Abstract
Background
In temporal bone specimens from long-term cochlear implant users, foreign body response within the cochlea has been demonstrated. However, how hearing changes after implantation and fibrosis progresses within the cochlea is unknown.
Objectives
To investigate the short-term dynamic changes in hearing and cochlear histopathology in minipigs after electrode array insertion.
Material and Methods
Twelve minipigs were selected for electrode array insertion (EAI) and the Control. Hearing tests were performed preoperatively and on 0, 7, 14, and 28 day(s) postoperatively, and cochlear histopathology was performed after the hearing tests on 7, 14, and 28 days after surgery.
Results
Electrode array insertion had a significant effect for the frequency range tested (1 kHz–20kHz). Exudation was evident one week after electrode array insertion; at four weeks postoperatively, a fibrous sheath formed around the electrode. At each time point, the endolymphatic hydrops was found; no significant changes in the morphology and packing density of the spiral ganglion neurons were observed.
Conclusions and Significance
The effect of electrode array insertion on hearing and intracochlear fibrosis was significant. The process of fibrosis and endolymphatic hydrops seemed to not correlate with the degree of hearing loss, nor did it affect spiral ganglion neuron integrity in the 4-week postoperative period.
Chinese Abstract
背景:在长期人工耳蜗植入者的颞骨标本中, 已发现耳蜗内异物反应。 然而, 植入后听力如何变化以及耳蜗内纤维化的发展尚不清楚。
目的:调查插入电极阵列后小型猪的听力和耳蜗组织病理学的短期动态变化。
材料和方法:选择 12 只小型猪进行电极阵列插入(EAI)和对照。 在术前以及术后 0、7、14 和 28 天进行听力测试, 并且在术后7、14、28天听力检查后进行耳蜗组织病理学检查。
结果:电极阵列插入对测试的频率范围 (1kHz–20kHz) 有着显著影响。电极阵列插入后一周有明显渗出; 术后4周, 电极周围形成纤维护套。 各时间点均发现内淋巴积水; 没有观察到螺旋神经节神经元的形态和堆积密度的显著变化。
结论及意义:电极阵列插入对听力和耳蜗内纤维化影响显著。 纤维化过程和内淋巴积水似乎与术后4周期间的听力损失程度无关, 也不影响螺旋神经节神经元的完整性。
Introduction
Cochlear implants (CI) are the most successful neuroprosthesis available. Since its approval by the Food and Drug Administration (FDA) in 1984, the cochlear implant has evolved rapidly over the past 40 years: it has been updated in terms of surgical techniques, electrode array design, speech processing strategies, and programming software; the indications have also been broadened, from the initial use for adults with bilateral severe to profound sensorineural deafness to the present, when the patient’s age has been lowered to 9 months, to include those with unilateral hearing loss and those with auditory neuropathies. As of 2022, one million people worldwide have been helped to return to the world of sound [Citation1].
Studies have found, however, that there is fibrosis and even ossification in the cochlea of long-term CI users [Citation2]. In the meantime, low-frequency residual hearing, critical for patients using combined acoustic and electrical stimulation, is lost as time progresses [Citation3].
Initially, researchers used temporal bone specimens to address the pathological changes to the cochlea during cochlear implantation, both in acquiring temporal bone specimens from patients with CIs and placing CIs on the temporal bone specimens. Electrode array insertion was performed on fresh temporal bone specimens and only immediate postoperative inner ear damage was observed. Whereas temporal bone specimens were collected from patients with CI, observations were limited to morphologic changes in inner ear tissue after long-term use.
In the same period of animal model experiments, although it has the advantage of controlling individual variability, causes of deafness, and surgical techniques, the animal model is most common in rodents, whose species affinity and anatomical structure are different from humans, and also need to design and use special implanted electrodes, which are significantly different from cochlear implants [Citation4]. In mice, the cochlear turns ranged from 1.75 to 2.2, in rats from 2.2 to 2.8, in guinea pigs from 4.0 to 4.5, and in humans from 2.5 to 3.0. The ratio between the quantities of cochlear lymphatic fluid in the three rodents and the human cochlea, based on earlier research, were 0.01:1, 0.04–0.05:1, and 0.17–0.2:1, respectively [Citation5]. Thus, the cochlea of the rodent model is incapable of accommodating a cochlear electrode array for clinical applications.
In the last decade, researchers have found that swine have anatomical, physiological, immunological, and genomic similarities to humans, making them superior to rodent models for research applications [Citation6]. In our previous studies, we have found that the auditory physiological characteristics and cochlea structure of minipigs are similar to those of humans: the frequency distribution(40 Hz-48kHz) and threshold for auditory perception allow coverage of humans(20 Hz-20kHz) [Citation7]; The volume and microstructure of the cochlea are compatible with that of the human body (the ratio of cochlea volume minipig:human is estimated to be 0.5–1.1:1), and a clinical electrode array can be fully implanted through the round window approach, which is an ideal large animal model for the study of cochlear implantation.
The issue of CI causing micro-damage to the cochlea persists. The dynamic pathological process of cochlear fibrosis and what role factors such as surgical trauma and foreign body response (FBR) play are still unclear. In this study, we aim to examine the hearing function and pathophysiological changes in the cochlea after CI in a Chinese domestic piglet model.
Methods
Animals
All animal experiments were approved by ethics from Kunming Institute of Zoology, Chinese Academy of Sciences (IACUC-OE-2023-07-003). A total of twelve 3-month-old domestic minipigs were used in this study. At the beginning of the experiment, the body weight was between 12.2 kg-22.3 kg. Twelve minipigs were performed with unilateral electrode array insertion (right side), and the contralateral sides were used as within-animal controls.
The electrode arrays were selected as CS-10A(TM) from Nurotron with a tip diameter of 0.3 mm and a length of 22 mm. The surgical steps are described below. Hearing tests were performed before surgery (Pre), immediately after surgery (Day 0), one week (Day 7), two weeks (Day 14), and four weeks (Day 28) after surgery. Respectively, cochlear sampling and histomorphometric processing were performed after audiometry at one week, two weeks, and four weeks after surgery (n = 4 for each time point).
Surgical approach
The surgery performed in this study has been described in detail in a previous publication by our research group [Citation8]. Briefly, a retroauricular incision was made to expose the bony surface of the mastoid after bluntly separating the subcutaneous tissue, parotid gland, and neck muscles. The mastoid bone was abraded to expose the external auditory canal, and the mucous membrane of the posterior wall of the external auditory canal was freed to reveal the tympanic chamber and fully disclose the round window membrane. The round window membrane was opened in a pore-like shape in the EAI group, and the electrode array was implanted slowly, steadily, and continuously (the duration of insertion was 40–60 s). Finally, a small piece of muscle was used to seal the pore, and the incision was closed to complete the procedure.
The electrode array was the Nurotron CS-10A(TM) with 24 electrode contacts and an effective length of 22 mm. The preliminary results showed that there was slight resistance when the electrode array was inserted deeper than the 16th electrode contact. Therefore, in order to avoid insertion trauma, the depth of insertion in this experiment was limited to the 16th electrode contact (13 mm, cover the basal turn of the cochlea).
Auditory brainstem response (ABR) measurements
Hearing tests were conducted on animals one week before surgery to ensure no hearing impairment was present. Animals which had for each ear click thresholds ≤ 25 dB peSPL were selected.
ABR testing was performed in a standardized soundproof booth for animal use. Anesthesia was provided by intramuscular injection of Zoletil 50 (5 mg/kg) with Xylazine (2 mg/kg) and mask inhalation of isoflurane, except for the immediate postoperative audiometry, where surgical anesthesia was sufficiently maintained.
ABR was recorded using a Tucker Davis Technologies (TDT) RZ6 device, and the sound was delivered through a calibrated loudspeaker (TDT, MF1 3027) in the free field condition. During audiometry, a heating blanket was placed underneath the animal to maintain body temperature. Three needle electrodes were inserted into the skin for ABR testing: the recording electrode was on top of the head, the reference electrode was located in the auricle of the test ear, and the ground electrode was in the contralateral auricle.
The ABR stimulus sounds were clicks and tone pip (1 kHz, 2 kHz, 4 kHz, 8 kHz, 16 kHz, and 20 kHz, 4 ms total duration with 1 ms rise/fall time). The stimulation rate was 21 times/second, and the number of presentations for each ABR was 1024 times. During ABR tests after surgery, the left ear was masked by a calibrated 80 dB white noise by an inserted earphone.
Histology
After the hearing data were collected, the minipigs were still under anesthesia for execution and cochlear sampling. After sampling, the cochlea apex was drilled, the oval window and the round window were fully opened, and the cochlea was perfused with 4% paraformaldehyde fixative, followed by immersion at 4 °C for 24 h. The cochlea was then decalcified using 10% EDTA at room temperature for at least four weeks.
The decalcified cochlea specimens were dehydrated, hyalinized, dipped in wax, and embedded in wax blocks so the cochlear axis was parallel to the cutting direction. Sections were made using a Leica RM2245 tissue slicer. When the cochlea appeared to have a three-turn structure, sections were made at a thickness of 4 µm and 30 sections were retained for HE staining and statistical analysis.
Fibrosis ratio as a percentage of fibrous tissue area in scala tympani (fibrous tissue area/scala tympani area) and degree of endolymphatic hydrops as scala media area ratio (a percentage of scala media area/the sum of the scala media and scala vestibuli area) were calculated. The number of spiral ganglion neurons (SGNs) was counted at the cochlear basal, middle, and apical turns. When counting SGNs, there should be a visible nucleus. The Rosenthal’s canal (RC) area was measured to calculate SGNs packing density (the number of SGNs/RC area). ImageJ software was used to measure the areas, including fibrous tissue area, scala tympani area, scala media area, scala vestibuli area and RC area.
Statistical analysis
Statistical analysis was conducted using ImageJ, SPSS 23.0 (IBM Corporation, Armonk, NY, USA), and GraphPad Prism version 8.4.3 (GraphPad Software, San Diego, California, USA). The results were presented as means ± the standard error of measurement (SEM). Statistical analysis was performed using the Mann-Whitney U test and Kruskal-Wallis test. The correlations between hearing loss and intracochlear pathologic changes (fibrosis ratio, scala media area ratio, and the packing density of SGNs) were measured by Spearman’s non-parametric rank correlation. Significance was set at p < 0.05.
Results
Changes in ABR thresholds
In the click ABR test, the hearing thresholds of all experimental animals were less than 20 dB peSPL preoperatively (). In the EAI group, the mean hearing threshold increased to 77 dB peSPL in the immediate postoperative period. It was 74.3 dB peSPL on Day 7 and 67.8 dB peSPL on Day 14 postoperatively (). Hearing thresholds in 4 weeks postoperatively were not statistically significant when comparing with the immediate postoperative hearing thresholds (, Kruskal-Wallis test, p > 0.05). The hearing thresholds did not differ significantly in the contralateral ear over time (Kruskal-Wallis test, p > 0.05).
Figure 1. Analysis of click ABR Threshold Changes. (A) Representative diagram of click ABR for the minipigs. The dotted line represents the hearing threshold of 20 dB. (B) The click ABR threshold (dB) before and after surgery. Statistically significant differences were noted between the EAI and the Control at the four postoperative time points of Day 0 (n = 12), Day 7 (n = 12), Day 14 (n = 8), and Day 28 (n = 4). ****p < 0.0001. (C) The click ABR threshold shift (dB) after surgery. No differences were detected by comparing the respective time points in the EAI or the Control (p > 0.05).
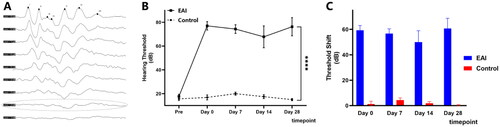
The full-frequency ABR test showed no statistically significant differences on hearing thresholds at each frequency (, Kruskal-Wallis test, p > 0.05). The EAI group’s threshold shift at all frequencies except 2 kHz and 20 kHz showed similar trends to the click ABR outcomes ( and Citation2B). It is worth emphasizing that although the hearing thresholds of all frequencies at four weeks postoperatively were better than the results in the immediate postoperative period, none of the differences were statistically significant ().
Figure 2. Analysis of tone burst ABR Threshold Changes at full frequencies in the EAI. (A) The tone burst ABR threshold (dB) after surgery at 1 kHz–20 kHz in the EAI. No differences were detected by comparing the respective time points at 1 kHz–20 kHz (p > 0.05). (B) The tone burst ABR threshold shift (dB) after surgery. Solid color for Day 0 postoperatively, 50% transparent color for Day 7, block pattern for Day 14, and translucent color for Day 28. Differences were not found in the comparison of the four time points (p > 0.05).
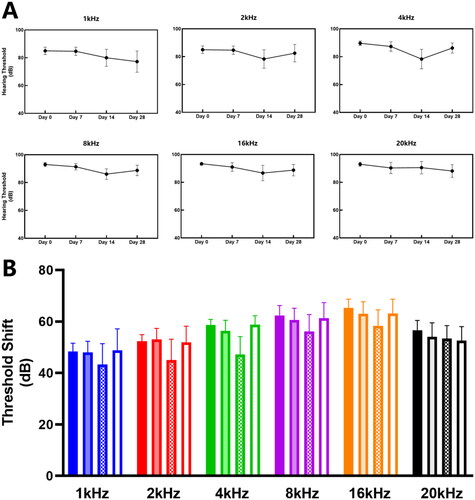
Table 1. The changes of ABR threshold (dB SPL) of different frequencies after surgery.
Cochlea histopathology
Histomorphologic observations were performed for the cochlear specimens of the EAI group and the Control group (). On Day 7 postoperatively, there was a large amount of exudate, including hemorrhage, in the scala tympani, which remained unabsorbed (); on Day 14, the exudate was basically absorbed completely, and a small amount of newborn fibrous tissue appeared (); and on Day 28, the fibrous sheath wrapped around the electrode was formed ().
Figure 3. HE staining of cochlear sections. (A) Typical morphology of the cochlea at 4x magnification. The dashed area is the middle turn of the cochlear. (B) The middle turn of the cochlear at 10× magnification. SV: Scala Vestibuli; SM: Scala Media; ST: Scala Tympani. (C) Spiral ganglion neurons are nested in Rosenthal’s canal at 40× magnification. (D, E, F) Cochlear morphology on postoperative Day 7 (D), Day 14 (E), and Day 28 (F). The arrows mark exudates and newborn fibrous tissue. The placement of the asterisk is the fibrous sheath.
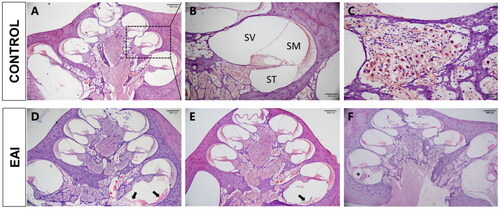
Tissue growth in the scala tympani
In the EAI group, the foreign body response induced by electrode array insertion was localized in the basal turn, followed by the middle turn (). As seen in the scala tympani of the basal turn, unabsorbed exudate was predominant at one week postoperatively, and a small amount of relatively loose fibrous tissue was detected; on Day 14, exudate was significantly absorbed, and a slight increase in newborn tissue was seen, which was not statistically different from that seen at one week postoperatively (); the fibrous sheath wrapped around the periphery of the electrode had been formed on Day 28.
Figure 4. Newborn fibrous tissue within the scala tympani in the EAI. (A) HE stained images of postoperative pathological changes within the scala tympani of the cochlear basal turn (10×). (B) Differences in fibrosis ratio on Day 7, Day 14, and Day 28 postoperatively. The asterisk indicates a significant difference in the fibrosis ratio in comparisons of Day 7 and Day 28 and Day 14 and Day 28 postoperatively; ****p < 0.0001.
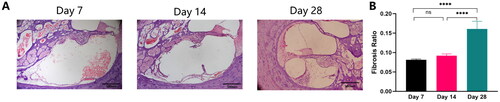
Endolymphatic hydrops in the scala media
Endolymphatic hydrops persisted during the 4-week postoperative period compared with the Control group (). Endolymphatic hydrops was present at one week postoperatively and were still evident at two weeks postoperatively, with no significant change compared to 1 week (). A decline in the percentage was reflected at four weeks postoperatively, but the difference was not statistically significant compared with two weeks postoperatively (, Kruskal-Wallis test, p > 0.05).
Figure 5. Endolymphatic hydrops in the scala media. (A) HE stained images of postoperative pathological changes within the scala media of the cochlear middle turn (10×, the dotted line represents the normal condition of the Reissner’s membrane). (B) Differences in scala media area ratio of the controls and the EAI on Day 7, Day 14, and Day 28 postoperatively; ***p < 0.001, ****p < 0.0001.
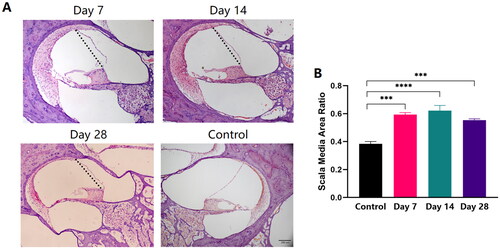
Spiral ganglion Integrity
Morphological observation and densitometry of spiral ganglion neurons were performed on cochlear specimens from different postoperative time points at basal, middle, and apical turns. The results indicated that the morphology of SGNs in Rosenthal’s canal was unaffected the month after electrode array insertion (). In different turns of the cochlea, the density of SGNs revealed no significant differences with short-term postoperative time changes (, Kruskal-Wallis test, p > 0.05).
Figure 6. Spiral Ganglion Integrity in Rosenthal’s Canal. (A) HE stained images of SGNs morphology (10×). (B) Postoperatively compared with controls, SGN density in the cochlear’s basal, middle, and apical turn on Day 7, Day 14, and Day 28. No significant changes in SGN density were demonstrated with postoperative time in either the basal, middle, or apical turn (p > 0.05).
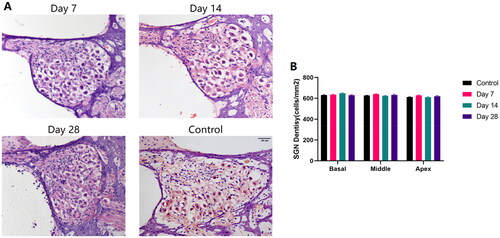
Correlations between ABR threshold shifts and histological measures
We observed a progressive development of fibrosis with postoperative time (, r = 0.9461, p < 0.0001) without correlation with threshold shift (, r = −0.1925, p = 0.7871). As for the endolymphatic hydrops, no correlation was seen either with postoperative time (, r = −0.5026, p = 0.1863) or when correlation analysis with postoperative threshold shift (, r = −0.2688, p = 0.6264).
Figure 7. Correlations Between ABR Threshold shifts and Histological Measures. (A) Fibrosis ratio over time (r = 0.9461, p < 0.0001). (B) The relationship between fibrosis ratio and click ABR threshold shifts (r = −0.1925, p = 0.7871). (C) Scala media area ratio over time (r = −0.5026, p = 0.1863). (D) The relationship between scala media area ratio and click ABR threshold shifts (r = −0.2688, p = 0.6264). The line indicates the linear correlation coefficient.
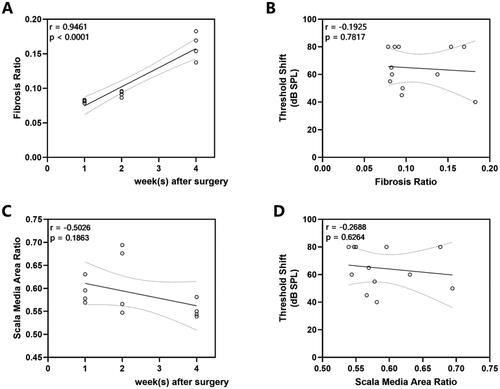
Discussion
To our knowledge, this article may be the first to address the dynamics of cochlear histopathology after electrode array insertion in minipigs [Citation8, Citation9]. The use of clinically applied electrodes for implantation emphasizes the applicability of the selected animal model for such studies. It also encourages academics working in this field, whether the electrodes coated with drugs or novel materials or linked to osmotic pumps, are no longer just theoretical or rodent experiments but have the opportunity to be validated and translated by the miniature porcine model.
Our previous study found that the sensitive frequency distribution and threshold of auditory perception of sound waves in minipigs are almost the same as in humans [Citation7]. Like humans, pigs are born with mature hearing. The human hearing threshold is 20 Hz − 20 kHz, and the sensitive hearing threshold is 1 kHz. In porcine, the frequency range of measurable thresholds is 40 Hz − 48 kHz, and the sensitive hearing threshold is 2 kHz; under acoustic stimuli greater than 20 kHz, the ABR waveform differentiation of porcine is poor. Therefore, this paper selected the six frequencies of 1 kHz, 2 kHz, 4 kHz, 8 kHz, 16 kHz, and 20 kHz for audiometry.
The most important thing is that the size and structure of the inner ear of the minipig match that of the human body, which can be directly connected to clinical deafness diagnosis and treatment technology [Citation7]. In addition, minipigs are the most cost-effective large animal model compared with non-human primates because of their low price, high fertility, and short generation cycle.
The ABR results of the EAI group in and indicated that electrode array insertion had a significant and irreversible effect on hearing. The immediate ABR results after surgery showed that the hearing threshold shift was at 59.3 ± 3.7 dB. It remained at the same level on Day 28 (60.6 ± 8.1 dB). This is similar to the results of the three available studies on cochlear implantation over time in a porcine model [Citation9–11]. The difference is that Yildiz et al. [Citation9] observed that eight weeks after surgery (56 days postoperatively), the ABR audiometry had exceeded 100 dB SPL; Yi [Citation10] and Chen [Citation11], on the other hand, only observed that hearing had decreased to more than 90 dB at one week postoperatively. In contrast, in the present study, after four weeks of continuous postoperative observation, the mean hearing thresholds on 1 kHz and 2 kHz were 77.2 dB and 82.5 dB, with a slight residual hearing ().
The possible reasons were analyzed as follows: the article mentioned that the surgical operation had not reached a mature level, whereas the current process on miniature pigs has been optimized, which ensures the integrity of the external auditory canal, the tympanic membrane, and the ossicular chain, and the standardization of surgical procedures, and the control of surgical time to within 1 − 1.5 h, which may all contribute to the minor residual hearing.
Besides changes in auditory function, we have also focused on foreign body response after electrode array insertion. After implantation, medical implants are recognized by the host immune system as ‘foreign bodies’, leading to a series of interactions between the implant and the host, resulting in a foreign body response. There are four phases: a non-specific protein adsorption phase, an acute inflammatory phase, a chronic inflammatory phase, and a collagen encapsulation and tissue fibrosis phase [Citation12]. The acute inflammatory phase usually subsides within a week, followed by chronic inflammation that lasts up to 3 - 4 weeks with gradual formation of fibrous encapsulation.
In the present study, exudation predominated one week postoperatively; new tissue gradually appeared at 1 to 2 weeks, and a fibrous sheath formed at four weeks. It is in accordance with the pattern of development of foreign body response. An important phenomenon, the formation of new tissue, was similarly confirmed in the study of human temporal bone specimens, from fibrosis to ossification [Citation13].
Recent studies have suggested that residual hearing may be lost by electrode insertion-induced fibrosis, new bone formation, and altered cochlear mechanical sound transmission. Endolymphatic hydrops is also thought to be associated with delayed loss of residual hearing [Citation14]. In the present study, the condition of endolymphatic hydrops lasted in the postoperative period, which was similar to previous studies’ results [Citation4]. However, neither the fibrosis nor endolymphatic hydrops were associated with hearing changes.
The cochlear damage after electrode array insertion seems to be larger in minipigs than in guinea pigs [Citation15, Citation16]. On the one hand, the insertion depth of the guinea pig was limited, ranging from 4 − 4.5 mm, which restricted the effect on the cochlea and hearing. On the other hand, the overall effect on hearing in Andrade’s study[Citation15] was better than that in Jwair’s study [Citation16], and our hearing results were similar to most of Jwair’s study data. Considering the heterogeneity, the sample size should be further expanded.
Based on this, we speculate that the effects on residual hearing are complex and integrated and should not result from a single factor. It may also be related to the inflammatory or immune response in the cochlea, as well as the injury of chronic electrical stimulation.
A major direct influence on cochlear implant outcome is the number and function of spiral ganglion neurons. At one month, we found that the integrity of the spiral ganglion was not significantly affected in all parts of the cochlea. Quesnel [Citation17] and Fayad [Citation18] observed temporal bone specimens from patients with prolonged cochlear implant use and obtained similar findings. In contrast, Trakimas [Citation19] and Knoll [Citation20] found a decrease in the number of SGNs at the cochlear basal turn in cases of electrode translocation or tip folding. Thus, the importance of ‘soft surgery’ with stable and slow implantation of electrodes is highlighted.
Limitations of the study
One limitation is the short time of observation in this study. The duration was four weeks postoperatively, and the possibility of further hearing loss exists. Also, the appearance of ossification was not observed, given the relatively short observation period. As a result, a long-term observation study will be conducted. Another important fact is that electrical stimulation was absent as a factor in the present study, and whether it can be an effective method for positively modulating SGNs function deserves future investigation.
Conclusion
We applied minipigs for dynamic observation of hearing function and cochlear morphology after electrode array insertion. The cochlea is as fragile as thought for the dramatic hearing loss after electrode array insertion. In addition, the change process of electrode array insertion and foreign body response in the short term was clarified. It provides a solid foundation for further investigating how to protect residual hearing better.
Acknowledgments
Haiqiao Du, Jianan Li and Zhifeng Chen contributed equally to this manuscript’s conception, research, and writing in this manuscript.
Disclosure statement
The authors have no conflicts of interest to disclose.
Data availability statement
Data generated or analyzed during this study are available from the corresponding author on request.
Additional information
Funding
References
- Zeng F-G. Celebrating the one millionth cochlear implant. JASA Express Lett. 2022;2(7):077201. doi:10.1121/10.0012825.
- Heutink F, Klabbers TM, Huinck WJ, et al. Ultra-High-Resolution CT to detect intracochlear new bone formation after cochlear implantation. Radiology. 2022;302(3):605–612. Mar doi:10.1148/radiol.211400.
- Gantz BJ, Dunn C, Oleson J, et al. Multicenter clinical trial of the nucleus hybrid S8 cochlear implant: final outcomes. Laryngoscope. 2016;126(4):962–973. doi:10.1002/lary.25572.
- Smeds H, Eastwood HT, Hampson AJ, et al. Endolymphatic hydrops is prevalent in the first weeks following cochlear implantation. Hear Res. 2015;327:48–57. doi:10.1016/j.heares.2015.04.017.
- Reiss LAJ, Kirk J, Claussen AD, et al. Animal models of hearing loss after cochlear implantation and electrical stimulation. Hear Res. 2022;426:108624. doi:10.1016/j.heares.2022.108624.
- Sjöstedt E, Zhong W, Fagerberg L, et al. An atlas of the protein-coding genes in the human, pig, and mouse brain. Science. 2020;367(6482):eaay5947. doi:10.1126/science.aay5947.
- Guo W, Yi H, Ren L, et al. The morphology and electrophysiology of the cochlea of the miniature pig. Anat Rec (Hoboken). 2015;298(3):494–500. doi:10.1002/ar.23095.
- Ji X, Luo Y, Guo W, et al. The miniature pig: a large animal model for cochlear implant research. J Vis Exp. 2022; 28(185). doi:10.3791/64174.
- Yildiz E, Gerlitz M, Gadenstaetter AJ, et al. Single-incision cochlear implantation and hearing evaluation in piglets and minipigs. Hear Res. 2022;426:108644. Dec doi:10.1016/j.heares.2022.108644.
- Yi H, Gw CW, Chen L, et al. Miniature pigs: a large animal model of cochlear implantation. Am J Transl Res. 2016;8(12):5494–5502.
- Chen W, Yi H, Zhang L, et al. Establishing the standard method of cochlear implant in rongchang pig. Acta Otolaryngol. 2017;137(5):503–510. doi:10.1080/00016489.2016.1267406.
- Zhang D, Chen Q, Shi C, et al. Dealing with the foreign-body response to implanted biomaterials: strategies and applications of new materials. Adv Funct Mater. 2020;31(6):2007226. doi:10.1002/adfm.202007226.
- Ishiyama P, Ishiyama G, Lopez IA, et al. Archival human temporal bone: anatomical and histopathological studies of cochlear implantation. J Pers Med. 2023;13(2):352 17 doi:10.3390/jpm13020352.
- Ishiyama A, Doherty J, Ishiyama G, et al. Post hybrid cochlear implant hearing loss and endolymphatic hydrops. Otol Neurotol. 2016;37(10):1516–1521. doi:10.1097/MAO.0000000000001199.
- Andrade JSC, Baumhoff P, Cruz OLM, et al. Cochlear implantation in an animal model documents cochlear damage at the tip of the implant. Braz J Otorhinolaryngol. 2022;88(4):546–555. doi:10.1016/j.bjorl.2020.07.017.
- Jwair S, Ramekers D, Thomeer H, et al. Acute effects of cochleostomy and electrode-array insertion on compound action potentials in normal-hearing Guinea pigs. Front Neurosci. 2023;17:978230. doi:10.3389/fnins.2023.978230.
- Quesnel AM, Nakajima HH, Rosowski JJ, et al. Delayed loss of hearing after hearing preservation cochlear implantation: human temporal bone pathology and implications for etiology. Hear Res. 2016;333:225–234. doi:10.1016/j.heares.2015.08.018.
- Fayad JN, Makarem AO, Linthicum FH.Jr. Histopathologic assessment of fibrosis and new bone formation in implanted human temporal bones using 3D reconstruction. Otolaryngol Head Neck Surg. 2009;141(2):247–252. doi:10.1016/j.otohns.2009.03.031.
- Trakimas DR, Kozin ED, Ghanad I, et al. Human otopathologic findings in cases of folded cochlear implant electrodes. Otol Neurotol. 2018;39(8):970–978. doi:10.1097/MAO.0000000000001886.
- Knoll RM, Trakimas DR, Wu MJ, et al. Intracochlear new Fibro-Ossification and neuronal degeneration following cochlear implant electrode translocation: long-term histopathological findings in humans. Otol Neurotol. 2022;43(2):e153–e164. doi:10.1097/MAO.0000000000003402.