Abstract
Background
Non-invasive right ventricular (RV) myocardial work (RVMW) is the latest indicator used to assess RV function. Nevertheless, the physiological determinants of RVMW have not been studied, and reference ranges for normal RVMW indices have yet to be established.
Methods
A total of 263 healthy volunteers (median age: 34 years, males: 38%) were prospectively enrolled. RVMW indices were analysed by an RV pressure-strain loop (RVPSL) in specific software.
Results
The lowest values of the RVMW indices in males and females were 133 mmHg% and 206 mmHg% for RV global work index (RVGWI), 165 mmHg% and 241 mmHg% for RV global constructive work (RVGCW) and 78% and 83% for RV global work efficiency (RVGWE), respectively. The highest values for RV global wasted work (RVGWW) in males and females were 67 mmHg% and 69 mmHg%, respectively. RVGWI and RVGCW were significantly lower in males than those in females. RVGWW significantly increased with age in males and females. RVGWE significantly decreased with age in females. Multivariable analysis revealed that RVGWI, RVGCW and RVGWE increased as pulmonary artery systolic pressure (PASP) increased.
Conclusions
Normal reference values of non-invasive RVMW were obtained by echocardiography. Quantitative data on RVMW could be essential in clinical work and clinical experiments.
1. Background
For decades, the importance of the right ventricle in clinical work and research on cardiovascular disease was neglected compared to that of the left ventricle. However, with increasing evidence that right ventricular (RV) dysfunction is closely related to the treatment and prognosis of diseases (e.g. acute myocardial infarction, heart failure, pulmonary hypertension [PH], after cardiac surgery and congenital heart disease), the right ventricle has received increasing attention from clinical and scientific researchers [Citation1]. The most frequently used methods to analyse RV function are measuring the tricuspid annular plane systolic excursion (TAPSE), RV fractional area change (RV FAC) and myocardial tissue Doppler velocities (S’) [Citation2–4]. However, TAPSE, RV FAC and S’ do not consider RV afterload. Because afterload has a major effect on the thin-walled right ventricle, ignoring the influence of afterload will make the parameters incapable of being used to accurately evaluate RV function [Citation5]. Recently, RV longitudinal strain, as a superior method of RV function evaluation, is still an afterload-dependent parameter [Citation6,Citation7].
RV myocardial work (RVMW) is a novel indicator to quantitatively analyse RV function [Citation8]. RVMW indices are acquired by integrating the RV global longitudinal strain (RV GLS), pulmonary artery pressures and tricuspid and pulmonic valvular events by specific software. Thus, RVMW could be used to theoretically evaluate RV function more precisely than conventional two-dimensional parameters. This study aimed (1) to obtain normal reference values for the RVMW in a large cohort of a healthy population; (2) to evaluate the effects of age and sex on RVMW parameters and (3) to explore the relationships between the RVMW indices and other baseline parameters.
2. Methods
2.1. Study population
A group of 372 healthy Asian volunteers were prospectively recruited from April 2021 to July 2021. The inclusion criteria were as follows: age ≥18 years, normal results on physical examination, normal electrocardiogram, normal results on two-dimensional echocardiography (2DE) and no history of cardiovascular or respiratory disease. The Institutional Ethics Committee approved the protocol, and all subjects provided informed consent before undergoing examinations.
2.2. Echocardiographic acquisition
Transthoracic echocardiography was performed using a Vivid E95 scanner (GE Vingmed Ultrasound, Norway) following recommended protocols [Citation9,Citation10]. 2DE images were acquired by an M5Sc probe, and four-dimensional echocardiography (4DE) RV images were acquired by a 4Vc probe. Acquisitions of the tricuspid regurgitation (TR) jets by colour Doppler were obtained from the apical four-chamber views. All electrocardiogram-triggered echocardiography images were obtained in three to five consecutive cardiac cycles during breath holding. Datasets were stored digitally and analysed offline.
2.3. Echocardiographic measurements
Echocardiographic images were analysed using EchoPAC v204 (GE Vingmed Ultrasound). RV end-diastolic and end-systolic volumes and RV ejection fraction were obtained by using the software package (4D Auto RVQ). RVMW indices were analysed with a software package (AFI) designed for the quantitative analysis of the left ventricular (LV) myocardial work (LVMW) by speckle tracking echocardiography. The prognostic validation of LVMW was performed in several studies [Citation11–13].
Pulmonary artery systolic pressure (PASP) was computed as PASP = 4vTR2 + mean right atrial (RA) pressure [Citation14]. Mean RA pressure was estimated based on the diameter and collapsibility of the inferior vena cava [Citation3]. The mean RV-RA gradient was estimated by tracing the TR velocity-time integral () [Citation15]. Pulmonary artery mean pressure (PAMP) equals the mean RV-RA gradient plus the mean RA pressure. According to the theoretical formula, the pulmonary artery diastolic pressure (PADP) was calculated as PADP = 1.5 [PAMP - (PASP/3)] [Citation3].
Figure 1. (A) Tracing the tricuspid regurgitation velocity-time integral; (B) analysis of speckle tracking echocardiography-derived right ventricular global longitudinal strain; (C) evaluation of the event timings of the tricuspid valve and pulmonic valve in the parasternal short-axis views; (D) measurement of right ventricular myocardial work by incorporating pulmonary pressures, right ventricular strain and cardiac cycle timings.
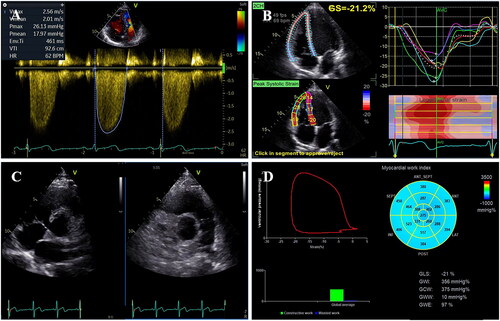
In the RV-focused apical four-chamber view, RV GLS was evaluated by tracing the interventricular septum and RV free wall [Citation10]. The event timings of the tricuspid and pulmonic valve were derived from direct visualisation in the parasternal short-axis views. Measurements of RV GLS, PASP and PADP were then synchronised by tricuspid and pulmonic valve event timings to noninvasively produce an RV pressure–strain loop (RVPSL) ().
RVMW indices acquired by RVPSL included the following four parameters:
RV global work index (RVGWI): the area of RVPSL, which represents the total RVMW during tricuspid valve closure and tricuspid valve opening.
RV global constructive work (RVGCW): positive work during shortening in systole and lengthening during isovolumic relaxation (IVR).
RV global wasted work (RVGWW): negative work during lengthening in systole and shortening during IVR.
RV global work efficiency (RVGWE): RVGCW/(RVGCW + RVGWW).
All the standard measurements were performed according to the widely accepted guidelines [Citation4].
2.4. Statistical analysis
The normality of variables was assessed with the Kolmogorov–Smirnov test. Normally distributed continuous variables are expressed as the mean ± SD, and those that were not normally distributed were expressed as the median (first quartile, third quartile). The highest (97.5th percentile) and lowest (2.5th percentile) expected values for RVMW indices were calculated from a bootstrap of 1000 samples.
Normally distributed data were compared using two-tailed independent Student’s t tests or one-way ANOVA. Nonnormally distributed data were compared using the Mann–Whitney U test or the Kruskal–Wallis test. The Spearman correlation coefficient was used to assess correlations between variables. Univariable and multivariable linear regression analyses were performed to test the correlations between the RVMW indices and the baseline parameters.
The intra- and interobserver variability of the RVMW indices were estimated in 20 random subjects by intraclass correlation coefficients and Bland–Altman analysis. One observer analysed the same echocardiographic images at two different times, and two independent blinded observers analysed the same echocardiographic images.
All data were analysed using SPSS 26.0 (SPSS Inc., IBM Corp). When the p value <.05, the difference between variables was considered statistically significant.
3. Results
3.1. Clinical characteristics
Seventeen subjects were excluded from enrolment due to poor 2DE images or poor RV 4DE images. Ninety-two subjects were excluded because the TR Doppler envelope could not be obtained or TR Doppler envelopes were of poor tracking quality. Thus, in our study population, the feasibility of the RVMW assessment was 70.7%. The clinical and echocardiographic characteristics of the enrolled subjects are displayed in .
Table 1. Clinical and echocardiographic characteristics of the study population.
3.2. RVMW parameters
summarises the RVMW parameters of the population. The lowest expected value for RVGWI was 133 mmHg% in males and 206 mmHg% in females, 165 mmHg% and 241 mmHg% for RVGCW and 78% and 83% for RVGWE, respectively. The highest expected values of the RVMW indices were 67 mmHg% in males and 69 mmHg% in females for RVGWW. RVGWI and RVGCW were significantly lower in males than in females, while RVGWW and RVGWE showed no significant differences between sexes. Correlations between indices of RVMW and classical parameters of RV systolic function are shown in Supplement Table 1.
Table 2. Parameters of right ventricular myocardial work.
3.3. Age and sex differences in RVMW parameters
and show the age and sex differences in the RVMW indices. RVGWW increased with age in males and females (R2 = 0.04, p = .034 and R2 = 0.11, p < .0001, respectively). RVGWE decreased with age in females (R2 = 0.04, p = .003) and as PASP increased (R2 = 0.06, p = .001). RVGWI and RVGCW were higher in females than those in males in the age <30 years, 30–40 years and 40–50 years subgroups (all p < .01). RVGCW was higher in males in the age ≥50 years subgroup than in those in the age <30 years, 30–40 years and 40–50 years subgroups (p = .005, p = .028 and p = .017, respectively). RVGWW was lower in females in the age <30 years group than in those in the age 40–50 years and ≥50 years subgroups (p < .0001 and p = .021, respectively).
Figure 2. Individual values of right ventricular global work index (RVGWI), right ventricular global constructive work (RVGCW), right ventricular global wasted work (RVGWW) and right ventricular global work efficiency (RVGWE) according to sex and age categories. Horizontal lines represent median values or mean values. *p Value <.05 between sexes.
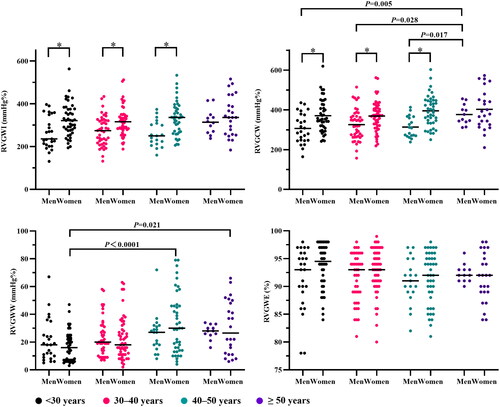
Table 3. Parameters of myocardial work and pulmonary artery pressure values according to sex and age.
3.4. RVMW indices and other baseline parameter relationships
Univariable analysis and multivariable analysis for the RVMW indices are shown in . As expected, RVGWE significantly decreased with age in the univariable analysis and the other RVMW indices increased with age. Multivariable analysis showed that (1) RVGWI and RVGCW were lower in males than in females (p < .0001 and p < .0001, respectively); (2) RVGWI, RVGCW and RVGWE increased with PASP (p < .0001, p < .0001 and p = .027, respectively) and (3) RVGWW increased with age, body mass index (BMI) and PADP (p = .001, p = .014 and p = .019, respectively).
Table 4. Univariable and multivariable analysis for RV myocardial work parameters.
3.5. Intra-observer and inter-observer variability
The intra- and inter-observer variability of the RVMW indices are summarised in , and , which indicate high intra- and interobserver reproducibility.
Figure 3. The Bland–Altman analysis for assessing intra-observer variability of right ventricular global work index, right ventricular global constructive work, right ventricular global wasted work and right ventricular global work efficiency.
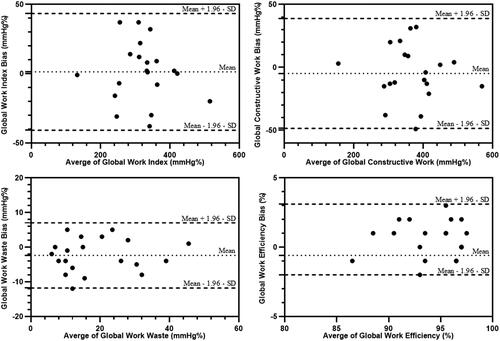
Figure 4. The Bland–Altman analysis for assessing inter-observer variability of right ventricular global work index, right ventricular global constructive work, right ventricular global wasted work and right ventricular global work efficiency.
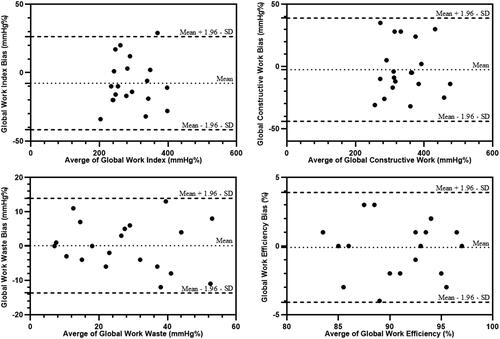
Table 5. Intra- and inter-observer variability of RV myocardial work indices.
4. Discussion
To our knowledge, this is the first study to report the use of echocardiography to quantitatively analyse non-invasive RVMW in a large cohort of a healthy population. Non-invasive RVMW, derived from RVPSL, was first introduced as the latest method to assess RV function by Butcher et al. [Citation8]. Recently, Russell et al. [Citation11] revealed that myocardial glucose metabolism, which was computed by 18 F-fluorodeoxyglucose (FDG) positron emission tomography (PET), was strongly correlated with non-invasive LVMW by an LV pressure-strain loop. Bokhari et al. [Citation16] used 18 F-FDG PET to demonstrate that RV myocardial glucose metabolism is associated with pulmonary pressure. Therefore, similar principles in non-invasive LVMW could be applied to non-invasive RVMW, allowing for the approximation of RVPSLs with RV force-segment length loops. Butcher et al. [Citation8] used the same method as in our study and demonstrated that the RVMW indices were substantially different between patients with a reduced LV ejection fraction and those in the healthy control group. Meanwhile, all RVMW indices were significantly correlated with RV GLS. Moreover, the standard echocardiographic parameters for the RV, including RV FAC, RV GLS, RV free wall longitudinal strain, TAPSE and echocardiographic stroke volume, were not significantly associated with invasive RV stroke volume obtained by right heart catheterisation. However, RVGCW was moderately correlated with invasive RV stroke volume. These discoveries reveal that afterload-enrolled non-invasive RVMW could be more reliable than conventional methods in evaluating RV function.
Our study establishes normal reference values for the RVMW indices in healthy Asian adults and examines the influence of baseline parameters on the reference ranges. Our data suggest that the RVMW index is independent of sex. In our study, RVGWI and RVGCW were lower in males than those in females, while no differences were found in RVGWW and RVGWE between sexes. Moreover, the multivariable analysis showed that RVGWI and RVGCW were lower in males than those in females. Specifically, when considering both age and sex, RVGWI and RVGCW were all higher in females than those in males in the subgroups of patients age <50 years. These findings may be due to RV myocardial systolic function being greater in females than that in males [Citation17]. Studies have pointed out that oestrogen in females has beneficial effects on the heart, such as regulating inflammation, myocardial hypertrophy, myocardial fibrosis and cardiovascular function [Citation18,Citation19]. After menopause, oestrogen in women is significantly reduced, and the protective effect on the heart is reduced.
Age has different effects on RVMW indices. Univariable analysis showed that RVGWI, RVGCW and RVGWW significantly increased and RVGWE significantly decreased with age. When sex was considered, only RVGWW increased with age in males and females. Multivariable analysis revealed that only RVGWW significantly increased with age. RVGWE decreased with age in females and PASP increased. RVGCW was higher in males in the age ≥50 years subgroup than that in the other age subgroups. RVGCW was lower in females in the age <30 years group than that in the age 40–50 years and age ≥50 years subgroups. Overall, the results above may reflect the physiological processes of ageing, such as progressive myocardial fibrosis and cardiomyocyte regulation [Citation20].
Finally, multivariable analysis showed that all the RVMW indices was not correlated with BMI, body surface area (BSA), or heart rate, except for RVGWW, which significantly increased with BMI. Multivariable analysis revealed that RVGWI, RVGCW and RVGWE increased with increasing PASP and RVGWW increased with increasing PADP. This result may be explained by the fact that increasing pulmonary artery pressure presents increasing RV afterload and may boost RVMW to higher levels of energy. Thus, in patients with PH, RVMW may have higher energy consumption levels to compensate for increased afterload, which is reflected in higher RVMW indices. Therefore, RVMW could play a vital role in assessing the effectiveness of treatment in patients with PH.
To date, LVMW has been studied in the areas of hypertension, heart failure, cardiac resynchronisation therapy, cardiac amyloidosis, nonobstructive hypertrophic cardiomyopathy, dilated cardiomyopathy, diabetes mellitus and so on [Citation21–25]. In contrast to LVMW, RVMW is rarely used in clinical and scientific research. As previous studies have demonstrated, TAPSE, RV FAC and S’ are the most commonly used measurements of RV function and are load-dependent [Citation4,Citation7]. However, some studies verified that RV GLS is less load-dependent [Citation7,Citation26,Citation27]. Because the RV has thin walls and low elastance, the afterload impact of RV GLS still cannot be ignored [Citation6]. Therefore, RVMW and the integration of RV GLS, pulmonary pressures and tricuspid and pulmonic valvular events could provide a more comprehensive and precise estimation of RV function. In the future, non-invasive analysis of RVMW by echocardiography can also be applied to assess RV function in a variety of diseases.
4.1. Clinical implications
As a non-invasive and convenient technique, the use of echocardiography for RVMW provides a scientific theoretical basis and practical significance for assessing RV function. However, to date, this method has only been used to assess RV function in patients with heart failure according to Butcher et al. [Citation8]. However, the data showed excellent agreement and repeatability in assessing RVMW indices, which increases the possibility that these afterload-enrolled RVMW indices could be used for risk stratification in other clinical practice and experimental studies, such as PH, RV dyssynchrony, cardiomyopathy, congenital heart disease, medical treatment and/or postoperative evaluation of cardiac disease. Moreover, the reference values of RVMW obtained from this study can be used as controls for the aforementioned diseases and efficacy evaluation.
4.2. Study limitations
Acquiring and quantifying RVMW datasets using a single provider platform may affect the applicability of these reference values to data obtained from other provider platforms. In addition, commercial software is required to measure RVMW and is specifically designed to measure LVMW. RV GLS is derived by tracing the RV free wall and interventricular septum because of the complex and irregular shape of the right ventricle [Citation10]. However, to our knowledge, there is still no ultrasound software that can use 3D RV GLS to generate RVMW. The RV afterload may have been inaccurate because it was estimated by calculating the pulmonary pressure rather than by performing direct measurements. Therefore, the calculation of RVMW is not as accurate as that of LVMW [Citation28,Citation29]. Non-invasive RVPSL may need to be validated by invasive RVPSL in the future. Meanwhile, for ethical reasons, right heart catheterisation was not used to verify the validity of RVMW. Additionally, approximately one in four healthy subjects were excluded because of small size and not holosystolic regurgitation, which may have caused selection bias. Furthermore, although all subjects were asymptomatic at the regular exams, we cannot rule out the possibility of subclinical cardiovascular or respiratory disease, particularly in elderly individuals. Finally, it remains unknown whether our results can be generalised to non-Asian subjects.
5. Conclusions
This is the first comprehensive study using echocardiography to obtain reference values for RVMW in a large cohort of a normal population. Age, sex and pulmonary artery pressure should be considered to identify RVMW indices. The availability of RVMW reference values could strengthen the value of echocardiography in evaluating RV function.
Supplemental Material
Download MS Word (17.5 KB)Disclosure statement
No potential conflict of interest was reported by the author(s).
References
- Konstam MA, Kiernan MS, Bernstein D, et al. Evaluation and management of right-sided heart failure: a scientific statement from the American Heart Association. Circulation. 2018;137(20):e578–e622.
- Aloia E, Cameli M, D'Ascenzi F, et al. TAPSE: an old but useful tool in different diseases. Int J Cardiol. 2016;225:177–183.
- Rudski LG, Lai WW, Afilalo J, et al. Guidelines for the echocardiographic assessment of the right heart in adults: a report from the American Society of Echocardiography endorsed by the European Association of Echocardiography, a registered branch of the European Society of Cardiology, and the Canadian Society of Echocardiography. J Am Soc Echocardiogr. 2010;23(7):685–713; quiz 786–688.
- Lang RM, Badano LP, Mor-Avi V, et al. Recommendations for cardiac chamber quantification by echocardiography in adults: an update from the American Society of Echocardiography and the European Association of Cardiovascular Imaging. J Am Soc Echocardiogr. 2015;28(1):1.e14–39.e14.
- Crystal GJ, Pagel PS. Right ventricular perfusion: physiology and clinical implications. Anesthesiology. 2018;128(1):202–218.
- Walker LA, Buttrick PM. The right ventricle: biologic insights and response to disease: updated. Curr Cardiol Rev. 2013;9(1):73–81.
- Tadic M, Pieske-Kraigher E, Cuspidi C, et al. Right ventricular strain in heart failure: clinical perspective. Arch Cardiovasc Dis. 2017;110(10):562–571.
- Butcher SC, Fortuni F, Montero-Cabezas JM, et al. Right ventricular myocardial work: proof-of-concept for non-invasive assessment of right ventricular function. Eur Heart J Cardiovasc Imaging. 2021;22(2):142–152.
- Cosyns B, Garbi M, Separovic J, et al. Education committee of the European Association of Cardiovascular Imaging A: update of the echocardiography core syllabus of the European Association of Cardiovascular Imaging (EACVI). Eur Heart J Cardiovasc Imaging. 2013;14(9):837–839.
- Badano LP, Kolias TJ, Muraru D, et al. Standardization of left atrial, right ventricular, and right atrial deformation imaging using two-dimensional speckle tracking echocardiography: a consensus document of the EACVI/ASE/industry task force to standardize deformation imaging. Eur Heart J Cardiovasc Imaging. 2018;19(6):591–600.
- Russell K, Eriksen M, Aaberge L, et al. A novel clinical method for quantification of regional left ventricular pressure-strain loop area: a non-invasive index of myocardial work. Eur Heart J. 2012;33(6):724–733.
- Edwards NFA, Scalia GM, Shiino K, et al. Global myocardial work is superior to global longitudinal strain to predict significant coronary artery disease in patients with normal left ventricular function and wall motion. J Am Soc Echocardiogr. 2019;32(8):947–957.
- Hubert A, Le Rolle V, Leclercq C, et al. Estimation of myocardial work from pressure-strain loops analysis: an experimental evaluation. Eur Heart J Cardiovasc Imaging. 2018;19(12):1372–1379.
- Vazquez de Prada JA, Ruano J, Martin-Duran R, et al. Noninvasive determination of pulmonary arterial systolic pressure by continuous wave doppler. Int J Cardiol. 1987;16(2):177–184.
- Aduen JF, Castello R, Lozano MM, et al. An alternative echocardiographic method to estimate mean pulmonary artery pressure: diagnostic and clinical implications. J Am Soc Echocardiogr. 2009;22(7):814–819.
- Bokhari S, Raina A, Rosenweig EB, et al. PET imaging may provide a novel biomarker and understanding of right ventricular dysfunction in patients with idiopathic pulmonary arterial hypertension. Circ Cardiovasc Imaging. 2011;4(6):641–647.
- Petersen SE, Aung N, Sanghvi MM, et al. Reference ranges for cardiac structure and function using cardiovascular magnetic resonance (CMR) in Caucasians from the UK biobank population cohort. J Cardiovasc Magn Reson. 2017;19(1):18.
- Deroo BJ, Korach KS. Estrogen receptors and human disease. J Clin Invest. 2006;116(3):561–570.
- Voloshenyuk TG, Gardner JD. Estrogen improves TIMP-MMP balance and collagen distribution in volume-overloaded hearts of ovariectomized females. Am J Physiol Regul Integr Comp Physiol. 2010;299(2):R683–R693.
- Karamitsos TD, Arvanitaki A, Karvounis H, et al. Myocardial tissue characterization and fibrosis by imaging. JACC Cardiovasc Imaging. 2020;13(5):1221–1234.
- Chan J, Edwards NFA, Khandheria BK, et al. A new approach to assess myocardial work by non-invasive left ventricular pressure-strain relations in hypertension and dilated cardiomyopathy. Eur Heart J Cardiovasc Imaging. 2019;20(1):31–39.
- Wang CL, Chan YH, Wu VC, et al. Incremental prognostic value of global myocardial work over ejection fraction and global longitudinal strain in patients with heart failure and reduced ejection fraction. Eur Heart J Cardiovasc Imaging. 2021;22(3):348–356.
- Vecera J, Penicka M, Eriksen M, et al. Wasted septal work in left ventricular dyssynchrony: a novel principle to predict response to cardiac resynchronization therapy. Eur Heart J Cardiovasc Imaging. 2016;17(6):624–632.
- Clemmensen TS, Eiskjær H, Ladefoged B, et al. Prognostic implications of left ventricular myocardial work indices in cardiac amyloidosis. Eur Heart J Cardiovasc Imaging. 2021;22(6):695–704. 22
- Huang D, Cui C, Zheng Q, et al. Quantitative analysis of myocardial work by non-invasive left ventricular pressure-strain loop in patients with type 2 diabetes mellitus. Front Cardiovasc Med. 2021;8:733339.
- Wright L, Negishi K, Dwyer N, et al. Afterload dependence of right ventricular myocardial strain. J Am Soc Echocardiogr. 2017;30(7):676.e671–684.e671.
- Missant C, Rex S, Claus P, et al. Load-sensitivity of regional tissue deformation in the right ventricle: isovolumic versus ejection-phase indices of contractility. Heart. 2008;94(4):e15.
- Redington AN, Gray HH, Hodson ME, et al. Characterisation of the normal right ventricular pressure-volume relation by biplane angiography and simultaneous micromanometer pressure measurements. Br Heart J. 1988;59(1):23–30.
- Sheehan F, Redington A. The right ventricle: anatomy, physiology and clinical imaging. Heart. 2008;94(11):1510–1515.