant Aspergillus fumigatus clinical isolates, including seven triazole-resistant isolates in this study, and analyses published previously from China and other countries. The dendrogram is based on a categorical analysis of nine microsatellite markers in combination with the unweighted Pair Group Method with arithmetic mean clustering. The scale bar indicates the percentage identity. *Denotes the seven clinical Chinese isolates in this study.
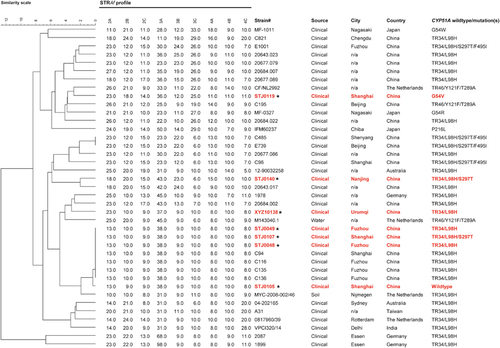
DISCUSSION
Our study showed that clinical A. fumigatus isolates from different areas in China have variable susceptibility profiles toward 10 common antifungal drugs, including two novel triazole antifungal agents: isavuconazole and ravuconazole. Despite the variability in drug susceptibilities, anidulafungin and micafungin were the most active/effective drugs (Table ), and triazoles were active against >95.6% (n=152/159) of the isolates, which is in agreement with other studies.Citation33, Citation34, Citation35 The novel triazoles isavuconazole and ravuconazole also had good in vitro activity against A. fumigatus (96.2% inhibition at MIC≤1 mg/L (n=153/159)). The in vitro activity of isavuconazole against A. fumigatus (modal MIC 1 mg/L) was similar to the activity of itraconazole (modal MIC 0.5 mg/L) but lower than either posaconazole (modal MIC 0.125 mg/L) or ravuconazole (modal MIC 0.25 mg/L) and voriconazole (modal MIC 0.25 mg/L), which was comparable to previous reports.Citation6, Citation33, Citation34, Citation35, Citation36 Nevertheless, ravuconazole showed excellent activity against A. fumigatus, as previously reported.Citation36, Citation37
In a 5-year period, the rate of triazole resistance in A. fumigatus isolates in our study was 4.4% (n=7/159), and this percentage was similar to the current global prevalence of triazole resistance in Aspergillus (3–6%).Citation10
Five of the seven resistant isolates exhibited a TR34/L98H or TR34/L98H/S297T mutation in the cyp51A gene, confirming the presence of TR34/L98H mutations in China.Citation14, Citation15, Citation16 The TR34/L98H mutation has been associated with exposure to azole fungicides in the environment rather than triazole therapy in patients.Citation38 Strikingly, seven such isolates in China (three from current study) showed no genetic variability, albeit with two different mutation patterns, suggesting a possible single and recent origin for these resistant isolates.
Variability in resistance frequency was observed in our study: triazole-resistant A. fumigatus was concentrated in the east (four non-wild-type isolates) and southeast (two non-wild-type isolates). One triazole-resistant isolate was obtained from the western area (), thousands of kilometers distant from the east. A similar variation in triazole-resistance prevalence between centers was found in the Netherlands.Citation7 Differences in resistance frequencies between medical centers might reflect differences in environmental exposure to triazole-resistant A. fumigatus. Further studies are needed to identify local environmental niches as they are probably critical to decrease the exposure of patients to A. fumigatus harboring these resistance mutations. Azole resistance in A. fumigatus due to non-cyp51A mechanisms is also increasingly reported,Citation39 which including activation of efflux pumps, in particular the overexpression of adenosine triphosphate-binding cassette transporters, transporters of the major facilitator superfamily, transcription factors, and non-synonymous mutations. The mutation P88L in HapE, an important subunit of the CCAAT-binding transcription factor complex, was found to confer resistance in A. fumigatus.Citation40 The occurrence of genomic deletions and non-synonymous mutations in genes (afyap1 and aldA) other than cyp51A has been described in A. fumigatus as possibly leading to azole resistance.Citation41
A. fumigatus isolates harboring the mutation TR34/L98H are found globally, and in this study, they conferred high MICs to all five triazole drugs. The results were different with the TR34/L98H/S297T mutants, which had lower voriconazole MICs (). This discrepancy had previously been noted, and we suggested at that time that the extra S297T mutation might represent a compensatory mutation.Citation42 The strain with the G54 point mutation represents the first report from China. Recently, this mutation in cyp51A, known previously from Europe and India, was also reported in Argentina.Citation39, Citation43, Citation44 Two new azoles, ravuconazole and isavuconazole, which are not yet approved for clinical use in China, showed reduced in vitro activity against itraconazole-resistant A. fumigatus isolates. This result is probably due to azole cross-resistance: 85.7% (n=6/7) of the itraconazole-resistant isolates were also resistant to ravuconazole and isavuconazole, and 71.4% (n=5/7) were resistant to posaconazole (). The isolate (STJ0119) with the G54 mutation was only resistant to itraconazole (MIC>16 mg/L) but not to the other triazoles.Citation45 This isolate was obtained from a patient admitted to a hospital in Shanghai with azole preexposure in the period before isolation. Unfortunately, we have no detailed information regarding the use of azole drugs in this patient. However, the TR46/Y121F/T289A combination of mutations was not found in this study, although recently a clinical isolate was reported from Beijing, China.Citation15
The geographical variation in A. fumigatus azole-resistant isolates suggests a need to include local drug resistance rates to devise public health policies and local guidelines for treatment and management. Furthermore, in the east and the southeast, where resistant isolates are prevalent, routine antifungal susceptibility testing should be performed for all clinically relevant A. fumigatus isolates to guide antifungal therapy and for epidemiological purposes.
Supplementary Table S1
Download MS Word (24.1 KB)Supplementary Table S2
Download MS Word (27 KB)Acknowledgments
This study was supported by the National Natural Science Foundation of China (81471926), the National Key Basic Research Program of China (2013CB531601 and 2013CB531606) and the Shanghai Municipal Natural Science Foundation (16ZR1432400 and 14DZ2272900). This publication was prepared as a collaborative study between the Department of Dermatology, Tongji Hospital of Tongji University, Tongji University School of Medicine, Shanghai, China and the Shanghai Institute of Medical Mycology, Changzheng Hospital, Second Military Medical University, Shanghai, China.
Supplementary Information for this article can be found on the Emerging Microbes & Infections website (http://www.nature.com/emi)
References
- Kosmidis C, Denning DW.The clinical spectrum of pulmonary aspergillosis. Thorax 2015;70: 270–277.
- Patterson TF, Thompson GR 3rd, Denning DWet al.Practice guidelines for the diagnosis and management of aspergillosis: 2016 update by the Infectious Diseases Society of America. Clin Infect Dis 2016;63: e1–e60.
- Tashiro T, Izumikawa K, Tashiro Met al.Diagnostic significance of Aspergillus species isolated from respiratory samples in an adult pneumology ward. Med Mycol 2011;49: 581–587.
- Denning DW, Cadranel J, Beigelman-Aubry Cet al.Chronic pulmonary aspergillosis: rationale and clinical guidelines for diagnosis and management. Eur Respir J 2016;47: 45–68.
- Kohno S, Izumikawa K.Posaconazole for chronic pulmonary aspergillosis: the next strategy against the threat of azole-resistant Aspergillus infection. Clin Infect Dis 2010;51: 1392–1394.
- Maertens JA, Raad II, Marr KAet al.Isavuconazole versus voriconazole for primary treatment of invasive mould disease caused by Aspergillus and other filamentous fungi (SECURE): a phase 3, randomised-controlled, non-inferiority trial. Lancet 2016;387: 760–769.
- Meis JF, Chowdhary A, Rhodes JLet al.Clinical implications of globally emerging azole resistance in Aspergillus fumigatus. Philos Trans R Soc Lond B Biol Sci 2016;371 (pii): 20150460.
- Howard SJ, Cerar D, Anderson MJet al.Frequency and evolution of Azole resistance in Aspergillus fumigatus associated with treatment failure. Emerg Infect Dis 2009;15: 1068–1076.
- Verweij PE, Chowdhary A, Melchers WJet al.Azole resistance in Aspergillus fumigatus: can we retain the clinical use of mold-active antifungal azoles? Clin Infect Dis 2016;62: 362–368.
- Verweij PE, Ananda-Rajah M, Andes Det al.International expert opinion on the management of infection caused by azole-resistant Aspergillus fumigatus. Drug Resist Updat 2015;21-22: 30–40.
- Kikuchi K, Watanabe A, Ito Jet al.Antifungal susceptibility of Aspergillus fumigatus clinical isolates collected from various areas in Japan. J Infect Chemother 2014;20: 336–338.
- Chowdhary A, Kathuria S, Randhawa HSet al.Isolation of multiple-triazole-resistant Aspergillus fumigatus strains carrying the TR/L98H mutations in the cyp51A gene in India. J Antimicrob Chemother 2012;67: 362–366.
- Wu CJ, Wang HC, Lee JCet al.Azole-resistant Aspergillus fumigatus isolates carrying TR(3)(4)/L98H mutations in Taiwan. Mycoses 2015;58: 544–549.
- Lockhart SR, Frade JP, Etienne KAet al.Azole resistance in Aspergillus fumigatus isolates from the ARTEMIS global surveillance study is primarily due to the TR/L98H mutation in the cyp51A gene. Antimicrob Agents Chemother 2011;55: 4465–4468.
- Chen Y, Lu Z, Zhao Jet al.Epidemiology and molecular characterizations of azole resistance in clinical and environmental Aspergillus fumigatus isolates from China. Antimicrob Agents Chemother 2016;60: 5878–5884.
- Liu M, Zeng R, Zhang Let al.Multiple cyp51A-based mechanisms identified in azole-resistant isolates of Aspergillus fumigatus from China. Antimicrob Agents Chemother 2015;59: 4321–4325.
- Chen J, Li H, Li Ret al.Mutations in the cyp51A gene and susceptibility to itraconazole in Aspergillus fumigatus serially isolated from a patient with lung aspergilloma. J Antimicrob Chemother 2005;55: 31–37.
- Pfaller MA, Castanheira M, Messer SAet al.In vitro antifungal susceptibilities of isolates of Candida spp. and Aspergillus spp. from China to nine systemically active antifungal agents: data from the SENTRY antifungal surveillance program. 2010 through 2012. Mycoses 2015;58: 209–214.
- Ashu EE, Hagen F, Chowdhary Aet al.Global population genetic analysis of Aspergillus fumigatus. mSphere 2017;2: e00019-17.
- Espinel-Ingroff A, Diekema DJ, Fothergill Aet al.Wild-type MIC distributions and epidemiological cutoff values for the triazoles and six Aspergillus spp. for the CLSI broth microdilution method (M38-A2 document). J Clin Microbiol 2010;48: 3251–3257.
- Rodriguez-Tudela JL, Alcazar-Fuoli L, Mellado Eet al.Epidemiological cutoffs and cross-resistance to azole drugs in Aspergillus fumigatus. Antimicrob Agents Chemother 2008;52: 2468–2472.
- Pfaller M, Boyken L, Hollis Ret al.Use of epidemiological cutoff values to examine 9-year trends in susceptibility of Aspergillus species to the triazoles. J Clin Microbiol 2011;49: 586–590.
- Espinel-Ingroff A, Cuenca-Estrella M, Fothergill Aet al.Wild-type MIC distributions and epidemiological cutoff values for amphotericin B and Aspergillus spp. for the CLSI broth microdilution method (M38-A2 document). Antimicrob Agents Chemother 2011;55: 5150–5154.
- Espinel-Ingroff A, Chowdhary A, Gonzalez GMet al.Multicenter study of isavuconazole MIC distributions and epidemiological cutoff values for Aspergillus spp. for the CLSI M38-A2 broth microdilution method. Antimicrob Agents Chemother 2013;57: 3823–3828.
- Arikan S, Gur D, Akova M.Comparison of Etest, microdilution and colorimetric dilution with reference broth macrodilution method for antifungal susceptibility testing of clinically significant Candida species isolated from immunocompromised patients. Mycoses 1997;40: 291–296.
- Ozmerdiven GE, Ak S, Ener Bet al.First determination of azole resistance in Aspergillus fumigatus strains carrying the TR34/L98H mutations in Turkey. J Infect Chemother 2015;21: 581–586.
- Hall T.BioEdit: a user-friendly biological sequence alignment editor and analysis program for Windows 95/98/NT. Nucleic Acids Symp Ser 1999;41: 95–98.
- de Valk HA, Meis JF, Curfs IMet al.Use of a novel panel of nine short tandem repeats for exact and high-resolution fingerprinting of Aspergillus fumigatus isolates. J Clin Microbiol 2005;43: 4112–4120.
- Kidd SE, Goeman E, Meis JFet al.Multi-triazole-resistant Aspergillus fumigatus infections in Australia. Mycoses 2015;58: 350–355.
- Tashiro M, Izumikawa K, Minematsu Aet al.Antifungal susceptibilities of Aspergillus fumigatus clinical isolates obtained in Nagasaki, Japan. Antimicrob Agents Chemother 2012;56: 584–587.
- Steinmann J, Hamprecht A, Vehreschild MJet al.Emergence of azole-resistant invasive aspergillosis in HSCT recipients in Germany. J Antimicrob Chemother 2015;70: 1522–1526.
- Chowdhary A, Sharma C, Kathuria Set al.Prevalence and mechanism of triazole resistance in Aspergillus fumigatus in a referral chest hospital in Delhi, India and an update of the situation in Asia. Front Microbiol 2015;6: 428.
- Gregson L, Goodwin J, Johnson Aet al.In vitro susceptibility of Aspergillus fumigatus to isavuconazole: correlation with itraconazole, voriconazole, and posaconazole. Antimicrob Agents Chemother 2013;57: 5778–5780.
- Guinea J, Pelaez T, Recio Set al.In vitro antifungal activities of isavuconazole (BAL4815), voriconazole, and fluconazole against 1,007 isolates of zygomycete, Candida, Aspergillus, Fusarium, and Scedosporium species. Antimicrob Agents Chemother 2008;52: 1396–1400.
- Howard SJ, Lass-Florl C, Cuenca-Estrella Met al.Determination of isavuconazole susceptibility of Aspergillus and Candida species by the EUCAST method. Antimicrob Agents Chemother 2013;57: 5426–5431.
- Diekema DJ, Messer SA, Hollis RJet al.Activities of caspofungin, itraconazole, posaconazole, ravuconazole, voriconazole, and amphotericin B against 448 recent clinical isolates of filamentous fungi. J Clin Microbiol 2003;41: 3623–3626.
- Pfaller MA, Messer SA, Hollis RJet al.Antifungal activities of posaconazole, ravuconazole, and voriconazole compared to those of itraconazole and amphotericin B against 239 clinical isolates of Aspergillus spp. and other filamentous fungi: report from SENTRY Antimicrobial Surveillance Program, 2000. Antimicrob Agents Chemother 2002;46: 1032–1037.
- Verweij PE, Snelders E, Kema GHet al.Azole resistance in Aspergillus fumigatus: a side-effect of environmental fungicide use? Lancet Infect Dis 2009;9: 789–795.
- Chowdhary A, Sharma C, Meis JF.Azole-resistant aspergillosis: epidemiology, molecular mechanisms, and treatment. J Infect Dis 2017;216: S436–S444.
- Camps SM, Dutilh BE, Arendrup MCet al.Discovery of a HapE mutation that causes azole resistance in Aspergillus fumigatus through whole genome sequencing and sexual crossing. PLoS One 2012;7: e50034.
- Hagiwara D, Takahashi H, Watanabe Aet al.Whole-genome comparison of Aspergillus fumigatus strains serially isolated from patients with aspergillosis. J Clin Microbiol 2014;52: 4202–4209.
- Abdolrasouli A, Rhodes J, Beale MAet al.Genomic context of azole resistance mutations in Aspergillus fumigatus determined using whole-genome sequencing. MBio 2015;6: e00536.
- Leonardelli F, Theill L, Nardin MEet al.First itraconazole resistant Aspergillus fumigatus clinical isolate harbouring a G54E substitution in Cyp51Ap in South America. Rev Iberoam Micol 2017;34: 46–48.
- Sharma C, Hagen F, Moroti Ret al.Triazole-resistant Aspergillus fumigatus harbouring G54 mutation: is it de novo or environmentally acquired? J Glob Antimicrob Resist 2015;3: 69–74.
- Mosquera J, Sharp A, Moore CBet al.In vitro interaction of terbinafine with itraconazole, fluconazole, amphotericin B and 5-flucytosine against Aspergillus spp. J Antimicrob Chemother 2002;50: 189–194.