Abstract
To guide the selection of condition indices for juvenile fishes, we compared the ability of several indirect condition indices (those based on length–mass relationships, the hepatosomatic index, and relative lipid estimates from the Distell fish fatmeter) to assess energy density and lipid content of Summer Flounder Paralichthys dentatus, Striped Bass Morone saxatilis, and Atlantic Croakers Micropogonias undulatus. These species use estuarine areas as nurseries, but they have different life history strategies and ecological niches that affect their energy storage strategies. We tested hypotheses that differences in the distribution and role of lipids as energy stores among species would influence the suitability of condition indices for estimating energy and lipid content. Length-based indices were most suitable for estimating energy and lipid content of juvenile Summer Flounder and Striped Bass, suggesting that length-based indices may be appropriate for juveniles that store energy as proteins (i.e., juveniles with low lipid content). The fatmeter suitably predicted energy and lipid content of Atlantic Croakers, indicating its potential for assessing condition of juveniles that store lipids for migration and that display a high range of observed lipid content. Even though a small size range was examined, fish length improved predictions of energy and lipid content for some of the indirect condition indices for Atlantic Croakers and Summer Flounder, suggesting that fish length should be considered when developing relationships among measures of condition. The selection of condition indices should be tailored to the life stage and species of interest based on the energy and lipid storage strategies used by the fish.
Received January 24, 2017; accepted April 24, 2017 Published online August 2, 2017
Condition is often used to describe the general health and nutritional status of individual fish and fish populations (Bolger and Connolly Citation1989; Goede and Barton Citation1990; Brown and Murphy Citation2004; Lloret et al. Citation2014). Indices of condition have been associated with growth (Adams and McLean Citation1985; Gabelhouse Citation1991), swimming performance (Kolok Citation1992; Martinez et al. Citation2003), and other measures of fitness. These associations are based on the premise that the condition of an individual is correlated with its energy or lipid stores, yet this assumption is not routinely examined among species, particularly for juvenile stages. In addition to fitness, the energy density and lipid content of an individual are related to its survival. In both laboratory and wild settings, higher lipid content is associated with improved survival of larval (Lochmann et al. Citation1995; Booth and Alquezar Citation2002) and juvenile (Gardiner and Geddes Citation1980; Thompson et al. Citation1991; Booth and Hixon Citation1999; Simpkins et al. Citation2003) fishes. Individuals in relatively higher condition may represent a small segment of the population, but because they tend to have an increased probability of survival, these individuals may ultimately drive population-level effects (Crowder et al. Citation1992; Stevenson and Woods Citation2006). As a result, fisheries scientists seek appropriate means of identifying well-conditioned individuals to investigate population-level measures of fitness and survival. Unfortunately, the relationships among condition indices and energy and lipid content are seldom compared among species with different ecological niches and life history strategies to assess the versatility of condition indices (but see Copeland et al. Citation2008).
Energy density and lipid content (hereafter, “direct condition indices”) are typically measured using bomb calorimetry and proximate composition analysis. Proximate composition analysis provides an estimate of the proportion of organic (e.g., lipid, protein, and carbohydrate) and inorganic (e.g., water and ash) compounds in the tissues of an individual fish (Stansby Citation1976; Shearer Citation1994). Bomb calorimetry directly estimates the energy density of individuals by measuring the amount of heat released during combustion of dried tissue (Cummins and Wuycheck Citation1971; Glover et al. Citation2010). Proximate composition analysis and bomb calorimetry are time-consuming and lethal methods that are costly when applied to large numbers of individuals. Therefore, these methods have limited utility in large-scale or long-term studies of fish condition.
Indirect indices of fish condition can be used to quickly estimate energy or lipid content and can be applied at lower cost; some indirect indices are nonlethal. Indirect indices are often based on easily measured features (e.g., morphometrics), which may reflect energy or lipid content, although justification for use of these indices is often lacking (Stevenson and Woods Citation2006). Many indirect indices of fish condition may be broadly described as indices based on fish length–mass relationships (hereafter, length-based indices), organ mass (i.e., organosomatic indices), or tissue properties. Fulton’s condition factor (K), a length-based index, is a measure of individual fish robustness because greater body mass at a given length corresponds to better condition (Ricker Citation1975). However, Fulton’s K may be correlated with body size for some species (Cone Citation1989; Sutton et al. Citation2000); therefore, when used to compare groups, Fulton’s K should be measured using fish of similar lengths (Bolger and Connolly Citation1989; Anderson and Neumann Citation1996). Relative condition factor (Kn) can compensate for size-dependent changes in condition (Le Cren Citation1951); however, Kn can be compared only among populations that share a common allometric growth relationship (Anderson and Neumann Citation1996).
Organosomatic indices use the mass of the liver, gonads, or other viscera and express condition as the proportion of mass that is attributable to a particular organ. The hepatosomatic index (HSI) is believed to be a good measure of condition because some fish species store lipids in the liver (Jensen Citation1979; Lambert and Dutil Citation1997) and because the liver is sensitive to feeding history (Heidinger and Crawford Citation1977; Foster et al. Citation1993). However, in species that do not use the liver as a significant storage organ, HSI may be poorly correlated with whole-fish lipid content (Peters et al. Citation2007).
Indirect condition indices may also be derived from tissue properties. Using the Distell fish fatmeter (hereafter, “fatmeter”), the transmission of low-power microwaves through tissues can be used to estimate lipid content (Kent Citation1990). The fatmeter measures the water content of the tissues beneath the skin, which relates to the amount of lipid stored in subdermal reserves and associated peripheral muscle tissues (hereafter, subdermal lipids). For many species, measures of subdermal lipid content from the fatmeter are well correlated with whole-fish or fillet lipid content measured by proximate composition analysis (0.58 ≤ r2 ≤ 0.87; Vogt et al. Citation2002; Hendry and Beall Citation2004; Bransden et al. Citation2007; Kaga et al. Citation2009; Saillant et al. Citation2009; Van Sang et al. Citation2009; Caldwell et al. Citation2013; Mesa and Rose Citation2015), but these relationships have often been developed for adult fish only, and the species investigated thus far are those that are likely to accumulate large lipid stores as adults. Only one study explicitly examined the lipid content of juvenile fish with the fatmeter (Hanson et al. Citation2010), and this was for salmonids. The utility of this technique may differ among life history stages and species with different body distributions of proteins and lipids (Lloret et al. Citation2014), but these potential limitations have not been studied to date.
The objective of this study was to assess the ability of various condition indices to predict energy density and lipid content in juvenile (age < 1 year) fishes. We focused on juvenile fish because energy storage at this life stage is not influenced by reproductive cycles. Juveniles of three mid-Atlantic estuarine fishes were examined: the Summer Flounder Paralichthys dentatus, Striped Bass Morone saxatilis, and Atlantic Croaker Micropogonias undulatus. These species were selected because they have different life history strategies and occupy different ecological niches that affect their energy storage strategies. For example, juvenile Summer Flounder and Atlantic Croakers may store energy in preparation for migration, whereas juvenile Striped Bass store energy for estuarine residence during winter (Schloesser and Fabrizio Citation2016). These strategies may influence the distribution and role of lipids as energy stores among species and thus may affect the suitability of condition indices for estimating energy and lipid content. Juvenile Summer Flounder have significantly lower lipid content than juvenile Striped Bass and Atlantic Croakers (Schloesser and Fabrizio Citation2015); therefore, we hypothesized that length-based indices would have a higher correlation with energy and lipid content of Summer Flounder than indices that reflect energy storage (i.e., HSI or relative subdermal lipid estimates from the fatmeter). As they grow, juvenile Atlantic Croakers and Striped Bass accumulate lipids more rapidly than Summer Flounder (Schloesser and Fabrizio Citation2015), suggesting a greater degree of lipid storage. Because adult Striped Bass store lipids primarily in the viscera (Jacobs et al. Citation2013), we suspected that organs in the coelom may reflect lipid storage, and therefore we hypothesized that the relationship between HSI values and energy and lipid content in Striped Bass would be stronger than those for subdermal lipids or length-based indices. Finally, because the majority of juvenile Atlantic Croakers move offshore to the continental shelf during the fall (Miller et al. Citation2003) and subdermal lipid reserves support migration in some species (Leonard and McCormick Citation1999), we expected the relative strength of the relationships for juvenile Atlantic Croakers to be higher for subdermal lipids than for HSI values or length-based indices.
To assess the relative strength of the relationships among indirect condition indices and energy and lipid content for juveniles of these species, several length-based indices, the HSI, and relative lipid estimates from the fatmeter were compared with the total body energy density and lipid content of the same individuals. Because individuals of many fish species show an increase in energy density and lipid content with body size (Shearer Citation1994; Brown and Murphy Citation2004; Wuenschel et al. Citation2006) and because energy storage strategies differ as fish grow (Lloret et al. Citation2014), we also hypothesized that fish length and interactions between length and the indirect condition indices could influence relationships among condition indices.
METHODS
All animal collection, handling, care, and experimental protocols complied with relevant laws and guidelines of the United States and were approved by the College of William and Mary’s Institutional Animal Care and Use Committee (Protocol Number IACUC-2010-07-20-6788-mcfabr). Juvenile fish were euthanized in an ice slurry, as recommended by Blessing et al. (Citation2010).
Field collections
Juvenile Summer Flounder, Striped Bass, and Atlantic Croakers were sampled monthly between August 2010 and August 2013 from Virginia’s estuaries (). Most samples were obtained according to a random stratified design using a 9.14-m otter trawl, as described by Tuckey and Fabrizio (Citation2013). A 4.88-m otter trawl was used to collect Summer Flounder and Atlantic Croakers from coastal lagoons along the eastern shore of Virginia. Although individuals were sampled in all seasons, more individuals were collected at times when they were readily available to the sampling gear (e.g., Striped Bass during winter). Monthly length thresholds were used to identify young-of-the-year fish (Tuckey and Fabrizio Citation2016). After capture, individuals were immediately euthanized and returned to the laboratory, where they were measured for length to the nearest millimeter and for relative lipid content by using the fatmeter (Distell Model FFM-992). Body mass was measured with a Mettler Toledo XA204 balance (accurate to 0.1 mg); fish were then frozen for later processing (HSI and direct condition indices).
Laboratory specimens
Because only a low percentage of wild fish may be in poor condition (Selleslagh and Amara Citation2013), individuals from a food-deprivation experiment were opportunistically used to increase the observed range of energy and lipid content for Summer Flounder and Striped Bass. The range of observed energy and lipid content for wild-caught Atlantic Croakers was acceptably broad such that food deprivation would not have appreciably increased the observed range. Live Summer Flounder and Striped Bass were collected in October 2010 and were maintained in recirculating (300-L) aquaria with filtered York River water at a temperature of 12–17°C and salinity of about 24‰. Three times per week, fish were offered Zeigler Finfish G prepared diets; however, some individuals refused to feed and exhibited a continuous decline in condition. Individuals that refused to feed were euthanized 2–5 months after collection and were examined here to extend the range of observed condition (n = 14 for Summer Flounder; n = 2 for Striped Bass). Individuals that fed were not used for condition index comparisons because differences in energy storage strategies between laboratory held and wild specimens might influence the relationships among condition indices.
Condition indices
Length-based indices, the HSI, and the measurements from the fatmeter were evaluated for their ability to reflect lipid content and energy density of juvenile Summer Flounder, Striped Bass, and Atlantic Croakers. Analyses were focused on young-of-the-year individuals that were large enough to maintain contact between the fish and the fatmeter sensor for condition assessment. We examined Summer Flounder between 156 and 289 mm TL (n = 73), Striped Bass between 117 and 193 mm FL (n = 66), and Atlantic Croakers between 118 and 214 mm TL (n = 72).
In fish, the fat content often decreases from the head to the tail, and large amounts of fat may be stored beneath the skin around the lateral line (Stansby Citation1976). Therefore, we selected a location immediately behind the operculum and parallel to the lateral line as the site at which to estimate lipid content with the fatmeter. Because Summer Flounder store excess lipids in the fin ray musculature (Gaylord et al. Citation2003), this area was evaluated as a potential measurement site; however, this site was eliminated from further consideration due to inconsistent fatmeter measurements. Individual fish were measured with the fatmeter four times on each side of the body. The side of the fish that produced fatmeter measurements with the highest r2 for the relationships with energy and lipid content was identified for each species and was used in subsequent analyses. The ability of the fatmeter to estimate the lipid content of a fish varies and depends on the lipid content of the sample: accuracy is reported as ±1.5% when lipid content is between 2% and 15%; ±2.5% when lipid content is between 16% and 30%; and ±4% when lipid content is over 31% (Distell Citation2010).
Fulton’s K was calculated as
where W is wet body mass (g), L is length (mm), and N is an integer to bring the value of K near 1 (Ricker Citation1975). The relative condition factor (Kn) was calculated as
where a and b are constants describing the allometric relationship for fish in a given population (Le Cren Citation1951). Constants were estimated using nonlinear regression based on lengths and masses from 2,291 Summer Flounder, 624 Striped Bass, and 3,798 Atlantic Croakers collected from Virginia estuaries between 2010 and 2012 (Schloesser Citation2015). Nonlinear least-squares estimation was conducted using the NLIN procedure in SAS version 9.3 (SAS Institute, Cary, North Carolina). The species-specific relationships were
and
Deviations between the observed mass and the mass expected from these nonlinear regressions were calculated as
where MassExpected is the expected mass based on equations (3)–(5). These length–mass deviations, or residual indices from the regression of wet mass on fish length (i.e., residualized wet weight index; Sutton et al. Citation2000), were also assessed for their ability to reflect lipid content and energy density.
For all individuals, the liver was extracted in a manner that minimized loss of body fluids (this was necessary to preserve the accuracy of proximate composition analysis). Liver mass was measured to calculate the HSI as
where Wliver is the wet mass of the liver (g) and W is wet body mass (Jensen Citation1979; Lambert and Dutil Citation1997).
After liver extraction, gut contents were removed in preparation for bomb calorimetry and proximate composition analysis. Whole fish, excluding the gut contents but including the liver, were homogenized in a blender and dried at 55°C for 4–7 d in a drying oven. Dried tissues were pulverized with a mortar and pestle and were partitioned into two samples: one for bomb calorimetry and the other for proximate composition analysis. Dried tissue samples were combusted in a Parr 6300 bomb calorimeter to determine energy density in calories per gram of dry fish tissue, which was then converted to energy density per gram of wet body mass (kJ/g). The bomb calorimeter was calibrated using a benzoic acid standard, and the calibration was checked every 10 measurements (mean difference ± SE = 0.035 ± 0.035 kJ/g; t-test: t26 = 1.0, P = 0.32; this mean difference corresponds to a 0.13% error). Dried tissues for proximate composition analysis (to assess lipid content) were shipped to the Department of Zoology, Southern Illinois University, Carbondale, a laboratory certified in performing these analyses. More specifically, lipid content was determined using a modified Folch extraction technique (a chloroform–methanol mixture was used as the solvent) and was reported as percentage of wet body mass because these units are used in bioenergetics models (Hartman and Brandt Citation1995).
Statistical analyses
The relationships among indirect and direct condition indices were examined with general linear models to determine whether the suitability of condition indices differed among species with different energy storage strategies. The hypothesis that fish length is an important predictor of energy density and lipid content was tested by including fish length as a predictor in the models, and the hypothesis that the relationship between direct and indirect condition indices varies with fish size was tested by including interactions between length and the indirect condition indices. The global model used to examine relationships between indirect and direct condition indices was
where Yi is the loge transformed energy density or lipid content of the ith individual as measured by bomb calorimetry or Folch extraction; β0 is the intercept, representing the overall mean energy or lipid content of individuals; β1 is the slope for the partial regression of energy or lipid content on the indirect condition index (Xi1; as measured by length-based indices, HSI, or the fatmeter), with length and the length × indirect index interaction held constant; β2 is the slope for the partial regression of energy or lipid content on fish length (Xi2), with the indirect condition index and the length × indirect index interaction held constant; β3 is the slope for the partial regression of energy or lipid content on the interaction (Xi3) between fish length and the indirect condition index (as measured by length-based indices, HSI, or the fatmeter), with the indirect condition index and length held constant; and εi is the random unexplained error, which is assumed to be independent and normally distributed.
The indirect condition indices, fish length, and their interaction were considered fixed effects. Because inclusion of the interaction terms resulted in collinearity, all predictors were centered to remove these effects (; Quinn and Keough Citation2002). Energy and lipid content data were loge transformed to improve normality and homogeneity of variance, which are necessary assumptions of these linear models. Fatmeter measurements were loge transformed because this transformation provides the best linear relationship with lipid content (Colt and Shearer Citation2001; Crossin and Hinch Citation2005; Hanson et al. Citation2010). For model selection purposes, general linear models were fitted with the MIXED procedure in SAS version 9.3 using restricted maximum likelihood (REML) for parameter estimation. For each indirect condition index, Akaike’s information criterion corrected for small sample size (AICc; Burnham and Anderson Citation2002) was used to determine whether fish length and the length × indirect index interaction explained additional variation in energy or lipid content. The AICc was then compared among those models to identify the “best” indirect condition index for estimating energy or lipid content for each species. Models that had AICc values within 2 units were considered to have a similar ability to account for variation in energy or lipid content (Burnham and Anderson Citation2002). When the best models included multiple predictors, partial regressions were used to examine the relationship between indirect and direct condition indices (Quinn and Keough Citation2002). The proportion of the total variation in energy and lipid content explained by the indirect condition indices was determined by using an REML-based estimate of r2 (i.e., ), as discussed by Singer (Citation1998). For ease of comparing our results with previously published data, r2 values are also provided for all condition index comparisons, with significance assessed at α = 0.05.
TABLE 1. Descriptive statistics for direct and indirect condition indices (Fulton’s K = Fulton’s condition factor; Kn = relative condition factor; L–M = length–mass; HSI = hepatosomatic index) measured from the same Summer Flounder (n = 73), Striped Bass (n = 66), and Atlantic Croakers (n = 72). The L–M deviations are the deviations from nonlinear regression models for fish length (mm) and mass (g) given by equations (3)–(5). Due to collinearity among predictors, the means of the raw indirect condition data were subtracted from individual observations of condition to estimate relationships between indirect condition indices and energy density (kJ/g of wet mass) or lipid content (g/g of wet mass).
RESULTS
The midline of the body was a feasible fatmeter measurement site for juvenile fish of all three species. At that location, the left side of Striped Bass and an average of measurements from both sides of Atlantic Croakers provided the highest r2 values with energy and lipid content (). Results are reported from fatmeter measurements on the non-eyed side of Summer Flounder ().
TABLE 2. Values of r2 between indirect condition indices (Fulton’s K = Fulton’s condition factor; Kn = relative condition factor; L–M = length–mass; HSI = hepatosomatic index) and loge transformed energy density (kJ/g of wet mass) and lipid content (g/g of wet mass) for juvenile Summer Flounder, Striped Bass, and Atlantic Croakers. Relative estimates of lipid content measured with the fatmeter were taken from both sides of each individual (FatmeterMean represents the average) and loge transformed to identify the “best” location for assessing lipid content. An asterisk denotes the fatmeter location used for index comparisons. All values were significant (P < 0.05) unless indicated by footnotes.
The relative ability of indirect condition indices to estimate energy or lipid content varied depending on species, and not all of the indirect condition indices were suitable surrogates. As hypothesized, the length-based condition index, Fulton’s K, best explained energy and lipid content for Summer Flounder, resulting in models with lower AICc values than those with condition indices associated with lipid storage (HSI values or relative lipid estimates from the fatmeter; ). Food deprivation greatly extended the range of observed energy density and lipid content for juvenile Summer Flounder and improved our ability to detect linear relationships between Fulton’s K and energy and lipid content (, ). For juvenile Striped Bass, length-based condition indices (Fulton’s K and Kn) also outperformed the models that included HSI values and fatmeter measurements (; , ), so our hypothesis that HSI values would be more suitable was not supported. For Striped Bass, Fulton’s K and Kn showed a similar ability to account for variation in energy density. As hypothesized, relative lipid estimates from the fatmeter were reliable predictors of energy and lipid content for juvenile Atlantic Croakers (; , ). The HSI and deviations from the length–mass regression were generally unsuitable for assessing energy and lipid content of these juvenile fishes. Note that values of were higher than the r2 values () when AICc indicated that additional predictors (e.g., length) helped to explain variation in energy or lipid content.
TABLE 3. General linear models used to predict energy density (kJ/g of wet mass) and lipid content (g/g of wet mass) with indirect condition indices (Fulton’s K = Fulton’s condition factor; Kn = relative condition factor; L–M = length–mass; HSI = hepatosomatic index) and fish length for Summer Flounder (n = 73), Striped Bass (n = 66), and Atlantic Croakers (n = 72). Predictors were centered about their means (), and Akaike’s information criterion corrected for small sample size (AICc) was used for final model selection (ΔAICc = difference in AICc value between the given model and the best-performing model). Parameter estimates for final models are presented with 95% confidence intervals. The proportion of the total variation in energy density and lipid content explained by the indirect condition indices was determined by using a restricted maximum likelihood (REML)-based r2 estimate ().
FIGURE 2. (A) Linear regressions (solid lines) of energy density and selected indirect condition indices for Summer Flounder and Striped Bass; (B) regressions of lipid content and selected indirect indices for all three species; and (C) partial regression plot used to assess the relationship between relative lipid estimates measured with the fatmeter and energy density for Atlantic Croakers. The indices shown are those selected from the general linear models based on the lowest Akaike’s information criterion corrected for small sample size (; Fulton’s K = Fulton’s condition factor). The solid symbols indicate laboratory-held individuals; the dashed lines denote 95% confidence intervals around the equations used to predict loge transformed energy and lipid content (see ).
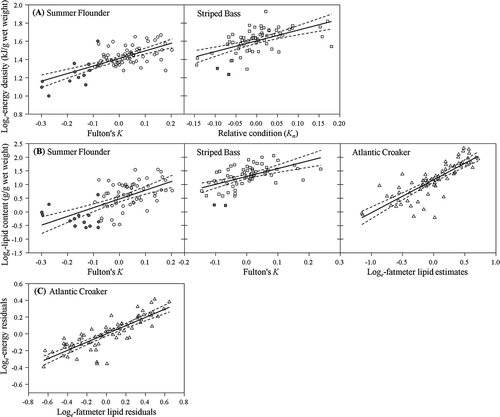
Even though juvenile fish were from a relatively small size range, energy density and lipid content increased with fish size, and length remained an important predictor in some models for juvenile Summer Flounder and Atlantic Croakers (). Notably, predictions of Summer Flounder energy density and lipid content improved when Kn was adjusted for the effects of length. Length was included in all models estimating lipid content of Atlantic Croakers except for Fulton’s K and relative lipid from the fatmeter; length was included in all models estimating energy density of Atlantic Croakers except for Fulton’s K and HSI values. The length × indirect index interaction had a negative effect on lipid content when estimated with length–mass deviations and had a positive effect on estimates of energy density with fatmeter measurements, suggesting that mass is less reflective of lipid content in Atlantic Croakers as they grow because subdermal lipid reserves are more important energy stores for larger Atlantic Croakers. Length did not improve predictions of energy or lipid content for Striped Bass, regardless of the condition index investigated ().
DISCUSSION
Results from this study suggest that the energy and lipid storage strategies used by juvenile fish ultimately influence the utility of condition indices for predicting energy or lipid content. The relative ability of indirect condition indices to estimate energy or lipid content was highest for species displaying a wide range in condition, notably the Atlantic Croaker; furthermore, relationships developed from a limited range of condition often predicted direct metrics with some uncertainty (r2 < 0.50). The importance of size for estimating energy and lipid content differed among species and depended on the condition index evaluated.
Due to the significantly lower lipid storage of juvenile Summer Flounder relative to juvenile Striped Bass and Atlantic Croakers (Schloesser and Fabrizio Citation2015), we expected that length-based indices would be suitable metrics of condition for this species. Flatfishes generally have a relatively low fat content (often ~1%) and a relatively high protein content (~20%) (Stansby Citation1976), and stored proteins may supplement energy needs in some flatfishes during adverse circumstances (e.g., food deprivation; Jobling Citation1980; Maddock and Burton Citation1994). Juvenile Summer Flounder likely also use this strategy given that they store a higher percentage of total energy as proteins compared with juvenile Striped Bass and Atlantic Croakers (Schloesser and Fabrizio Citation2015). Consistent with that expectation, energy content and lipid content of Summer Flounder were best estimated from Fulton’s K. However, the suitability of Fulton’s K as an estimator of condition varies among species. For juveniles of some fish species, r2 values for the relationship between Fulton’s K and energy density or lipid content range from 0.55 to 0.80 (Costopoulos and Fonds Citation1989; Pangle and Sutton Citation2005; Hanson et al. Citation2010), but for other species, the r2 values are less than 0.25 (Trudel et al. Citation2005; Peters et al. Citation2007; Davidson and Marshall Citation2010). Fulton’s K is useful for examining changes in condition of fish among seasons (Heincke Citation1908; cited in Froese Citation2006); the specimens examined here were collected throughout the year and exhibited intra-annual dynamics in condition indices (Schloesser and Fabrizio Citation2016), which helped to provide sufficient contrast in the data to permit description of the relationship between Fulton’s K and energy and lipid content. The additional use of food-deprived individuals furthered the observed contrast in Fulton’s K with energy and lipid content of Summer Flounder. Fulton’s K is also more reliable for predicting lipid content in temperate freshwater fishes that exhibit greater and more variable lipid storage (Mozsár et al. Citation2015). However, intra-annual dynamics in the energy storage strategies of juveniles of the three species we examined (Schloesser and Fabrizio Citation2016) along with the broad spatial domain of the samples may have also introduced uncertainty into the relationships, thus limiting the amounts of the total variability in energy or lipid content that were explained (<50%).
Although we hypothesized that HSI values of juvenile Striped Bass would best estimate energy and lipid content, these variables were better estimated by length-based indices. In fact, the HSI was not a good predictor of energy or lipid content for any of the juvenile fishes examined here. The liver may not be a primary storage organ for lipids in juveniles (Litvin et al. Citation2011), which allocate proportionally more energy to growth rather than storage (Hurst and Conover Citation2003). The allocation of energy to growth (i.e., body mass) will result in less energy storage, and condition indices that reflect lipid storage may be less suitable for estimating energy density than those based on length–mass relationships. Alternatively, condition indices such as HSI that reflect lipid storage may only be suitable if examined during specific time periods when storage may be beneficial to juvenile survival (e.g., during winter instead of throughout the year). Finally, juvenile fish may also store energy as constituents other than lipids, and not all lipids function to store energy. The use of multiple lipid classes and energy storage compounds by individuals may result in weak relationships among indirect and direct condition indices. For example, fish that store energy as protein (instead of lipid) would not only exhibit lower lipid content but also lower energy density because protein contains less energy by mass than lipid. Under these circumstances, establishing a strong relationship between the HSI and lipid content or energy density is difficult due to the low and potentially limited range of observed lipid values (Weisberg Citation2014). The juvenile Striped Bass examined here had protein contents similar to those observed in juvenile Summer Flounder (Schloesser and Fabrizio Citation2015), suggesting that length-based indices may outperform the HSI for individuals with high protein contents.
The utility of the fatmeter for estimating direct condition indices appears to be related to the degree of subdermal lipid storage. As hypothesized, the fatmeter provided better estimates of condition for juvenile Atlantic Croakers than did length-based indices or the HSI. Among the species examined, Atlantic Croakers exhibited a wider range of energy and lipid content (; Schloesser and Fabrizio Citation2015), which was reflected in subdermal lipids. However, the fatmeter may have limited utility for predicting whole-body energy or lipid content for species that display low lipid content, like juvenile Summer Flounder. Although the accuracy of the fatmeter is high (±1.5%) for low-lipid-content individuals (Distell Citation2010), the degree of accuracy may limit applicability of fatmeter-based metrics for low-lipid-content species, particularly in combination with a limited observed range of subdermal lipid stores. Juvenile Atlantic Croakers exhibit a wide range in lipid content because individuals dramatically increase their subdermal lipid stores in preparation for fall migrations from Chesapeake Bay to the coastal ocean (Schloesser and Fabrizio Citation2016). Because migration may be supported by subdermal lipid reserves (Leonard and McCormick Citation1999), the fatmeter may be particularly useful for application to species with a migratory life history strategy involving the juvenile stage. The fatmeter may not be effective for species that are nonmigratory as juveniles or that do not readily utilize subdermal lipid stores, such as Striped Bass (Schloesser and Fabrizio Citation2016). Because the fatmeter measures water content in tissues near the skin, visceral lipid stores are often beyond the measurement area (Davidson and Marshall Citation2010) and therefore would not influence fatmeter measurements.
Lipid storage locations are particularly important to consider when applying condition indices to laboratory-held fish. Relationships between energy and lipid content and indirect approaches based on tissue properties (e.g., the fatmeter) are rarely developed for juvenile fishes, and the few studies that are reported in the literature often used laboratory-held specimens to extend the range of observed energy or lipid content. For juvenile Rainbow Trout Oncorhynchus mykiss obtained from a hatchery, a low r2 value was found between lipid from proximate composition analysis and fatmeter estimates (Hanson et al. Citation2010). Similar issues were noted by Garner et al. (Citation2012) for juvenile Atlantic Croakers when the condition of laboratory-held individuals was examined with bioelectric impedance analysis (BIA), which assesses similar lipid reserves as the fatmeter (Hanson et al. Citation2010). Condition measurements from BIA suggested a loss in condition of laboratory-held individuals despite increases in relative condition and energy density (Garner et al. Citation2012). Correlations among BIA measures and energy density were particularly low for fish that were fed (Garner et al. Citation2012). The differences in composition among wild and laboratory-held specimens are likely to be pronounced when fish are fed to satiation due to differences in energy storage tissues, nutritional value of food resources, and higher activity levels of wild fish. Differences in proximate components have been documented between wild and laboratory-held individuals in several studies, including the quantity and composition of fatty acids (Alasalvar et al. Citation2002), temporal patterns in lipid content (Thompson et al. Citation1991), HSI values (Grant et al. Citation1998), and variations in lipid: protein ratios in muscle tissue (McDonald et al. Citation1998; Morley et al. Citation2012). These observations indicate that feeding fish in a laboratory setting may lead to different tissue-specific distributions of proximate components compared with their wild conspecifics. However, if laboratory-held individuals are deprived of food, body composition may be similar to that of wild specimens that do not feed successfully. Because comparisons of individuals from wild and laboratory settings may not be physiologically meaningful (Copeland and Carline Citation2004), condition indices developed with fish fed in a laboratory should not be applied to wild populations unless it can be shown that the composition, distribution, and temporal patterns of proximate components are similar between wild and laboratory-held fish.
The importance of fish length for estimating energy and lipid content varied among species and among indices. Fish length did not improve model fit for any condition index evaluated for Striped Bass, potentially due to the examination of fish from a relatively restricted size range (i.e., juveniles). The size range of juvenile Striped Bass examined here was narrower than the size ranges observed for juvenile Summer Flounder and Atlantic Croakers; fish length improved model fit for some of the length-based indices applied to Summer Flounder and the majority of the indirect condition indices for Atlantic Croakers. The importance of fish length for describing energy and lipid content of Atlantic Croakers suggests that lipid storage co-occurs with growth. For juvenile Atlantic Croakers and other high-lipid, migratory species, maximal survival may require an energy allocation strategy that balances growth and storage (Post and Parkinson Citation2001).
Interestingly, Fulton’s K did not require adjustments for size to predict energy or lipid content of the species examined, but estimation of energy and lipid content with Kn (a length-independent index) improved by including fish length. The appropriateness of Fulton’s K as a measure of energy or lipid content has been questioned because K may be correlated with body size in species exhibiting allometric growth, such as Summer Flounder and Atlantic Croakers (the exponent in the allometric relationship [b] ± 95% confidence interval was 3.36 ± 0.01 and 3.37 ± 0.02, respectively). Because Fulton’s K is calculated using the length of the individual and thus is not completely independent of length, the effect of size on energy storage capacity may be reasonably reflected in this index, and model fits were not improved by including fish size. Perhaps unsurprisingly, because Kn is independent of length, fish size improved prediction of energy and lipid content, despite the relatively narrow size range investigated here. Although Kn is more suitable than Fulton’s K for comparing condition among individuals from a population exhibiting allometric growth, Kn may not be as beneficial for estimating energy or lipid content of individual fish unless fish size is explicitly incorporated into the models. Similarly, several models estimating energy and lipid content with deviations from a length–mass regression were improved by inclusion of fish length; however, deviations from a length–mass regression were generally unsuitable surrogates for energy and lipid content of juvenile fishes. Deviations from a regression model are less commonly used to investigate fish condition, and this may be due to the inability to meet key assumptions of the length–mass model (e.g., residuals are independent of body size; Garcia-Berthou Citation2001; Green Citation2001; but see Schulte-Hostedde et al. Citation2005). Further investigation into relationships among indirect and direct condition indices should explicitly consider fish size.
In conclusion, the species-specific distribution of proximate constituents, notably lipid, throughout the body may influence the utility of some indirect techniques for assessing energy or lipid content in juvenile fish. Food deprivation may increase the observed range of energy and lipid content and improve the detectability of relationships among measures of condition, but researchers should be cautious about feeding practices that may alter the distribution of energy storage compounds. Length-based measures of condition seem appropriate for species or life stages that have minimal lipid stores or that rely on protein as an energy source (e.g., Summer Flounder). Relative lipid measurements from the fatmeter are appropriate if subdermal lipids are a significant storage depot or if those stores change proportionately with other storage strategies (e.g., Atlantic Croaker). For these and similar species, the energy storage strategies used by fishes with different life history traits and ecological niches can be used to inform the selection of indirect condition indices that may be useful for assessing individual fitness and understanding factors that influence survival. Ultimately, only a comparison of indirect indices to energy or lipid content can determine whether an indirect index will be useful for assessing condition for a given species and life stage.
ACKNOWLEDGMENTS
We thank R. Latour (Virginia Institute of Marine Science [VIMS]), E. Hilton (VIMS), and T. Sutton (Nova Southeastern University) for loaning us equipment needed to conduct this research; J. Trushenski (Southern Illinois University) for conducting proximate composition analysis; and R. Brill and two anonymous reviewers for providing valuable comments on earlier versions of the manuscript. This project would not have been possible without the help of many individuals who collected specimens, particularly the staff of the VIMS Recruitment Program (H. Brooks, A. Comer, J. Conwell, W. Lowery, L. Machut, L. Nys, R. Norris, and T. Tuckey). Virginia Sea Grant, the Virginia Marine Resources Commission, and the VIMS Ziegler Fellowship provided funds to support this research. This paper is Contribution Number 3629 of VIMS, College of William and Mary.
REFERENCES
- Adams, S. M., and R. B. McLean. 1985. Estimation of Largemouth Bass, Micropterus salmoides Lacèpede, growth using liver somatic index and physiological variables. Journal of Fish Biology 26:111–126.
- Alasalvar, C., K. D. A. Taylor, E. Zubcov, F. Shahidi, and M. Alexis. 2002. Differentiation of cultured and wild Sea Bass (Dicentrarchus labrax): total lipid content, fatty acid and trace mineral composition. Food Chemistry 79:145–150.
- Anderson, R. O., and R. M. Neumann. 1996. Length, weight, and associated structural indices. Pages 447–482 in B. R. Murphy and D. W. Willis, editors. Fisheries techniques, 2nd edition. American Fisheries Society, Bethesda, Maryland.
- Blessing, J. J., J. C. Marshall, and S. R. Balcombe. 2010. Humane killing of fishes for scientific research: a comparison of two methods. Journal of Fish Biology 76:2571–2577.
- Bolger, T., and P. L. Connolly. 1989. The selection of suitable indices for the measurement and analysis of fish condition. Journal of Fish Biology 34:171–182.
- Booth, D., and R. Alquezar. 2002. Food supplementation increases larval growth, condition and survival of Acanthochromis polyacanthus. Journal of Fish Biology 60:1126–1133.
- Booth, D. J., and M. A. Hixon. 1999. Food ration and condition affect early survival of the coral reef damselfish, Stegastes partitus. Oecologia 121:364–368.
- Bransden, M. P., S. C. Battaglene, R. M. Goldsmid, G. A. Dunstan, and P. D. Nichols. 2007. Broodstock condition, egg morphology and lipid content and composition during the spawning season of captive Striped Trumpeter, Latris lineata. Aquaculture 268:2–12.
- Brown, M. L., and B. R. Murphy. 2004. Seasonal dynamics of direct and indirect condition indices in relation to energy allocation in Largemouth Bass Micropterus salmoides Lacèpede. Ecology of Freshwater Fish 13:23–36.
- Burnham, K. P., and D. R. Anderson. 2002. Model selection and multimodel inference: a practical information-theoretic approach, 2nd edition. Springer-Verlag, New York.
- Caldwell, L. K., A. L. Pierce, and J. J. Nagler. 2013. Metabolic endocrine factors involved in spawning recovery and rematuration of iteroparous female Rainbow Trout (Oncorhynchus mykiss). General and Comparative Endocrinology 194:124–132.
- Colt, J., and K. D. Shearer. 2001. Evaluation of the use of the Torry Fish Fatmeter to nonlethally estimate lipid in adult salmon. Report of Research to the U.S. Army Corps of Engineers, Portland District, Contract Report W66QKZ00805700, Seattle.
- Cone, R. S. 1989. The need to reconsider the use of condition indices in fishery science. Transactions of the American Fisheries Society 118:510–514.
- Copeland, T., and R. F. Carline. 2004. Relationship of lipid content to size and condition in Walleye fingerlings from natural and aquacultural environments. North American Journal of Aquaculture 66:237–242.
- Copeland, T., B. R. Murphy, and J. J. Ney. 2008. A comparison of relative weight and nutritional status among four fish species in two impoundments. Journal of Freshwater Ecology 23:373–385.
- Costopoulos, C. G., and M. Fonds. 1989. Proximate body composition and energy content of Plaice (Pleuronectes platessa) in relation to the condition factor. Netherlands Journal of Sea Research 24:45–55.
- Crossin, G. T., and S. G. Hinch. 2005. A nonlethal, rapid method for assessing the somatic energy content of migrating adult Pacific salmon. Transactions of the American Fisheries Society 134:181–191.
- Crowder, L. B., J. A. Rice, T. J. Miller, and E. A. Marshall. 1992. Empirical and theoretical approaches to size-based interactions and recruitment variability in fishes. Pages 237–255 in D. L. DeAngelis and L. J. Gross, editors. Individual based models and approaches in ecology. Chapman and Hall, New York.
- Cummins, K. W., and J. C. Wuycheck. 1971. Caloric equivalents for investigations in ecological energetics. International Association of Theoretical and Applied Limnology, Communication 18, Stuttgart, Germany.
- Davidson, D., and C. T. Marshall. 2010. Are morphometric indices accurate indicators of stored energy in Herring Clupea harengus? Journal of Fish Biology 76:913–929.
- Distell. 2010. User manual, Distell Fish Fatmeter Model FFM-992 and Model FFM-692. Distell, West Lothian, Scotland.
- Foster, A. R., D. F. Houlihan, and S. I. Hall. 1993. Effects of nutritional regime on correlates of growth rate in juvenile Atlantic Cod (Gadus morhua): comparison of morphological and biochemical measurements. Canadian Journal of Fisheries and Aquatic Sciences 50:502–512.
- Froese, R. 2006. Cube law, condition factor and weight–length relationships: history, meta-analysis and recommendations. Journal of Applied Ichthyology 22:241–253.
- Gabelhouse, D. W. Jr. 1991. Seasonal changes in body condition of White Crappies and relations to length and growth in Melvern Reservoir, Kansas. North American Journal of Fisheries Management 11:50–56.
- Garcia-Berthou, E. 2001. On the misuse of residuals in ecology: testing regression residuals versus the analysis of covariance. Journal of Animal Ecology 70:708–711.
- Gardiner, W. R., and P. Geddes. 1980. The influence of body composition on the survival of juvenile salmon. Hydrobiologia 69:67–72.
- Garner, S. B., J. L. van der Ham, K. M. Boswell, and J. H. Cowan Jr. 2012. Use of bioelectric impedance analysis to assess total-body condition and predict energy density in juvenile Atlantic Croaker. Transactions of the American Fisheries Society 141:1117–1125.
- Gaylord, T. G., M. H. Schwarz, G. M. Davitt, R. W. Cool, and M. L. Jahncke. 2003. Dietary lipid utilization by juvenile Summer Flounder Paralichthys dentatus. Journal of the World Aquaculture Society 34:229–235.
- Glover, D. C., D. R. DeVries, R. A. Wright, and D. A. Davis. 2010. Sample preparation techniques for determination of fish energy density via bomb calorimetry: an evaluation using Largemouth Bass. Transactions of the American Fisheries Society 139:671–675.
- Goede, R. W., and B. A. Barton. 1990. Organismic indices and an autopsy-based assessment as indicators of health and condition of fish. Pages 93–108 in S. M. Adams, editor. Biological indicators of stress in fish. American Fisheries Society, Symposium 8, Bethesda, Maryland.
- Grant, S. M., J. A. Brown, and D. L. Boyce. 1998. Enlarged fatty livers of small juvenile cod: a comparison of laboratory-cultured and wild juveniles. Journal of Fish Biology 52:1105–1114.
- Green, A. J. 2001. Mass/length residuals: measures of body condition or generators of spurious results? Ecology 82:1473–1483.
- Hanson, K. C., K. G. Ostrand, A. L. Gannam, and S. L. Ostrand. 2010. Comparison and validation of nonlethal techniques for estimating condition in juvenile salmonids. Transactions of the American Fisheries Society 139:1733–1741.
- Hartman, K. J., and S. B. Brandt. 1995. Estimating energy density of fish. Transactions of the American Fisheries Society 124:347–355.
- Heidinger, R. C., and S. D. Crawford. 1977. Effect of temperature and feeding rate on the liver-somatic index of the Largemouth Bass, Micropterus salmoides. Journal of the Fisheries Research Board of Canada 34:633–638.
- Heincke, F. 1908. Bericht über die untersuchungen der biologischen anstalt auf Helgoland zur naturgeschichte der nutzfische (1 April 1905 bis 1 Oktober 1907). [Report on the research of the biological institution of Heligoland on the natural history of crops (1 April 1905 to 1 October 1907).] Pages 66–155 in Die Beteiligung Deutschlands an der Internationalen Meeresforschung, IV & V Jahresbericht. Verlag von Otto Salle, Berlin.
- Hendry, A. P., and E. Beall. 2004. Energy use in spawning Atlantic Salmon. Ecology of Freshwater Fish 13:185–196.
- Hurst, T. P., and D. O. Conover. 2003. Seasonal and interannual variation in the allometry of energy allocation in juvenile Striped Bass. Ecology 84:3360–3369.
- Jacobs, J. M., R. M. Harrell, J. Uphoff, H. Townsend, and K. Hartman. 2013. Biological reference points for the nutritional status of Chesapeake Bay Striped Bass. North American Journal of Fisheries Management 33:468–481.
- Jensen, A. J. 1979. Energy content analysis from weight and liver index measurements of immature Pollock (Pollachius virens). Journal of the Fisheries Research Board of Canada 36:1207–1213.
- Jobling, M. 1980. Effects of starvation on proximate chemical composition and energy utilization of Plaice, Pleuronectes platessa L. Journal of Fish Biology 17:325–334.
- Kaga, T., S. Sato, T. Nagasawa, M. Fukuwaka, T. Nomura, and S. Urawa. 2009. Rapid estimation of lipid content of immature Chum and Pink salmon in the ocean with a handheld microwave meter. North Pacific Anadromous Fish Commission, Document 1208, Vancouver.
- Kent, M. 1990. Hand-held instrument for fat/water determination in whole fish. Food Control 1:47–53.
- Kolok, A. S. 1992. Morphological and physiological correlates with swimming performance in juvenile Largemouth Bass. American Journal of Physiology: Regulatory, Integrative, and Comparative Physiology 263:R1042–R1048.
- Lambert, Y., and J. D. Dutil. 1997. Can simple condition indices be used to monitor and quantify seasonal changes in the energy reserves of Atlantic Cod (Gadus morhua)? Canadian Journal of Fisheries and Aquatic Sciences 54:104–112.
- Le Cren, E. D. 1951. The length–weight relationship and seasonal cycle in gonad weight and condition in the perch (Perca fluviatilis). Journal of Animal Ecology 20:201–219.
- Leonard, J. B. K., and S. D. McCormick. 1999. Effects of migration distance on whole-body and tissue-specific energy use in American Shad (Alosa sapidissima). Canadian Journal of Fisheries and Aquatic Sciences 56:1159–1171.
- Litvin, S. Y., V. G. Guida, and M. P. Weinstein. 2011. Lipid class dynamics and storage depots in juvenile Weakfish Cynoscion regalis and their application to condition assessment. Journal of Fish Biology 78:1294–1310.
- Lloret, J., G. Shulman, and R. M. Love. 2014. Condition and health indicators of exploited marine fishes. Wiley, Chichester, UK.
- Lochmann, S. E., G. L. Maillet, K. T. Frank, and C. T. Taggart. 1995. Lipid class composition as a measure of nutritional condition in individual larval Atlantic Cod (Gadus morhua). Canadian Journal of Fisheries and Aquatic Sciences 52:1294–1306.
- Maddock, D. M., and M. P. M. Burton. 1994. Some effects of starvation on the lipid and skeletal muscle layers of the Winter Flounder, Pleuronectes americanus. Canadian Journal of Zoology 72:1672–1679.
- Martinez, M., H. Guderley, J.-D. Dutil, P. D. Winger, P. He, and S. J. Walsh. 2003. Condition, prolonged swimming performance and muscle metabolic capacities of cod Gadus morhua. Journal of Experimental Biology 206:503–511.
- McDonald, D. G., C. L. Milligan, W. J. McFarlane, S. Croke, S. Currie, B. Hooke, R. B. Angus, B. L. Tufts, and K. Davidson. 1998. Condition and performance of juvenile Atlantic Salmon (Salmo salar): effects of rearing practices on hatchery fish and comparison with wild fish. Canadian Journal of Fisheries and Aquatic Sciences 55:1208–1219.
- Mesa, M. G., and B. P. Rose. 2015. An assessment of morphometric indices, blood chemistry variables and an energy meter as indicators of the whole body lipid content in Micropterus dolomieu, Sander vitreus and Ictalurus punctatus. Journal of Fish Biology 86:755–764.
- Miller, M. J., D. M. Nemerson, and K. W. Able. 2003. Seasonal distribution, abundance, and growth of young-of-the-year Atlantic Croaker (Micropogonias undulatus) in Delaware Bay and adjacent marshes. U.S. National Marine Fisheries Service Fishery Bulletin 101:100–115.
- Morley, J. W., J. A. Buckel, and T. E. Lankford Jr. 2012. Comparing multiple predictors of energy content in juvenile Bluefish. Transactions of the American Fisheries Society 141:1109–1116.
- Mozsár, A., G. Boros, P. Sály, L. Antal, and S. A. Nagy. 2015. Relationship between Fulton’s condition factor and proximate body composition in three freshwater fish species. Journal of Applied Ichthyology 31:315–320.
- Pangle, K. L., and T. M. Sutton. 2005. Temporal changes in the relationship between condition indices and proximate composition of juvenile Coregonus artedi. Journal of Fish Biology 66:1060–1072.
- Peters, A. K., M. L. Jones, D. C. Honeyfield, and J. R. Bence. 2007. Monitoring energetic status of Lake Michigan Chinook Salmon using water content as a predictor of whole-fish lipid content. Journal of Great Lakes Research 33:253–263.
- Post, J. R., and E. A. Parkinson. 2001. Energy allocation strategy in young fish: allometry and survival. Ecology 82:1040–1051.
- Quinn, G. P., and M. J. Keough. 2002. Experimental design and data analysis for biologists. Cambridge University Press, New York.
- Ricker, W. E. 1975. Computation and interpretation of biological statistics of fish populations. Fisheries Research Board of Canada Bulletin 191.
- Saillant, E., M. Dupont-Nivet, M. Sabourault, P. Haffray, S. Laureau, M. Odile-Vidal, and B. Chatain. 2009. Genetic variation for carcass quality traits in cultured Sea Bass (Dicentrarchus labrax). Aquatic Living Resources 22:105–112.
- Schloesser, R. W. 2015. Condition of juvenile fishes in estuarine nursery areas: measuring performance and assessing temporal and spatial dynamics with multiple indices. Doctoral dissertation. College of William and Mary, Williamsburg, Virginia.
- Schloesser, R. W., and M. C. Fabrizio. 2015. Relationships among proximate components and energy density of juvenile Atlantic estuarine fishes. Transactions of the American Fisheries Society 144:942–955.
- Schloesser, R. W., and M. C. Fabrizio. 2016. Temporal dynamics of condition for estuarine fishes in their nursery habitats. Marine Ecology Progress Series 557:207–219.
- Schulte-Hostedde, A. I., B. Zinner, J. S. Millar, and G. J. Hickling. 2005. Restitution of mass-size residuals: validating body condition indices. Ecology 86:155–163.
- Selleslagh, J., and R. Amara. 2013. Effect of starvation on condition and growth of juvenile Plaice Pleuronectes platessa: nursery habitat quality assessment during the settlement period. Journal of the Marine Biological Association of the UK 93:479–488.
- Shearer, K. D. 1994. Factors affecting the proximate composition of cultured fishes with emphasis on salmonids. Aquaculture 119:63–88.
- Simpkins, D. G., W. A. Hubert, C. M. Del Rio, and D. C. Rule. 2003. Physiological responses of juvenile Rainbow Trout fasting and swimming activity: effects on body composition and condition indices. Transactions of the American Fisheries Society 132:576–589.
- Singer, J. D. 1998. Using SAS Proc Mixed to fit multilevel models, hierarchical models, and individual growth models. Journal of Educational and Behavioral Statistics 24:323–355.
- Stansby, M. G. 1976. Chemical characteristics of fish caught in the northeast Pacific Ocean. Marine Fisheries Review 38:1–11.
- Stevenson, R. D., and W. A. Woods Jr. 2006. Condition indices for conservation: new uses for evolving tools. Integrative and Comparative Biology 46:1169–1190.
- Sutton, S. G., T. P. Bult, and R. L. Haedrich. 2000. Relationships among fat weight, body weight, water weight, and condition factors in wild Atlantic Salmon parr. Transactions of the American Fisheries Society 129:527–538.
- Thompson, J. M., E. P. Bergersen, C. A. Carlson, and L. R. Kaeding. 1991. Role of size, condition, and lipid content in the overwinter survival of age-0 Colorado Squawfish. Transactions of the American Fisheries Society 120:346–353.
- Trudel, M., S. Tucker, J. F. T. Morris, D. A. Higgs, and D. W. Welch. 2005. Indicators of energetic status in juvenile Coho Salmon and Chinook Salmon. North American Journal of Fisheries Management 25:374–390.
- Tuckey, T. D., and M. C. Fabrizio. 2013. Influence of survey design on fish assemblages: implications from a study in Chesapeake Bay tributaries. Transactions of the American Fisheries Society 142:957–973.
- Tuckey, T. D., and M. C. Fabrizio. 2016. Estimating relative juvenile abundance of ecologically important finfish in the Virginia portion of the Chesapeake Bay. Virginia Institute of Marine Science, Federal Aid in Sport Fish Restoration, Project F-104-R-20, Annual Report, Gloucester Point.
- Van Sang, N., M. Thomassen, G. Klemetsdal, and H. M. Gjøen. 2009. Prediction of fillet weight, fillet yield, and fillet fat for live River Catfish (Pangasianodon hypophthalmus). Aquaculture 75:127–137.
- Vogt, A., T. R. Gormley, G. Downey, and J. Somers. 2002. A comparison of selected rapid methods for fat measurement in fresh Herring (Clupea harengus). Journal of Food Composition and Analysis 15:205–215.
- Weisberg, S. 2014. Applied linear regression, 4th edition. Wiley, Hoboken, New Jersey.
- Wuenschel, M. J., A. R. Jugovich, and J. A. Hare. 2006. Estimating the energy density of fish: the importance of ontogeny. Transactions of the American Fisheries Society 135:379–385.