Abstract
Background
Diabetes is associated with inner ear dysfunction. Furthermore, C57BL/6J mice fed high fat diet (HFD), a model for insulin resistance and diabetes, develop endolymphatic hydrops (EH).
Aim
Evaluate if betahistine, spironolactone (aldosterone antagonist) and empagliflozin (sodium –glucose cotransporter2 inhibitor) can prevent EH induced by HFD and explore potential mechanisms.
Methods
C57BL/6J mice fed HFD were treated with respective drug. The size of the endolymphatic fluid compartment was measured using contrast enhanced MRI. Secondarily, mice treated with cilostamide, a phosphodiesterase3 inhibitor, to induce EH and HEI-OC1 auditory cells were used to study potential cellular mechanisms of betahistine.
Results
HFD-induced EH was prevented by betahistine but not by spironolactone and empagliflozin. Betahistine induced phosphorylation of protein kinaseA substrates but did not prevent cilostamide-induced EH.
Conclusions
Betahistine prevents the development of EH in mice fed HFD, most likely not involving pathways downstream of phosphodiesterase3, an enzyme with implications for dysfunction in diabetes. The finding that spironolactone did not prevent HFD-induced EH suggests different mechanisms for EH induction/treatment since spironolactone prevents EH induced by vasopressin, as previously observed.
Significance
This further demonstrates that independent mechanisms can cause hydropic inner ear diseases which suggests different therapeutic approaches and emphazises the need for personalized medicine.
Chinese Abstract
背景:糖尿病与内耳功能障碍有关。 此外, 用高脂肪饮食 (HFD) 喂养的C57BL/6J 小鼠(抗胰岛素和糖尿病的模型)可发生内淋巴积水 (EH)。
目的:评估倍他司汀、螺内酯(醛固酮拮抗剂)和恩格列净(钠-葡萄糖协同转运蛋白 2 抑制剂)是否可以预防 HFD 诱发的 EH, 并探索潜在的机制。
方法:用相应的药物治疗喂食 HFD 的 C57BL/6J 小鼠。 使用对比增强 MRI来测量内淋巴液体室的大小。 其次, 用磷酸二酯酶 3 抑制剂西洛酰胺处理来诱导小鼠的 EH 和 HEI-OC1 听觉细胞。这样的小鼠用于研究倍他司汀的潜在细胞机制。
结果:倍他司汀可预防 HFD 诱导的 EH, 但螺内酯和恩格列净却无法做到。倍他司汀诱导蛋白激酶 A 底物的磷酸化, 但不能防止西洛胺诱导的 EH。
结论:倍他司汀可防止喂食 HFD 的小鼠发生 EH, 很可能不涉及磷酸二酯酶 3 的下游通路, 这是一种对糖尿病功能障碍有影响的酶。 螺内酯不能预防 HFD 诱发的 EH, 这一发现提示, 要用不同的机制来诱导/治疗EH, 因为, 如前所述, 螺内酯可防止加压素诱导的 EH。
意义:我们进一步证明, 独立的机制可以导致内耳水肿病症。我们还提出不同治疗方法, 并强调个体化药物治疗的必要性。
Introduction
A characteristic finding of several inner ear disorders is the expansion of the endolymphatic fluid compartment (EFC) of the inner ear, i.e. endolymphatic hydrops (EH), resulting from deteriorated ion homeostasis and fluid volume regulation [Citation1]. EH may result from a number of processes such as autoimmune disorders, viral infections, electrolyte and fluid imbalance and trauma [Citation1]. EH associated changes in the inner ear are believed to be of significance in relation to the symptoms associated with for example Menière’s disease such as fluctuating hearing loss, tinnitus, vertigo and aural fullness [Citation1,Citation2].
Type 1 and type 2 diabetes negatively impact on the function of the vestibular and auditory systems of the inner ear as shown both in humans and in animal models [Citation3–5]. Although the mechanisms involved have not been identified, we [Citation6–8] and others [Citation9] have shown that the inner ear appears to be a direct target for insulin action and insulin resistance. Also, using magnetic resonance imaging (MRI) with a gadolinium based contrast agent, we have recently shown that C57BL/6J mice fed high fat diet (HFD), a model for insulin resistance and diabetes [Citation10], develop EH [Citation6].
Regarding prevention of EH development, we have shown that spironolactone, an aldosterone antagonist, prevents the development of EH in mice when induced by the cAMP increasing hormone vasopressin and by rolipram, an inhibitor of the cAMP degrading enzyme phosphodiesterase (PDE) 4 but not EH induced by cilostamide, an inhibitor of the cAMP and cGMP degrading enzyme PDE3 [Citation11]. Furthermore, PDE3B dysregulation has been implicated in the context of insulin resistance and diabetes [Citation12]. Thus, the effect of spironolactone on EH appears to be pathway dependent and may provide explanations why certain drugs may be effective in some patients with hydropic ear disease while not in others [Citation11].
Inhibitors of the sodium and glucose co-transporter SGLT2 [Citation13], highly expressed in the kidneys but also in the inner ear [Citation14], are used for the treatment of type 2 diabetes. In addition to a hypoglycemic effect, SGLT2 inhibitors, such as empagliflozin and other, have been shown to have multiple effects that are beneficial in diabetes such as improving insulin sensitivity [Citation13]. Also, by inhibiting reabsorption of sodium in the kidneys, SGLT2 inhibitors have beneficial effects on body fluid homeostasis [Citation13]. The effects of SGLT2 inhibitors on inner ear fluid homeostasis, however, need to be further investigated.
Insulin resistance and type 2 diabetes are frequent companions to weight gain and adiposity. Weight gain and adiposity are also frequent in patients treated with anti-psychotic drugs [Citation15]. In the latter case, betahistine, a histaminergic H1 receptor (Gq) agonist and H3 receptor (Gi) antagonist, has been used as a prevention [Citation15]. The histaminergic system, including H1 and H3 receptors, has indeed been shown to be a potential target for the treatment of obesity and diabetes [Citation16]. Betahistine, has also been extensively used in the symptomatic treatment of vestibular disorders such as Menieres disease, a hydropic inner ear disease [Citation17].
In the present study the primary aim was to test the ability of betahistine, spironolactone and empagliflozin, an SGLT2 inhibitor, to prevent EH development in mice fed a HFD, a model for insulin resistance and type 2 diabetes [Citation10]. Based on the initial findings, we went on to explore potential cellular mechanisms involved in betahistine action using House Ear Institute-Organ of Corti 1 (HEI-OC1) auditory cells [Citation18] and CBA/J mice with cilostamide induced EH [Citation11] as models.
Methods
Animals and high fat diet interventions
Female C57BL/6J mice (Taconic, Ry, Denmark) (8 weeks of age, 22–24 gram) were fed HFD [Citation6] (D12492, 60 E% fat content; Research Diets, New Brunswick, NJ, USA) for 30 days in the absence (12 mice) or presence (11 mice) of betahistine dihydrocloride [Citation17] (Sigma Aldrich 26 mg/kg/day), in the absence (5 mice) or presence (6 mice) of spironolactone [Citation11] (Abcam, 1.2 mg/kg/day) and in the absence (6 mice) or presence (6 mice) of empagliflozin [Citation13] (Cayman Chemical Company, 1.2 mg/kg/day). Epididymal adipose tissue was collected from mice before and after HFD feeding for the analysis of IRS1 (insulin receptor substrate1), a marker for insulin sensitivity [Citation6,Citation10], and Nedd4-2 protein expression [Citation7]. Female CBA/J mice (Janvier Labs) (8 weeks of age, 26–27 gram) were treated with cilostamide (0.5 mg/kg/day) [Citation11,Citation12], another strategy to induce EH targeting the cAMP and cGMP degrading enzyme PDE3 [Citation6,Citation12], without (7 mice) and with (6 mice) betahistine (26 mg/kg/day) [Citation17].
The drugs were administered via micro-osmotic pumps (model 1004; Alzet Corp., Palo Alto, CA) for 30 days. The pumps were implanted surgically in the subcutaneous tissue on the back between the scapulae [Citation11].
All animal procedures were approved by the Regional Ethical Committee for Animal Experiments in Malmö/Lund.
Magnetic Resonance Imaging (MRI)
MRI was performed as previously described [Citation6,Citation11]. Animals were anesthetized with 3.5% isoflurane in a mixture with 400 ml/min air and 50 ml/min oxygen and maintained at 1.5–2% isoflurane inside the magnet. Gadolinium contrast agent (Clariscan, GE Healthcare, USA) (Gadoterate meglumine, 279.3 mg/mL, 0.5 mmol/mL) was administered intraperitoneally (100 ug/20g) in the left lower abdominal quadrant 55–65 min before MRI. The induction chamber was kept warm at 37 °C. Inside the magnet, the respiratory rate of the animal was monitored (SA Instruments Inc, New York, USA) and the body temperature was maintained using a Lauda Rc6 CS recirculating water bath (Köningshofen, Germany).
MRI was performed with a 9.4 T magnet (Agilent Inc., Palo Alto, USA) using Avance III electronics (Bruker, Ettlingen, Germany) [Citation6,Citation11]. The system is equipped with a 12 cm inner diameter gradient system having a maximum gradient strength of 670 mT/m. The animals were imaged using a quadrature transmit/receive cryoprobe (Bruker, Ettlingen, Germany). T1-weighted 3D images were acquired with a gradient echo 3D sequence; repetition time TR: 11 ms, echo time TE: 3.695 ms, number of averages: 2, data matrix size 220 × 220 × 147 pixels, field of view 15 × 15 × 10 mm3, scan time was just below 12 min. Images were reconstructed by zero filling to increase the apparent resolution of the image to a matrix size of 440 × 440 × 294 pixels and an apparent pixel resolution of 0.034 mm.
Quantitative assessment of gadolinium in the endolymphatic relative the perilymphatic
Space
Synedra view personal 16 (Synedra, Innsbruck, Austria), as well as Adobe Photoshop CS5 (Adobe, San Jose, USA), were used for post-production processing of images, for the quantification of signal intensity and for labeling and demonstration of perilymph in the scala tympani (ST) and scala vestibule (SV) and endolymph in the scala media (SM). Images parallel to the modiolus of mouse cochleae, were used for measurements [Citation6,Citation11]. To evaluate the effect of various treatments on the size of the endolymphatic fluid compartment (EFC), the relative area of SM in the basal turn of the cochlea was estimated by calculating the ratio between SM (endolymph, non-contrast enhanced) and SM plus SV (perilymph, contrast enhanced) [Citation6,Citation11]. The ratio of areas was subsequently converted to percentage. The observer assessing and outlining the perilymphatic and endolymphatic sizes, was blinded to the treatments given.
HEI-OC1 auditory cells and culture and preparation of adipose tissue lysates
HEI-OC1 auditory cells were cultured in 6-well plates (TC plate 12 well Standard, Sarstedt, Numbrecht, Germany) under permissive conditions (33 °C, 10% CO2) in high-glucose Dulbecco’s Eagle’s medium (DMEM, Sigma) containing 10% fetal bovine serum (FBS, Sigma) without antibiotics [Citation18]. The cells were then cultured, treated, washed and lysed as previously described [Citation6,Citation7]. In short, after reaching 70–80% confluence cells were washed twice with phosphate-buffered saline (PBS) and preincubated for 2 h in Krebs-Ringer bicarbonate buffer containing 2 mM glucose, 10 mM Hepes, pH 7.4, 120 mM NaCl, 5 mM NaHCO3, 5 mM KCl, 1.2 mM KH2PO4, 2.5 mM CaCl2, 1.2 mM MgSO4 and 0.2% bovine serum albumin (BSA). Thereafter fresh buffer was added and cells were treated with or without betahistine as indicated in the Result section. After treatments, the cells were harvested in a buffer containing 50 mM TES, pH 7.4, 250 mM sucrose, 1 mM EDTA, 2 mM EGTA, 40 mM phenyl-phosphate, 5 mM sodium fluoride, 1 mM dithiothreitol (DTT), 50 µM sodium vanadate, Pefabloc (Sigma), Complete (Roche, protease inhibitors) and 1% NP40 (100 uL/well) (lysis buffer) and subjected to sonication (10 short pulses). The lysates were centrifuged for 5 min at 5000 × g, 4 °C and the supernatants were used for further analysis.
Epididymal adipose tissue was isolated from C57Bl/6J mice before and after HFD feeding and without or with betahistine. The tissue samples were homogenized (20 strokes) in 3 volumes of a lysis buffer. The lysates were centrifuged for 5 min at 5000 × g, 4 °C, the floating fat cake was removed and the infranatants were used for further analysis [Citation6].
SDS-PAGE and Western blotting
HEI-OC1 auditory cells and adipose tissue lysates (20–30 µg protein as measured by Bradford) were mixed with LDS sample buffer 4X (Invitrogen) containing 300 mM DTT and subjected to electrophoresis on 4–12% bisacrylamide gels (Novex, Invitrogen). Proteins were transferred to Immobilon-P, PVDF membranes (Merck Millipore), blocked with 10% milk in Tris-buffered saline tween-20 (TBST: 50 mM Tris, pH 7.6, 150 mM NaCl and 0.1% Tween-20) for 30 min and incubated at 4 °C overnight with primary antibodies as indicated. Membranes were incubated with secondary antibody conjugated with horseradish peroxidase (HRP) for 1 h at room temperature, incubated with SuperSignal West Pico ECL (enzymatic chemiluminescence) reagent (Thermo Scientific, Rockford, USA) for 10 min followed by imaging (Molecular Imager ChemiDoc XRS+, Bio-rad Laboratories, Solna, Sweden) and quantification (Image lab software, version 3.0, Bio-Rad Laboratories [Citation6,Citation7].
Antibodies
The following primary antibodies were used for Western blotting, Nedd4-2, pPKA consensus sequence and IRS1 (Cell Signalling). Anti-rabbit and anti-mouse secondary antibodies conjugated to horseradish peroxidase (HRP) were from Thermo Fisher Scientific (Rockford, IL, USA) and GE Healthcare (Little Chalfont, UK), respectively.
Statistical analysis
Results are expressed as mean ± SEM. Differences between groups were tested for statistical significance using paired or non-paired Student’s t test. p values less than .05 were considered to denote statistical significance. *p < .05, **p < .01, ***p < .001.
Results
Betahistine prevents HFD feeding/insulin resistance induced EH most likely not involving pathways downstream of PDE3
The effects of betahistine on HFD induced EH in C57BL/6J mice were evaluated using contrast enhanced MRI two times for each animal, before and after 30 days. Examples of images are shown in . As shown in and , HFD feeding induced hydrops in the absence of betahistine, however, in the presence of betahistine, EH was prevented. Betahistine did not impact significantly on HFD induced weight gain (). HFD feeding, without or with betahistine, was associated with reduced expression of insulin receptor substrate1 (IRS1) in adipose tissue in agreement with the development of systemic insulin resistance (). On the other hand, HFD feeding increased the expression of Nedd4-2, an effect which was prevented in presence of betahistine.
Figure 1. MRI images from mice fed HFD in the absence or presence of betahistine. MRI was performed at day –1 and day 30. Examples of images parallel to the modiolus of cochlea of mice fed HFD were analyzed by MRI both before and after HFD feeding without or with betahistine is shown. The gadolinium contrast agent enhances the MR signal for perilymph (SV and ST, high signal intensity), but not for endolymph (SM, low signal intensity). The ratio of areas was subsequently converted to percentage as shown in and described in methods. SV, scala vestibule; ST, scala tympani; SM, scala media.
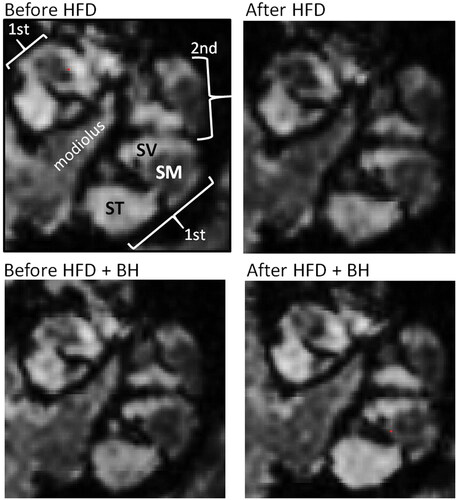
Figure 2. Betahistine prevents HFD feeding induced EH but not weight gain in C57BL/6J. (A–C) C57BL/6J female mice were fed HFD without or with betahistine for 30 days. Contrast enhanced MRI was performed at day –1 and day 30. (A) The relative size of the EFC was estimated for each ear and the same ear was compared before (pre, 12 mice) and after 30 days of treatment (11 mice). Data are presented as means ± SEM shown for all ears (T), right ears (R) and left ears (L). HFD-group, p = 0.020 (T), 0.046 (R), 0.022 (L); HFD + betahistine group, p = 0.11 (T), 0.57 (R), 0.13 (L). (B) Time course in changes in body weight from base-line at 8 weeks of age and during 30 days of treatment. No significant difference in weight between HFD fed and HFD + betahistine fed mice was observed at any time-point. (C) Adipose tissue from control mice (n = 3), mice fed HFD (n = 4) and mice fed with HFD in presence of betahistine (n = 4). Adipose tissue lysates were prepared and IRS1 and Nedd4-2 analyzed by western blotting. Western blot signals were quantified and normalized to non-treated samples. Data are presented as means ± SD. *p < .05, ***p < .001 vs control, fp < .05 vs HFD.
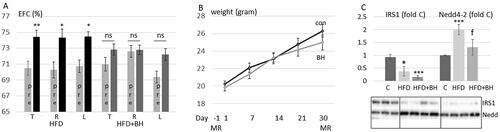
To explore potential roles of protein kinase A and PDE3B downstream pathways as targets for betahistine action we used as models HEI-OC1 auditory cells and CBA/J mice treated with cilostamide to induce EH. Betahistine increased the phosphorylation of protein kinase A substrates () but did not prevent EH induced by cilostamide ().
Figure 3. Betahistine induces phosphorylation of protein kinase A substrates in HEI-OC1 auditory cells and does not prevent EH induced by cilostamide, a PDE3 inhibitor. (A) HEI-OC1 auditory cells were stimulated without or with betahistine for 10 min (n = 3–7). Phosphorylation of protein kinase A (PKA) substrates was analyzed by western blotting. Western blot signals were quantified and normalized to untreated samples. Data are presented as means ± SD. **p < .01 vs control. (B) CBA/J female mice were treated with cilostamide without (7 mice) or with (6 mice) betahistine. The relative size of the EFC was estimated for each ear and the same ear was compared before and after treatment as described in and Methods. Data are presented as means ± SEM. Cilostamide group, p = .037; HFD + betahistine group, p = .0077.
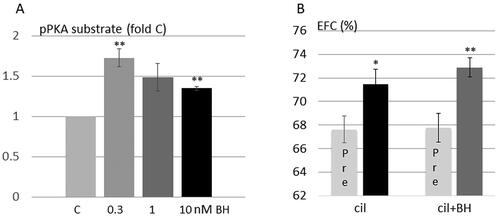
Spironolactone and empagliflozin do not prevent HFD feeding induced EH
As shown in , spironolactone, previously shown to prevent EH induced by vasopressin and the PDE4 inhibitor rolipram but not by cilostamide [11], and the SGLT2 inhibitor empagliflozin did not prevent HFD induced EH. Also, spironolactone and empagliflozin did not impact significantly on HFD induced weight gain ().
Figure 4. Spironolactone and empagliflozin do not prevent HFD-feeding induced EH in C57BL/6J. (A,C) C57BL/6J female mice were fed HFD without (5 mice) or with spironolactone (6 mice) for 30 days. Contrast enhanced MRI was performed at day –1 and day 30. (A) The relative size of the EFC was estimated for each ear and the same ear was compared before (pre) and after 30 days of treatment. Data are presented as means ± SEM shown for all ears (T), right ears (R) and left ears (L). HFD-group, p = 0.0020 (T), 0.0043 (R), 0.103 (L); HFD + spironolactone group, p = 0.0011 (T), 0.013 (R), 0.046 (L). (C) Time course in changes in body weight from base-line at 8 weeks of age and during 30 days on HFD or HFD + spironolactone. No significant difference in weights between HFD fed and HFD + spironolactone treated mice was observed at any time-point. (B,D) C57BL/6J female mice were fed HFD without (6 mice) or with (6 mice) empagliflozin for 30 days. Contrast enhanced MRI was performed at day -1 and day 30. (B) The relative size of the EFC was estimated for each ear before (pre) and after 30 days of treatment. Data are presented as means ± SEM shown for all ears (T), right ears (R) and left ears (L). HFD-group, p = 0.00040 (T), 0.019 (R), 0.012 (L); HFD + empagliflozin group, p = 0.00033 (T), 0.0.061 (R), 0.0012 (L). (D) Time course in changes in body weight from base-line at 8 weeks of age and during 30 days on HFD and HFD + empagliflozin. No significant difference in weights between HFD fed and HFD + empagliflozin treated mice was observed at any time-point.
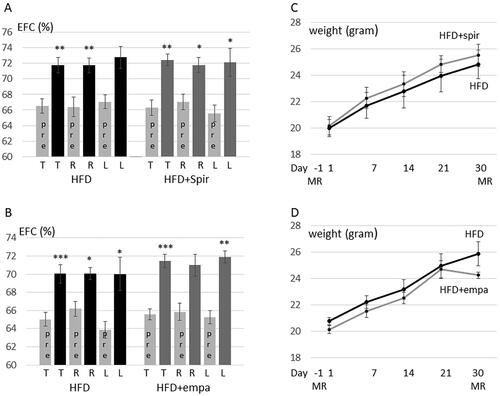
Discussion
In the present study we evaluated the impact of betahistine, spironolactone and empagliflozin on HFD induced EH in C57BL/6J mice using MRI with gadolinium contrast. C57BL/6J mice fed HFD is a commonly used animal model for studies of the pathophysiology of insulin resistance, impaired glucose homeostasis and early type 2 diabetes [Citation10].
New therapy options for the treatment of diabetes include SGLT2 inhibitors that block renal sodium and glucose reabsorption [Citation13]. The kidney and inner ear have a lot in common when it comes to the importance of fluid and ion homeostasis [Citation19,Citation20]. Thus, the SGLT2 inhibitor empagliflozin would be a feasible candidate to prevent the development of hydrops in the HFD fed mouse model of diabetes. However, we could not demonstrate neither beneficial nor non-beneficial effects in the present study. SGLT2 has been shown to be expressed in the inner ear and phlorizin, a SGLT2 inhibitor, has previously been shown to potentiate ototoxicity induced by aminoglycoside possibly due to the ability of SGLT2 to transport gentamicin [Citation14].
Neither did spironolactone prevent hydrops induced by HFD feeding. In previous studies, spironolactone did prevent hydrops induced by vasopressin and the PDE4 inhibitor rolipram but not hydrops induced by the PDE3 inhibitor cilostamide [Citation11], indicating that the ability of spironolactone to prevent hydrops is pathway-dependent. The insulin signaling target PDE3B was previously shown to be downregulated in adipose tissue from over-weight persons and in adipocytes treated with ceramide, a lipid metabolite involved in insulin resistance development [Citation12]. Thus, PDE3B dysregulation could act as a mediator of HFD feeding-induced hydrops in a pathway not targetable by spironolactone.
Betahistine has been used in Menieres disease in humans for several decades, but with little evidence and debated efficacy. Recently, the hitherto most ambitious study of the effect of betahistine, a multi centre double blind, controlled study, with two dose levels, did not show any significant effect [Citation17]. Nevertheless, in the present study betahistine was shown to prevent hydrops induced by HFD feeding. Although the mechanism underlying the anti-hydropic effect of betahistine is not known, the histaminergic system, including H1 and H3 receptors, has been shown to be a potential target for the treatment of obesity and diabetes [Citation16]. In the present study betahistine did not prevent weight gain which could otherwise, at least to some extent, have provided an alternative explanation to the beneficial effect of betahistine on hydrops development. Furthermore, betahistine did not reverse HFD induced downregulation of IRS1 in adipose tissue, a marker for insulin resistance. This suggests a more direct effect of betahistine on EH. On the other hand, HFD feeding resulted in upregulation of Nedd2-4, a regulator of the sodium transporter ENaC [Citation19], in adipose tissue, an effect which was prevented by betahistine. Whether HFD feeding results in upregulation of Nedd4-2 also in the inner ear remains to be explored, however, could involve direct effects of insulin on the inner ear during insulin resistance induced hyperinsulinemia.
The mechanisms underlying the anti EH effect of betahistine in the present study could involve PDE3B. PDE3B dysregulation has been implicated in the context of insulin resistance and diabetes [Citation12] and cilostamide, a PDE3 inhibitor, was shown to induce hydrops [Citation11]. Thus, we tested the ability of betahistine to prevent hydrops induced by cilostamide. However, no effect of betahistine on cilostamide induced EH was observed indicating that betahistine does not target pathways downstream of PDE3B.
Cyclic AMP signaling is compartmentalized and cAMP pools regulated by other PDEs could be involved in the anti-hydropic effect of betahistine. Betahistine has been shown to act as an inverse agonist in the low 0.1–10 nM range and as an agonist in the 100 nM-µM range at the histamine H3 receptor (H3R), a Gi/o protein couplet receptor [Citation16]. In agreement with the reversed agonist effect, 3–10 nM betahistine induced phosphorylation of protein kinase A substrates in HEI-OC1 auditory cells.
In summary, we demonstrate that betahistine prevents HFD induced EH in C57BL/6J mice, a model for insulin resistance and diabetes most likely not involving pathways downstream of PDE3B. On the other hand, spironolactone had no effect on hydrops induced by HFD feeding. However, spironolactone was recently shown to prevent vasopressin and rolipram induced hydrops. Considering the possibilities of several different etiologies to the development of hydrops, it must be reasonable to speculate that there is certain situations where betahistine may have an effect. With further discriminations of different genotypes causing a similar phenotype of hydropic inner ear disease, or Menieres disease, one might hope for a possibility to advice on different therapies for different patients. These results underline the need for personalized medicine in the treatment of hydropic inner ear diseases.
However, the significance of the results showing a beneficial effect with betahistine on EH development in HFD fed mice needs to be further evaluated in clinical studies or on clinical materials evaluating subgroups of patients with Menieres disease and with dysmetabolic condition. Also, in the case of lack of effect of a SGLT2 inhibitor, empagliflozin, more animal studies are needed, for example additional animal models of diabetes could be used. Perhaps different gender of mice could be included and the age of animals could be taken into account.
Acknowledgement
Lund University Bioimaging Center (LBIC) is gratefully acknowledged for providing experimental resources. ED and AP are affiliated with Lund University Diabetes Center (LUDC). HEI-OC1 auditory cells were a kind gift from Dr. Federico Kalinec.
Disclosure statement
No potential conflict of interest was reported by the author(s).
Additional information
Funding
References
- Salt AN, Plontke SK. Endolymphatic hydrops: pathophysiology and experimental models. Otolaryngol Clin North Am. 2010;43(5):971–983.
- Nakashima T, Pyykkö I, Arroll MA, et al. Meniere’s disease. Nat Rev Dis Primers. 2016;2:16028.
- Teng ZP, Tian R, Xing FL, et al. An association of type 1 diabetes mellitus with auditory dysfunction: a systematic review and meta-analysis. Laryngoscope. 2017;127(7):1689–1697.
- Samocha-Bonet D, Wu B, Ryugo DK. Diabetes mellitus and hearing loss: a review. Ageing Res Rev. 2021;71:101423.
- Elangovan S, Spankovich C. Diabetes and auditory-vestibular pathology. Semin Hear. 2019;40(4):292–299.
- Pålbrink AK, Kopietz F, Morén B, et al. Inner ear is a target for insulin signaling and insulin resistance: evidence from mice and auditory HEI-OC1 cells. BMJ Open Diab Res Care. 2020;8(1):e000820.
- Pålbrink AK, Morén B, Stenkula KG, et al. Insulin regulates Nedd4-2 via a PKB-dependent mechanism in HEI-OC1 auditory cells-crosstalks with sphingolipid and cAMP signaling. Acta Otolaryngol. 2022;142(1):6–12.
- Degerman E, Rauch U, Lindberg S, et al. Expression of insulin signalling components in the sensory epithelium of the human saccule. Cell Tissue Res. 2013;352(3):469–478.
- Huerzeler N, Petkovic V, Sekulic-Jablanovic M, et al. Insulin receptor and glucose transporters in the Mammalian Cochlea. Audiol Neurootol. 2019;24(2):65–76.
- Winzell MS, Ahrén B. The high-fat diet-fed mouse: a model for studying mechanisms and treatment of impaired glucose tolerance and type 2 diabetes. Diabetes. 2004;53(suppl_3):S215–S219.
- Degerman E, In 't Zandt R, Pålbrink A, et al. Endolymphatic hydrops induced by different mechanisms responds differentially to spironolactone: a rationale for understanding the diversity of treatment responses in hydropic inner ear disease. Acta Otolaryngol. 2019;139(8):685–691.
- Degerman E, Ahmad F, Chung YW, et al. From PDE3B to the regulation of energy homeostasis. Curr Opin Pharmacol. 2011;11(6):676–682.
- Lingli X, Wenfang X. Characteristics and molecular mechanisms through which SGLT2 inhibitors improve metabolic diseases: a mechanism review. Life Sci. 2022;300:120543.
- Jiang M, Wang Q, Karasawa T, et al. Sodium-glucose transporter-2 (SGLT2; SLC5A2) enhances cellular uptake of aminoglycosides. PLoS One. 2014;9(9):e108941.
- Smith RC, Maayan L, Wu R, et al. Betahistine effects on weight-related measures in patients treated with antipsychotic medications: a double-blind placebo-controlled study. Psychopharmacology. 2018;235(12):3545–3558.
- Provensi G, Blandina P, Passani MB. The histaminergic system as a target for the prevention of obesity and metabolic syndrome. Neuropharmacology. 2016;106:3–12.
- Adrion C, Fischer CS, Wagner J, et al. Efficacy and safety of betahistine treatment in patients with Meniere’s disease: primary results of a long term, multicentre, double blind, randomised, placebo controlled, dose defining trial (BEMED trial). BMJ. 2016;352:h6816.
- Kalinec GM, Webster P, Lim DJ, et al. A cochlear cell line as an in vitro system for drug ototoxicity screening. Audiol Neurotol. 2003;8(4):177–189.
- Kim SH, Marcus DC. Regulation of sodium transport in the inner ear. Hear Res. 2011;280(1–2):21–29.
- Wangemann P. K(+) cycling and its regulation in the cochlea and the vestibular labyrinth. Audiol Neurootol. 2002;7(4):199–205.