Abstract
In order to analyze X-ray markers for potential use in biodegradable implants or radiostereogrammatic analysis (RSA), we combined iopromide contrast fluid with biodegradable calcium phosphate cement. The radio-opacity of 10×10 mm markers containing different iodine concentrations (0, 120, 240, 360 and 720 mg per gram cement) was compared to an aluminium wedge of increasing (1–10 mm) thickness. The addition of iopromide increased the radio-opacity in a dose-dependent manner, which was comparable to 9-mm aluminium at concentrations of 240–720 mg/g. Radiographs of markers placed in explanted rabbit and in human femora were made to investigate the clinical accuracy for position determination. Markers of 1×1 mm (120 mg/g) were clearly discernable in all femora, and could be used to adequately measure distances of 5–45 mm (accuracy 0.10–2.19 mm). These markers might be embedded in biodegradable implants or used as temporary markers in the bone to analyze postoperative position on radiographs.
New biodegradable devices offer a valuable alternative to metal implants in a wide range of orthopedic procedures (van Dijk et al. Citation2002a). Biodegradable implants can be used in minimally invasive surgery, they do not require removal of hardware, and as stronger biodegradable materials are developed, weight-bearing applications become an option (Wuisman et al. Citation2002). Another advantage is the radiolucency of most polymeric materials: they allow easy visualization of bone ingrowth on radiographs and do not interfere with MRI or CT-scan. However, the device itself is usually not visible on radiographs and requires additional techniques (e.g. MRI) to analyze correct insertion (Lohman et al. Citation1999, Heidemann and Gerlach Citation2002).
Radio-opaque markers—usually tantalum (Ta) marker balls—are often embedded in biodegradable implants to determine the position. These markers are also used alone in radiostereogrammatic analysis (RSA) of prosthesis migration, and have a reliable safety track record. RSA reconstructs the three-dimensional position of marked objects by computer analysis of a two-beam radiograph. However, a substantial number (11–13) of markers placed outside the bone poses a risk of marker migration and third-body wear, which might limit their application (Biedermann et al. Citation2001, Lawrie et al. Citation2003). Moreover, Ta markers might be substituted with biodegradable markers for immediate post-implantation position measurements or a short follow-up period, provided they exhibit adequate radio-opacity.
To our knowledge, this is the first study to quantify the radio-opacity of biodegradable markers specifically designed for position determination in bone. We used clinically approved materials to develop biodegradable pellets of variable radio-opacity which would allow transient position measurement after implantation. The aim of this study was to measure relative radio-opacity in an ex-vivo setting using an aluminium wedge as a standard (Genant and Kuijk Citation2000). Marker positions were measured on rabbit and human femora in an ex-vivo setting, to imitate different anatomical locations.
Materials and methods
Marker production
The markers were a composite of biodegradable calcium phosphate cement and a non-ionic, iodinated, low-osmolar radiological contrast agent for intravascular administration. The chemical structure of calcium phosphate cement, which is used clinically as a bone defect filler, mimics that of natural bone; it is slowly replaced by ingrowing bone after implantation (Blokhuis et al. Citation2000).
Solutions of different iodine content were made from Ultravist (Schering, Weesp, the Netherlands), which contains 300 mg iodine per mL. Distilled water (dH2O) was used as a 0 mg/mL solution. Ultravist was condensed to one-third of the initial volume in a rotational vacuum-concentrator (Christ RVC 2-25, Osterode am Harz, Germany), resulting in a 900-mg/mL solution. A 600-mg/ml solution was made from a 1:1 mixture of the 300 mg/mL and the 900-mg/mL solutions. Mixing these solutions with Biocement D (Merck Bio-Materials, Darmstadt, Germany) in a 2:5 liquid to powder ratio resulted in cement pastes of increasing iodine content: 0, 120, 240, 360 mg iodine/g cement. A paste of 720 mg iodine/g was mixed from a 4:5 liquid to powder ratio, using the 900-mg/mL solution. The iopromide-CaP composites hardened overnight at 37 °C in cylindrical polyethylene molds of 10×10 mm or 1×1 mm (height×diameter) (Stallmann et al. Citation2003). The 10×10 mm markers were used for radio-opacity measurements because the size allowed more measuring positions per marker than the 1×1 mm size.
Radio-opacity measurements
An aluminium wedge of stepwise increasing thickness, 1.0–10.0 mm in 1.0 mm increments, was used as a standard for radio-opacity measurements. Sets of 10×10 mm markers of increasing iodine content were radiographed with the standard. All radiographs were taken using a single bundle set at 33 mAs, 40 kV, 0.6 s (Philips, Eindhoven, the Netherlands). The plate distance was 100 cm. The radiographs were digitized and imported into a graphics program (Paintshop Pro 5.1, Jasc Software, Eden Prarie, MN, USA). Average RGB color values (range 0–255) were measured by randomly selecting 5 positions on every marker and on every step of the aluminium standard. The values of the standard were fitted to a curve, representing the relation between RGB color value and aluminium thickness. Using this curve, the radio-opacity of the markers (expressed in mm aluminium) was calculated.
Ex vivo distance measurements
We drilled three 1.5-mm diameter holes—set apart 20.0 or 30.0 mm—in 8 cadaveric rabbit femora, using a calibrated drill guide with holes at the appropriate distances. Subsequently, 1×1 mm markers of 120 mg iodine per g of cement were placed in the drill-holes and the marked bones were radiographed. With a digital marking gauge, two observers measured the distances between the markers on the radiographs. These were compared to the actual distances of the drill-holes determined by the drill guide.
Molds containing similar 1×1 mm markers were placed on 17 cadaveric human femora (provided by the Department of Anatomy) and radiographed. The distance of those markers interspaced 5, 15, 35 and 45 mm, was measured on the radiographs by two observers and compared to the actual distances of the markers in the mold. To assess the bone quality of the femora, the bone mineral density (BMD) was determined by dual energy radiographic absorptiometry (DXA) with an automated Hologic QDR-2000 system (Genant and Kuijk Citation2000).
Statistics
The correlation of aluminium thickness to RGB color values was described as the R2-value of a curve connecting the average measurements. The radio-opacity of the samples was expressed as mm aluminium. A one-way ANOVA with post-hoc Bonferroni was used to compare radio-opacity between 10×10 mm samples of increasing iodine concentrations; p<0.05 was considered significant. The accuracy of distance measurements was calculated as the root-mean-square error of the measurements (Valstar et al. Citation1997).
Results
At all iodine concentrations except 720 mg/mL, the markers hardened into solid cylinders which could easily be removed from the molds. The latter concentration resulted in slightly doughy samples. Analysis of the wedge radiographs resulted in a linear relationship between aluminium thickness and RGB color value (). The R2 value of the aluminium standard curve was 0.99, which indicates a high correlation between aluminium thickness and RGB color values. The radio-opacity of 10×10 mm markers significantly increased with increasing iodine content; each higher concentration was more radio-opaque than the previous one. There was an initial sharp increase between 0 and 240 mg/g, which leveled off at higher concentrations (). shows a radiograph of the wedge with a range of markers, to illustrate the experimental set-up.
Figure 1. The average RGB color value of the aluminium wedge is plotted as a function of the thickness of the wedge segment. The trend line (R2=0.99) was calculated as: aluminium thickness=(RGB color value + 13.6) / 26.04. Values represent the average±SD of two experiments, totaling at least 10 measurements.
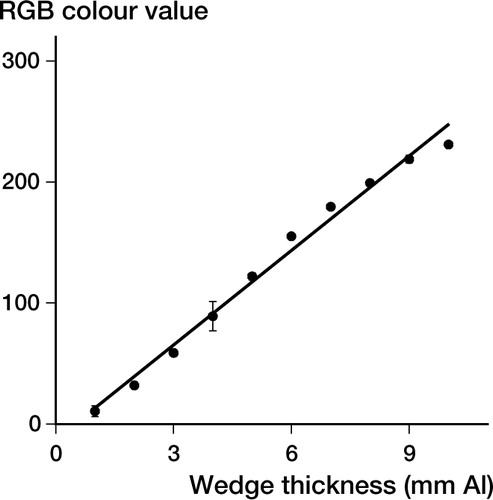
Figure 2. The average calculated radio-opacity (using the aluminium wedge standard curve) of 10×10 mm markers is plotted for different iodine concentrations (0, 120, 240, 360, 720 mg/g). In the range 0–240 mg/g, each increase in iodine concentration caused a significant increase in radio-opacity compared to the previous concentration (a: p<0.001 compared to control; b: p<0.001 compared to 120 mg/g; c: p<0.015 compared to 240 mg/g), while iodine concentrations higher than 240 mg/g caused only a minimal increase in radio-opacity. The results are the average of two experiments, totaling five measurements per marker and at least four markers per iodine concentration.
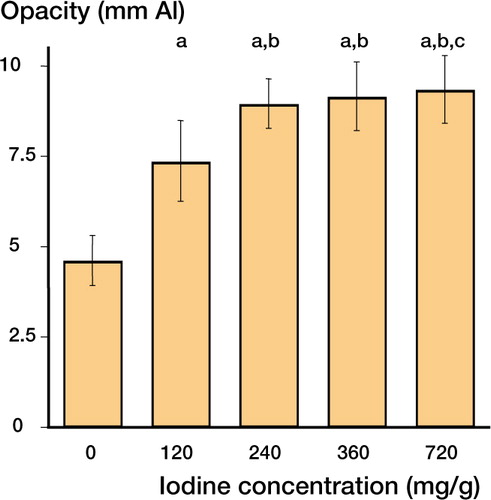
Figure 3. This radiograph of the experimental set-up shows the aluminium wedge and a series of 10×10 mm markers of increasing iodine concentration (0–720 mg/g).
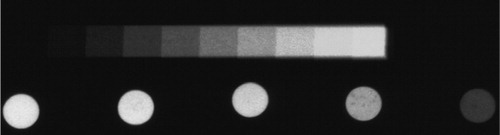
Both in the rabbit and in human femora, radiographs clearly distinguished the 1×1 mm markers from the surrounding bone ( and ). The average BMD of the human femora was 0.74 (SD 0.19) g/cm2. The distance measurements of markers in rabbit and human femora are given in the Table, together with standard deviation and accuracy.
Discussion
Quantification of the experimental markers resulted in a sharp increase in opacity in the concentration interval between 0 and 240 mg iodine per g of cement. Higher iodine concentrations only had a limited effect, suggesting an effective range of iodine concentrations of 120–240 mg/g (). Controlling the radio-opacity allows modification of the biodegradable markers to meet specific requirements determined by anatomical location, clinical situation or implant characteristics.
Distances measured between markers in rabbit femora (n=8) and in human femora (n=17) are shown together with standard deviation and accuracy. The increasing difference between the actual marker distance and the distance measured in the human femora may partly reflect the effect of magnification of the distance measured caused by the distance of the markers from the film, which is more pronounced in the large human bones than in the small rabbit bones
The 120-mg/g implantable markers were clearly visible in rabbit and in human bones, which implies that these markers could be used both in small and large animals, and would be visible in human bones. The standard deviation in rabbit bones was similar to the difference in marker and drill-hole diameter, whereas the magnification of the image due to increased distance of the markers from the plate may have increased the error in the human femora experiment. Several markers embedded in radiolucent implants might allow accurate position determination on post-implantation radiographs. Furthermore, the low standard deviation of the measurements may possibly allow the detection of clinically relevant micro-motion of implants or marked bone segments when analyzed using current RSA software, which increases the accuracy of the measurements (Fukuoka et al. Citation2000, Barker and Donnelly Citation2003).
The linear relationship between wedge thickness and color intensity on radiographs indicates the efficacy of this method in accurately determining radio-opacity of test-samples. The aluminium-wedge method is used clinically in radiographic absorptiometry giving a BMD measurement in which the density of the metacarpals or phalanges is expressed in aluminium equivalent values (Genant and Kuijk Citation2000).
Possible options for the clinical application of our study might include a biodegradable-polymer implant containing calcium phosphate markers, which slowly degrades. The wide range of available biodegradable materials has resulted in an increasing array of well-characterized implants for orthopedic surgery. Several animal studies have described the predictable degradation of calcium phosphates by ingrowing bone in the treatment of bone defects and the augmentation of screw-fixation (Blokhuis et al. Citation2000). Similar to calcium phosphate cements, biodegradable polymeric implants are slowly replaced by ingrowing host cells without significant tissue reaction to the material. Goat studies on spinal fusion showed an improved success rate using poly-L-lactic acid cages compared to titanium cages (van Dijk et al. Citation2002a). In a recent study, biodegradable composite pins of poly(L, DL-lactide) mixed with calcium phosphate caused only mild tissue and lymph node reaction during in-vivo degradation (Prokop et al. Citation2004). These Polypin-C implants have been used for fixation of dislocated radial head fractures with a high clinical success rate (Prokop et al. Citation2002).
The ex-vivo nature of the study limits the potential extrapolation to a clinical setting, regarding both the degradation of the markers and a possible elution of iodine which might decrease radio-opacity over time. Important issues for the in-vivo characterization of biodegradable markers in animal studies would include resorption speed, the effect of resorption on radio-opacity, possible marker migration, and the effect of bone ingrowth and remodeling. Furthermore, when embedded in a biodegradable device, the degradation rate of the device is likely to be the primary determinant of marker resorption, potentially allowing the design of implants with predictable radio-durabil-ity. The biodegradation rate in vivo would depend on anatomical location, vascularization of the site and on implant characteristics such as size, shape and composite materials (Blokhuis et al. Citation2000). Fundamental choices pertaining to the design of such animal studies include: the complexity of the model (singular markers versus embedding in a device), implantation site (soft tissue versus bone), type of animal (small animal versus farm animal), and anatomical location (extremity versus axial skeleton). Pilot studies could include subcutaneous implantation of singular markers in small animals, but ultimately more complex models of e.g. spinal fusion devices with embedded markers in large animals would be indicated. Since the degradation of biodegradable materials has been described in detail in preclinical studies on calcium phosphates and polymer composites (van Dijk et al. Citation2002b, Prokop et al. Citation2004), our study focused on the radio-opacity of the experimental markers.
The cylindrical shape of the markers used was different from the spherical Ta markers. This results in a larger volume and, depending on the angle, may result in a slightly stretched projection of the marker. In RSA studies, correction of this stretch effect might be required. Clinical RSA studies often include rotation measurements and three-dimensional reconstructions of the implant position using sets of markers for each object. Although these were not performed, both are dependent on marker distribution and represent several individual distance measurements such as those performed in this study (Ryd et al. Citation2000).
Iodine toxicity issues in vivo would be improbable for the 1×1 mm implantable markers, since they contain a minimal amount of iodine (±0.02 mg) compared to the amount that is used in radiological contrast agents (300 mg/mL). The clinical accuracy of position determination may be improved further by using sets of at least five markers, advanced computerized reconstruction algorithms, and a dual-beam technique (Ryd et al. Citation2000, Choo and Oxland Citation2003).
We produced biodegradable markers of medically approved materials that could be used for ex-vivo radiographic distance measurements in both human and rabbit bones. The low standard deviation of the measurements allowed the detection of clinically relevant marker distances between the marked segments. As RSA is being used increasingly to monitor the performance of prostheses (Fukuoka et al. Citation2000, Strom et al. Citation2003), these markers seem to offer a valuable opportunity to analyze the position and performance of biodegradable implants without compromising inherent biodegradability. Thus, the current results warrant preclinical studies to optimize iodine-CaP composite markers for different implants and anatomical locations and to evaluate the aptness of such markers.
The assistance of Esther Lok of the Experimental Surgery Department (KDL) in the production of the radiographs is greatly appreciated. The authors also wish to thank Emiel van Haaren for the DXA scans of the human femora. No competing interests declared.
- Barker T M, Donnelly W J. Automated image analysis technique for measurement of femoral component subsidence in total hip joint replacement. Med Eng Phys 2003; 25: 91–7
- Biedermann R, Stockl B, Krismer M, Mayrhofer P, Ornstein E, Franzen H. Evaluation of accuracy and precision of bone markers for the measurement of migration of hip prostheses. A comparison of conventional measurements. J Bone Joint Surg (Br) 2001; 83: 767–71
- Blokhuis T J, Termaat M F, den Boer F C, Patka P, Bakker F C, Haarman H J. Properties of calcium phosphate ceramics in relation to their in vivo behavior. J Trauma 2000; 48: 179–86
- Choo A M, Oxland T R. Improved RSA accuracy with DLT balanced calibration marker distributions with an assessment of initial-calibration. J Biomech 2003; 36: 259–64
- Fukuoka S, Yoshida K, Yamano Y. Estimation of the migration of tibial components in total knee arthroplasty. A roentgen stereophotogrammetric analysis. J Bone Joint Surg (Br) 2000; 82: 222–7
- Genant H K, Kuijk C V. Bone densitometry. Orthopaedic Basic Science2nd Edition, J A Buckwalter, T A Einhorn, S R Simon. American Academy of Orthopaedic Surgeons, Rosemont, IL 2000; 280–8
- Heidemann W, Gerlach K L. Imaging of biodegradable osteosynthesis materials by ultrasound. Dentomaxillofac Radiol 2002; 31: 155–8
- Lawrie D F, Downing M R, Ashcroft G P, Gibson P H. Insertion of tantalum beads in RSA of the hip: variations in incidence of extra-osseous beads with insertion site. Acta Orthop Scand 2003; 74: 404–7
- Lohman M, Partio E K, Vehmas T, Kivisaari A, Kivisaari L. MR imaging in biofix-osteosynthesis. Acta Radiol 1999; 40: 415–7
- Prokop A, Jubel A, Helling H J, Udomkaewkanjana C, Brochhagen H G, Rehm K E. New biodegradable polylactide implants (Polypin-C) in therapy for radial head fractures. Chirurg 2002; 73: 997–1004
- Prokop A, Jubel A, Helling H J, Eibach T, Peters C, Baldus S E, Rehm K E. Soft tissue reactions of different biodegradable polylactide implants. Biomaterials 2004; 25: 259–67
- Ryd L, Yuan X, Lofgren H. Methods for determining the accuracy of radiostereometric analysis (RSA). Acta Orthop Scand 2000; 71: 403–8
- Stallmann H P, Faber C, Slotema E T, Lyaruu D M, Bronckers A L, Nieuw Amerongen A V, Wuisman P I. Continu-ous-release or burst-release of the antimicrobial peptide human lactoferrin 1-11 (hLF1-11) from calcium phosphate bone substitutes. J Antimicrob Chemother 2003; 52: 853–5
- Strom H, Mallmin H, Milbrink J, Petren-Mallmin M, Nivbrant B, Kolstad K. The cone hip stem: a prospective study of 13 patients followed for 5 years with RSA. Acta Orthop Scand 2003; 74: 525–30
- Valstar E R, Spoor C W, Nelissen R G, Rozing P M. Roentgen stereophotogrammetric analysis of metal-backed hemispherical cups without attached markers. J Orthop Res 1997; 15: 869–73
- van Dijk M , Smit T H, Burger E H, Wuisman P I. Bioabsorbable poly-L-lactic acid cages for lumbar interbody fusion: three-year follow-up radiographic, histologic, and histomorphometric analysis in goats. Spine 2002a; 27: 2706–14
- van Dijk M, Tunc D C, Smit T H, Higham P, Burger E H, Wuisman P I. In vitro and in vivo degradation of bioabsorbable PLLA spinal fusion cages. J Biomed Mater Res 2002b; 63: 752–9
- Wuisman P I, van Dijk M, Smit T H. Resorbable cages for spinal fusion: an experimental goat model. J Neurosurg 2002; 97: 433–9