ABSTRACT
This article deals with physico-mathematical approaches to anatomy in post-Harveyan physiology. But rather than looking at questions of iatromechanics and animal locomotion, which often attracted this approach, I look at the problem of how blood returned to the heart – a part of the circulation today known as venous return but poorly researched in the early modern period. I follow the venous return mechanisms proposed by lesser-known authors in the mechanization of anatomy, such as Jean Pecquet (1622–1674) and Nicolaus Steno (1638–1686), alongside the more famous Giovanni Alfonso Borelli (1608–1679). Their mechanisms differed only in small details. Yet, these minor differences highlight significant aspects of the mechanization of the life sciences in the seventeenth century. First, they relied more on observations than hitherto acknowledged, even if only indirectly. Second, their mechanisms drew more from the physico-mathematical disciplines than from the trending corpuscularian philosophies of their time. Finally, these mechanisms led to a more accurate understanding of the circulation that remains valid today, thus revealing their cognitive benefits. In short, through the single problem of how blood returned to the heart, this article portrays the increasing complexity of anatomy in the early modern period.
In 1628, William Harvey (1578–1657) dramatically changed the history of anatomy by publishing his theory of the circulation of blood. Harvey’s understanding of the circulation was entirely new but at the same time relatively simple and, because of that, compelling. The blood, pushed by the heart’s contraction, flowed away from the heart into the arteries. It then returned to the heart through the veins during the heart’s expansion. In the following years, as objections were raised, Harvey and other anatomists solidified their understanding of the circulation with further observations.Footnote1 In the final quarter of the seventeenth century, most anatomists and natural philosophers accepted that blood circulated in the body.Footnote2
Yet, despite the simplicity of Harvey’s theory, the circulation of the blood is a much more complex phenomenon than Harvey and his fellow anatomists ever thought. The circulation not only remains a matter of investigation but its research methods are also much broader than what Harvey considered proper of anatomy.Footnote3 Modern physiology is filled with concepts taken from physics and mathematics, and mathematicians and engineers alike research the circulation as well.Footnote4 How did physiology become so complex? And is this only a modern phenomenon?
This article shows that there was already a physico-mathematical approach to physiology taking place in the generation after Harvey.Footnote5 But rather than looking at questions that often attracted this approach, namely animal locomotion and iatromechanics, the article focuses on an ordinary physiological problem.Footnote6 I follow three seventeenth-century responses to the question of how blood returned to the heart – a part of the circulation today known as venous return. Venous return mechanisms are now understood as crucial to the circulation of the blood.Footnote7 Yet, many early modern anatomists, Harvey included, simply did not explore this question. Therefore, this article highlights the important role of less well-known actors in the history of the mechanization of anatomy, such as Jean Pecquet (1622–1674) and Nicolaus Steno (1638–1686), alongside more famous authors like Giovanni Alfonso Borelli (1608–1679).Footnote8 Although their mechanisms of venous return differed in small details, they were very similar. Yet, these minor differences shed light on significant aspects of the mechanization of the life sciences in the seventeenth century. First, their mechanisms relied more on observations than hitherto acknowledged, even if only indirectly. Second, Pecquet and Steno’s mechanisms had little to do with particles in motion or material reductions of the body to machines. Instead, their mechanical examples drew from the physico-mathematical disciplines of their time. Thus, following the actors’ terms and social aspects like the circles they frequented and their professional training, I argue that, in this case, physico-mathematics was central to the mechanization and mathematization of anatomy. Finally, their physico-mathematical explanation of venous return led to a more accurate understanding of the circulation that remains valid, thus revealing the cognitive benefits of such mechanisms.
I start this article with a historiographical discussion of why focusing on physico-mathematics is a fruitful method to study the mechanization of the life sciences. I then introduce how the anatomists Jean Pecquet and Nicolaus Steno explained the return of blood to the heart. Finally, I show how their theories affected Borelli’s understanding of venous return. In a nutshell, through the single problem of how blood returned to the heart, this article shows how anatomy became complex in the early modern period.
The mechanization of anatomy and physico-mathematics
The traditional narrative of the seventeenth-century mechanization of the body begins with William Harvey’s comparison of the heart with a pump. It then follows René Descartes’ (1596–1650) reduction of the body to a hydraulic machine and culminates with Giovanni Alfonso Borelli’s mechanical study of animal motion.Footnote9 Richard Westfall canonized this narrative by describing Borelli’s De motu animalium (Rome, 1680–1) as ‘among the best products of iatromechanics’, by which he meant the application of mechanics to medicine.Footnote10 Yet, for Westfall, iatromechanics was but a ‘puppet regime’ of the mechanical philosophy.Footnote11 For that reason, the same epistemic shortcomings that Westfall found in the mechanical philosophy, namely its lack of observations and the ‘imaginary construction of invisible mechanism’, remained in his analysis of the mechanization of the body.Footnote12 Westfall thus concluded that ‘iatromechanics was simply irrelevant to [modern] biology’ – a claim that I challenge in this article.Footnote13
A generation later, Daniel Garber and Sophie Roux proposed a broader definition of the seventeenth-century mechanical philosophy that avoided Westfall’s reductionisms.Footnote14 According to them, mechanical philosophy encompassed efforts to explain phenomena in terms of particles in motion and an explicit rejection of scholasticism. But it also included a methodological comparison of natural phenomena to machines and the desire to study the laws of nature in mathematical terms. Seventeenth-century natural philosophers adopted these four features in unequal manners. For this reason, historians and philosophers of science now speak of mechanical philosophies in the plural.Footnote15
Focusing on analogies with machines, Sophie Roux suggested to distinguish them from other mechanical explanations that involved particles in motion.Footnote16 She further suggested to carefully discern between the kinds of machines being used in analogies: simple machines like levers, cinematic machines like clocks, or physico-chemical machines like pneumatic gadgets and chemical reactions.Footnote17 She rightly argued that this distinction leads to a better understanding of the epistemology of mechanistic analogies as well as the role of the soul in them. Dennis Des Chenne arrived at similar conclusions by distinguishing ‘mechanism’ as an ontology from ‘mechanism’ as a method.Footnote18 For him, Descartes’ reduction of animals to machines was an ontological step that scholars like Borelli did not take. This allowed them to rely on machines without rejecting final causes in animal motion. Following similar methods of inquiry, other scholars also complicated further the Cartesian and other mechanizations of the body.Footnote19
Along the same lines, but cutting across epistemological discussions, Domenico Bertoloni Meli recently proposed that ‘mechanisms’ should be understood as ‘investigative projects’.Footnote20 This suggestion has two important implications. First, he accepts that early modern scholars used not just machines but also instruments and concepts like springs and permeability to explain natural phenomena.Footnote21 Secondly, he argues that ‘focusing exclusively on the mechanical philosophy leads to a one-sided view’ of mechanism.Footnote22 This approach is historically rewarding because it calls for greater attention to the actors’ terms rather than to philosophical assumptions that they might have.Footnote23 Indeed, regardless of philosophical commitments or worldviews, seventeenth-century scholars regularly used mechanisms in anatomical research. Thus, it is in this non-committal sense that I use terms like mechanism and mechanization in this essay.
This focus on mechanisms alone has proved useful in times during which the mechanical philosophies were not yet prevalent. Aristotle (384–322 BC), Hippocrates (c. 460–370 BC), and Galen (c. 129–216 AD) – the very names supposedly rejected by the mechanical philosophers – all had recourse to mechanisms.Footnote24 In the specific case of ancient anatomy, Erasistratus (c. 304–250 BC) and Galen derived their mechanical analogies from pneumatic and mechanical treatises of their time.Footnote25 More relevant for the early modern period, William Harvey, no mechanical philosopher himself, also used mechanisms in his research regularly.Footnote26 In particular, in a recent analysis of Harvey’s unpublished notes on muscle motion, Peter Distelzweig showed that ‘Harvey establishes a parallel between mechanics and muscle anatomy’ based on the Aristotelian Mechanical Questions.Footnote27 Therefore, just like Galen, Harvey based his mechanisms on the mechanical tradition of his time. Indeed, Harvey’s contemporaries Galileo Galilei (1564–1642) and René Descartes (1596–1650) – much closer to the mechanical philosophies than Harvey – were also strongly influenced by the Aristotelian Mechanical Questions, as Matteo Valleriani and Helen Hattab recently demonstrated.Footnote28 This study of mechanism detached from philosophical commitment thus reveals a more eclectic group of sources than previously assumed.
There is no doubt, however, that recourse to mechanism was more frequent in anatomical research during the second half of the seventeenth century.Footnote29 But this mechanization drew from varied sources and not just from the mechanical philosophies of Descartes, Pierre Gassendi (1592–1655), and others.Footnote30 For instance, writing about medicine in the 1640s and 1650s, Evan Ragland highlights the influence of ancient anatomy in the use of mechanisms by physicians in Leiden.Footnote31 And Justin Smith showed that the most important sources for Gottfried Wilhelm Leibniz’s (1646–1716) mechanization of the body after 1680 were anatomists like Jean Pecquet and Nicolaus Steno, not Descartes and his Cartesian followers.Footnote32 Yet, the question remains: what sources did anatomists working between 1660 and 1680 – like Pecquet, Steno, and Borelli – use?
As I argue in this article, Pecquet, Steno, and Borelli also relied on the traditions of mechanics of their time, just like Galen and Harvey before them. This tradition was then defined by the physico- or mixed mathematical disciplines that studied the application of mathematics to natural phenomena.Footnote33 Physico-mathematics encompassed disciplines like astronomy, optics, mechanics, and hydrostatics. As a continuous development of medieval mixed mathematics, these disciplines are best understood in contrast to pure mathematics like geometry and arithmetic. But mechanics in the 1650s was also different from its medieval and Renaissance ancestor.Footnote34 For instance, seventeenth-century mechanics addressed new machines like siphons and pendulums and explored new topics such as the vacuum and water motion. Moreover, seventeenth-century physico-mathematics gave greater epistemic relevance to experiments and observations. Indeed, Peter Dear explained that the increasing interest in observations in the early modern period occurred precisely due to the diffusion of physico-mathematics’ textbooks.Footnote35
Thus, if physico-mathematics influenced Pecquet and Steno’s mechanisms, then observations also played a role in their development, even if implicitly. That observations mattered in the mechanization of physics and chemistry is already well known by historians. Even famous Cartesian natural philosophers like Christiaan Huygens (1629–1695) and Jacques Rouhault (1618–1672) excelled in experimental research.Footnote36 And, in the words of Antonio Clericuzio, chemistry ‘provided the best experimental evidence to support atomism’ in the early modern period.Footnote37 With regards to the life sciences, anatomists also criticized Cartesian physiology precisely on the basis of sound observational evidence, which the latter apparently lacked.Footnote38 But what exact role did observations play in the new development of a physiological mechanism? This article answers this question by comparing venous-return mechanisms as developed by three different authors.
Finally, it should be noted that physico-mathematics intersected the mechanical philosophies in multiple ways.Footnote39 To mention one example, Robert Boyle (1627–1691), one of the first authors to use the term ‘mechanical philosophy’, made important contributions to physico-mathematics, especially on hydrostatics.Footnote40 But other authors like Blaise Pascal (1623–1662) and Gilles Personne de Roberval (1602–1675) do not fit so well under the category of mechanical philosopher. Interestingly, both Pascal and Roberval frequented the same circles in Paris of Pierre Gassendi (1592–1655), who was one of the leading voices of the mechanical philosophies. This shows that the blending of physico-mathematics and mechanical philosophy also occurred at the social level. Yet, if the mechanical philosophies are difficult to grasp in all their complexities, early modern mechanics and hydrostatics are not, since they were well-defined physico-mathematical disciplines. Indeed, some of its practitioners were de facto opponents of the mechanical philosophies, such as Jesuit mathematicians.Footnote41 Therefore, it is easier to narrow down the influence of physico-mathematics in a certain mechanism than to track the philosophical worldview underlying it. In short, this article does not make claims on the worldviews of the actors here studied, but only on the role of physico-mathematics in their physiological mechanisms.
Jean Pecquet on the circulation of the blood
In his original work on the circulation of the blood, William Harvey argued that the main driver for the circulation was the pulsation of the heart.Footnote42 Writing about blood flow in the veins, Harvey said that the motion of the heart’s ventricles is ‘sufficient for the distribution of blood throughout the whole body and for its drawing forth from the vena cava’.Footnote43 Thus, for Harvey, the pulsation of the heart alone was sufficient to pull the blood out of the vena cava. The vena cava is the largest vein in the body to which all other veins converge.Footnote44 Yet, Harvey was silent on the actual flow inside of it.
One of the first anatomists to comment specifically on blood flow inside the veins was the young Frenchman Jean Pecquet in his book Experimenta nova anatomica (Paris, 1651).Footnote45 This book was a major contribution to the history of anatomy because it showed that the liver – traditionally thought to produce blood from chyle, a milky fluid produced during digestion – did not produce blood after all. Instead, chyle bypassed the liver into the thoracic duct, a new duct discovered by Pecquet that connected the lacteal vessels to the blood circulation.Footnote46 Pecquet’s book was a great success and, within three years of publication, went through an augmented second edition and an English translation.Footnote47 In this book, Pecquet included a dissertation centred on the problem of blood motion in the veins.Footnote48 There, he argued that the original impulse of the heart’s contraction was not enough to account for the return of blood to the heart through the veins, a motion today called venous return.Footnote49 More importantly, in certain parts of venous return, Pecquet noticed that the blood moves in a direction contrary to that of its own weight, or, in modern terms, against the law of gravity.Footnote50 This contrary motion of the blood is particularly problematic in human beings and upright animals because more than half of the blood flows upwards towards the heart through the inferior vena cava ().Footnote51 How can such a large quantity of blood move upwards without the original impulse of the heart?
Figure 1. Gravity-dependent distribution of blood in a dog and in a human. From Furst, The Heart and Circulation, 2nd ed. (Cham: Springer, 2020), p. 324.
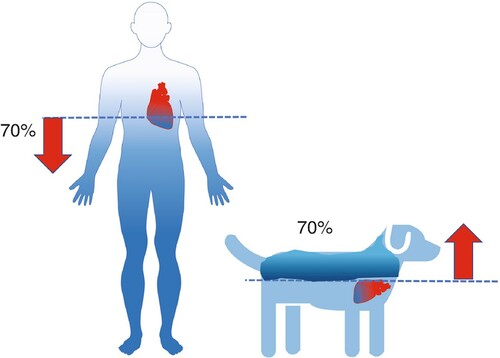
One of the most popular explanations for this blood flow in the veins was – and still is – the siphon effect.Footnote52 This effect equates blood vessels to a siphon by assuming a physical continuity between arteries and veins and by comparing the open ends of the siphon to the heart. In a siphon, the height of the parts of a U-shaped pipe does not affect the flow of the fluid, as long as the place where the siphon starts is higher than the end of the siphon ().Footnote53 In a human body, due to the siphon effect, the height of the heart would not affect the blood flow, as long as the aorta (where the siphon starts) is higher than the vena cava (where the siphon ends). Moreover, since Harvey explained that the heart ejected blood forcefully into the aorta, the pumping of the heart would restart the siphon mechanism, keeping the circulation going. It was, to all effects, an analogy with a hydraulic machine. The problem, argued Pecquet, is that the siphon effect can only be applied to a uniform flow, in which the fluid passes at the same time through all the siphon.Footnote54 But, in the case of a living being, this kind of flow does not occur because the heart’s systole and diastole do not occur at the same time. Moreover, by ligating the veins in the legs of a dog, Pecquet showed that, even though the siphon effect was interrupted, blood that remained in the upper part of the veins continued to flow towards the heart.Footnote55 Therefore, by means of reasoning and observations, Pecquet refuted the siphon effect explanation.
Figure 2. The siphon principle. From Furst, The Heart and Circulation, 2nd ed. (Cham: Springer, 2020), p. 325.
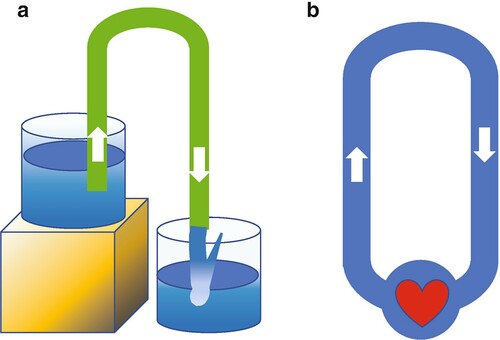
But there was more to Pecquet’s rejection of the siphon. A few years before Pecquet, the German physician Hermann Conring (1606–1681) defended that the upwards motion of blood in the vena cava is ‘in a certain manner like an inflexed siphon’.Footnote56 He then quoted the pneumatic treatises of Hero of Alexandria (c. 10–70 AD) and Girolamo Cardano (1501–1576) to explain how water moved upwards in a siphon.Footnote57 According to them, water moved to avoid creating a vacuum because, according to Aristotle and many others, a vacuum could not exist.Footnote58 Therefore, just like the reason of ‘escaping a vacuum’ [fuga vacui] pulled water upwards in a siphon, so too it pulled blood upwards in the vena cava. But Pecquet’s hydrostatic sources were not Hero nor Cardano. Instead, they were the mathematicians Blaise Pascal, Gilles Personne de Roberval, and Adrien Auzout (1622–1691).Footnote59 In the late 1640s, these three Frenchmen developed new hydrostatical experiments that seemed to show that a vacuum existed. Their work came in sequence with the famous experiment done in 1644 by Evangelista Torricelli (1608–1647), Galileo’s successor as the mathematician of the Medici court in Florence.Footnote60 The Torricellian experiment consisted in turning a mercury-filled tube upside down and dipping it into a larger vessel also filled with mercury. The observable result is that only part of the mercury flowed into the vessel, leaving a column of mercury inside the tube and what seemed to be empty space on top ().
Figure 3. A sophisticated version of the Torricellian experiment, with water on top of the mercury (AC) in the original tube. From Pecquet, Experimenta nova anatomica (Paris, 1651), p. 59. Reproduced by kind permission of the Master and Fellows of Balliol College, University of Oxford.
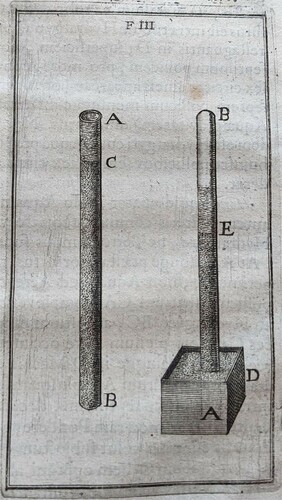
Pecquet met these French mathematicians in meetings that regularly took place in Paris for a selected group of scholars to share scientific ideas.Footnote61 He joined the group in the mid-1640s, right when Pascal, Roberval, and Auzout were working on hydrostatics. Interestingly, not all of them concluded from these experiments that a vacuum existed. Roberval in particular preferred to avoid a commitment to the topic, saying that hydrostatics was concerned with describing and not explaining phenomena because it was a mathematical discipline.Footnote62 But Pascal, who had directed the famous Puy-de-Dôme experiment, was convinced that a vacuum did exist.Footnote63 Regardless, two things regarding the air remained clear to all of them, namely that air had weight and that it had what came to be known as ‘elasticity’ [elater].Footnote64
When Pecquet wrote his book in 1650, he took the weight of the air as a given.Footnote65 That is perhaps why he did not address this matter when discussing the siphon. Pascal had showed that the drive that pushed water upwards inside a siphon was the weight of the air, not the fear of a vacuum.Footnote66 This remained clear when experimenting with siphons in which water did not flow upwards because they were higher than what air pressure could lift the water. Thus, for Pecquet, siphons were not a good machine analogy. But besides the siphon effect, there was another argument that said that the heart’s diastole pulled the blood upwards into the heart, not unlike what Harvey had said. However, Pecquet argued that the heart’s diastole was a consequence of the entry of blood into the heart and not a cause.Footnote67 But Pecquet’s arguments, in this case, also betray his strong interests in physico-mathematics much more clearly.Footnote68 Moreover, they show why the problem of venous return was particularly attractive for those with similar interests.
Pecquet wrote that several anatomists explained the diastole effect by having recourse to an attraction, which was the same as the force provoked by the fear of a vacuum.Footnote69 Indeed, several medical authors addressed attractions in the body at this time.Footnote70 But in the words of Roger French, ‘Pecquet’s work marks something of a watershed in the circulation controversy’.Footnote71 Indeed, Pecquet bluntly stated that an attraction caused by the fear of a vacuum is ‘refuted by means of hydraulic machines’ [hydraulicis exploditur machinis].Footnote72 Therefore, after discussing the heart’s diastole, Pecquet first added a chapter to show that ‘there is not only weight [of the air], but also an elasticity of the rarefied air [which] is demonstrated by experiments’.Footnote73 Pecquet was not the first physician to write about this topic, since the physician Pierre Guiffart (1597–1658) had also published a pamphlet about the vacuum and Pascal’s first hydrostatic experiments in Rouen.Footnote74 Pecquet’s novelty lies instead in inserting these experiments inside an anatomy book. Indeed, after this discussion, and almost as an intrusion in the anatomical text, Pecquet added an entire section entitled ‘Physico-Mathematical Experiments on the Vacuum’.Footnote75 Here, he printed reports of experiments on the vacuum done by Pascal, Roberval, and Auzout, making them available for the first time in print.Footnote76 Pascal’s experiment was the account of the Puy-de-Dôme observations. But the experiments of Roberval and Auzout consisted in observing phenomena within a vacuum, which showed better the effect of the elater of the air. For example, Roberval inserted a fish’s bladder inside a Torricellian tube, so that when the mercury fell, the bladder was left in the empty space and inflated due to low air pressure (). Based on such experiments, Pecquet concluded that there was no attraction of the blood because there was no attraction inside siphons.
Figure 4. Roberval’s experiment. From Pecquet, Experimenta nova anatomica (Paris, 1651), p. 51. Reproduced by kind permission of the Master and Fellows of Balliol College, University of Oxford.
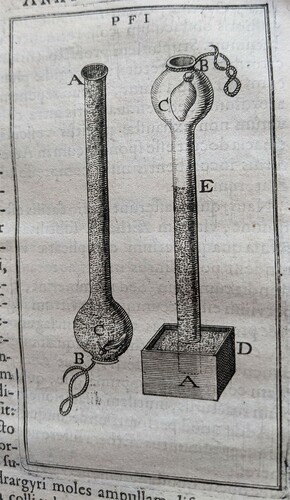
But, if there was no attraction caused by the heart’s diastole, and the siphon effect could not be used, what were ‘the true causes of the motion of blood’, as Pecquet put it?Footnote77 Before this dilemma, Pecquet admitted that the only other explanation had to be related to the compression of blood vessels ‘by the agitation or even the weight of the neighbouring parts’.Footnote78 Then, as if drawing from physico-mathematical vocabulary on the vacuum, he claimed that blood vessels have an ‘innate elasticity [innatus elater]’ that allows them to be contracted and expanded by the passage of blood.Footnote79 This claim was supported either by the vessels’ spontaneous contraction, due to the entry of a large quantity of blood, or violent contraction, due to what Pecquet called the ‘sharpness of bitter humor [humoris acrioris asperitas]’.Footnote80 It is not clear what Pecquet means here, but he gave two examples: that the bitter pancreatic juice wrinkles (and thus longitudinally contracts) the intestines, and that ligatures on veins show they can contract. In the end, Pecquet concluded that the compression of veins was ‘perfected’ by ‘the pressure of breathed air into the lungs and by the endeavour of the muscles adjacent to the pipes [i.e. the blood vessels] whilst they stirred’.Footnote81 For Pecquet, the mechanism that pushed the blood in the veins was the contraction of the veins’ walls, which Borelli later called a ‘peristaltic force’.Footnote82 But Pecquet demonstrated this elasticity of the veins only with reasoning and without observations of the veins. Moreover, he did not develop these ideas further. For instance, he did not reference the specific muscles that worked on venous return nor did he explain how exactly the inspired air pushed the veins.
Curiously, Pecquet answered these questions in his account of the motion of chyle. Moreover, in the case of the chyle, he supported his claims with the observations that composed the main research for this book, described in the book’s first part. Just like in venous return, the upwards motion of the chyle in the thoracic duct also seemed to defy the law of gravity and so there had to be another mechanism to push it forward.Footnote83 Pecquet wrote that the liver, stomach, and other organs in the region of the mesentery move in response to the motion of the diaphragm, which is the muscle below the lungs.Footnote84 Therefore, not just muscles, but also other organs contributed to the motion of chyle. In Pecquet’s words, these organs,
acting as a pestle on the things below, by the weight of their bulk through intervals, not only compel the chyle to depart out of the stomach into the intestines through the pylorus, but also distend their little pores, and in that direction thrust the subtlest substance of the aliment into the lacteals’.Footnote85
Nicolaus Steno’s experience of venous return
As a young medical student, Nicolaus Steno studied Pecquet’s work carefully. In his manuscript student notes, written in 1659 in Copenhagen, Pecquet’s Experimenta nova anatomica (Paris, 1654) is one of the few books that Steno studied from cover to cover.Footnote89 Not only did he excerpt from all sections of Pecquet’s book, but he also annotated his own excerpts thoroughly. Moreover, these manuscript notes reveal Steno’s early interest in physico-mathematics, especially because of excerpts that Steno took from the hydrostatics chapter of Magnes sive de arte magnetica (Cologne, 1643) by the Jesuit mathematician Athanasius Kircher (1602–1680).Footnote90 Steno expanded this mathematical interest gradually throughout his anatomical career. In his first publication, the Observationes anatomicae (Leiden, 1662), Steno famously announced his discovery of a new salivary duct, still known today as ductus stenonianus.Footnote91 But in this book, Steno also used methods and ideas from the mixed mathematical disciplines, such as measuring and comparing weights of glands and describing them as filters.Footnote92 Steno’s interest in mathematics culminated with the development of a geometrical theory of muscle contraction published years later in a book partly entitled Musculi descriptio geometrica (Florence, 1667) [Geometrical Description of Muscles].Footnote93 In this book’s preface, Steno argued that anatomy should become a mathematical discipline, just like astronomy, geography, and optics. In his words, ‘why can we not give to the muscles what astronomers give to the sky, what geographers give to the earth and … what writers on optics concede to the eyes?’Footnote94 Steno’s growing interest in physico-mathematics still needs further studies. But suffice to say that when Steno wrote about venous return in 1663, he was well-read in anatomy, experienced in dissections, and increasingly aware of the epistemic benefits of mixed mathematics.
In 1663, Steno was still a medical student at the University of Leiden. But just like in Pecquet’s Paris, Steno’s Leiden was a vibrant place for the development of new ideas where students could perform research by themselves.Footnote95 In Leiden, Steno learned from and collaborated with some of the most famous physicians of his time: professors like Franciscus Sylvius (1614–1672), practitioners like Wilhelm Piso (1611–1678), and colleagues like Jan Swammerdam (1637–1680). Moreover, Steno remained in regular contact with Thomas Bartholin (1616–1680), his former mentor at the University of Copenhagen and an influential voice in the medical Republic of Letters. The letters from Steno to Bartholin are the only extant source of Steno’s research on venous return.
A few months after publishing the Observationes anatomicae, in the summer of 1662, Steno began to study the motion of the heart. In this regard, Franciscus Sylvius told Steno that it was ‘not the heart, but the part of the [vena] cava closest to the heart [that was] the last to die’.Footnote96 Compelled by his professor’s remark, Steno undertook a series of vivisections to confirm this observation. He observed in a dying animal that the motion of the vena cava was, indeed, ‘most manifestly distinct from the motion of the heart’.Footnote97 Moreover, this motion ‘continued in the descending branch of the vena cava’, and, when the heart stopped beating, ‘the vena cava alone seemed to move’.Footnote98 Steno complemented these observations with a record of the hour in which the heart stopped beating and the number of pulsations that occurred in the vena cava after the heart failed – a testimony to Steno’s interest in quantification. Yet, Steno confessed that he did not know ‘the cause and manner’, i.e. why and how these motions occurred, so he asked Thomas Bartholin for an opinion.Footnote99 Bartholin’s reply however was not encouraging. He said that Johannes Walaeus (1604–1649) had already made similar observations.Footnote100 Indeed, Walaeus had been a colleague of Sylvius in 1640s Leiden and he had probably been the source for Sylvius’ comments to Steno.Footnote101 In addition, Bartholin wrote that there was nothing ‘admirable in the study’ of this motion of the vena cava, since ‘all the blood of the body enters the heart by means of this vessel’.Footnote102 Regardless, this motion remained in Steno’s mind.
A few months later, in the early winter of 1663, Jan Swammerdam dissected the lungs of an animal as part of his studies on respiration.Footnote103 His goal was to simulate the process of respiration by blowing air into the lungs with a bellow and the help of colleagues like Steno and Robert Padtbrugge. During this operation, Steno noticed something unusual, namely that the vena cava pushed the blood forward towards the heart ‘every time the animal has distended its thorax in inspiration’.Footnote104 This observation was unrelated to the work of Swammerdam, who was concerned with the eventual passage of air from the lungs into the heart. Nevertheless, the others told Steno that they had seen this motion before on other occasions. Moreover, according to Steno, the first to see this motion was their other colleague Johanne vonder Lahr. That Steno was concerned with ascertaining priority reflects his ongoing dispute regarding the discovery of the salivary duct. But it also shows that Steno considered this observation of the vena cava important.Footnote105
In the spring of 1663, Steno wrote again to Thomas Bartholin about this topic. This time, besides mentioning the new observation, he acknowledged that others had written on the subject. But he added that their explanations only worked ‘if it was licit to have recourse to the escaping of a vacuum [fuga vacui]’.Footnote106 According to them, Steno wrote, the dilation of the lungs ‘attracts’ the blood that is inside the vena cava.Footnote107 But ‘since escaping a vacuum, [a theory] nearly defeated in its entirety, seems to have run into a void’, Steno continued, it ‘seems truer’ to explain the motion of blood through ‘pulsing alone’.Footnote108 This claim resembles the typical mechanical rejection of attractions and invisible forces. But it also resonates with the physico-mathematical epistemology of Roberval that only described phenomena without explaining the underlying causes. Indeed, Steno does not state in this letter to Bartholin where he stands on the vacuum debate. Rather, he simply proposes that ‘the thorax, while dilated by the action of the muscles’, propels at the same time air into the lungs and venous blood into the heart. The reason, he adds, is that the dilated thorax ‘pushes everything in its surroundings to where resistance is less’.Footnote109
This observation and mechanism were important because, as Steno told Bartholin, they could shed light on the ‘uses of respiration in the circulation of blood’.Footnote110 The role of respiration on circulation was one of the questions left open by William Harvey and so it was one of the most desired prizes of post-Harveyan anatomy.Footnote111 This was one of the questions at the centre of Swammerdam’s research on respiration and Marcello Malpighi (1628–1694) had just written about it with his observations of the pulmonary alveoli with a microscope. Their main concern was what happened to the blood in vessels connected to the pulmonary artery and vein – a section of the circulation known as pulmonary circulation. Yet, unlike Swammerdam and Malpighi, Steno was not interested in the role of respiration in pulmonary circulation, but rather in the superior and inferior vena cava which received blood from all the body.Footnote112 No one before Steno had linked the motion of the lungs to the blood flow in the vena cava and supported it with an observation. Therefore, Bartholin was sceptical and abstained from making a judgement until there were ‘more observations’.Footnote113
Steno then replied that more people besides himself and Swammerdam had also seen this phenomenon, namely the young anatomists mentioned above and a certain Becker.Footnote114 But more importantly, in his reply, Steno improved his mechanism based on the physico-mathematical discipline of mechanics, which he metaphorically takes as a teacher.Footnote115 He wrote that inspired air pours into the lungs so that ‘the ribs, which move away from each other, and the diaphragm, moving outwards, exert a force on the air’.Footnote116 This motion of the ribs shows that they ‘yield to air by as much as the external surface compresses the air’.Footnote117 Indeed, he continued,
Mechanics teaches that a great force is necessary to condense it [i.e. the air], and experience shows that condensation never occurs unless all the surrounding bodies are strong enough to resist the compressed air. But if even a small part of the surroundings is unable to resist it, air propelled by a thrusting cause finds there a crack through which it escapes.Footnote118
Finally, in one of his first letters, Steno told Bartholin that the relevance of this mechanism could only be understood ‘if further experience confirms it’.Footnote122 The choice of the word ‘experience’ [experientia] and not ‘experiment’ [experimentum] may be important here. In the medieval and early modern periods, both terms meant a statement about how nature generally behaves.Footnote123 But in the late sixteenth century, scholars, and especially physicians, started to associate the term ‘experiment’ with contrived tests – more similar to the modern understanding of scientific experiments.Footnote124 Steno often spoke of ‘experiments’ when discussing chemical reactions or interventions to the vivisected body like ligatures in blood vessels.Footnote125 However, by speaking of experience, in this case, Steno seems to be asking for further exposure to a physiological phenomenon upon which he had no control.Footnote126 Indeed, Steno mentions other friends who had observed the same phenomenon on other occasions as a way to convince Bartholin that this was real. Yet, precisely because Steno only saw this phenomenon once, it is remarkable how rapidly he associated it with venous return and developed an entire mechanism for it. This confirms that Steno was familiar with Pecquet’s mechanism for the motion of chyle (which was the same), and with basic knowledge of hydrostatics, especially the elastic properties of air. It was as if Steno had already thought of this mechanism for venous return but lacked an observation to fully articulate it in writing. The observation consisted only of the joint motion of the thorax and vena cava. But for Steno, it was as if he experienced an entire mechanism.
Giovanni Borelli on venous return
And so comes Borelli, who wrote about the circulation in the second and less well-known part of De motu animalium. Unlike Pecquet and Steno, Borelli was not a physician but a mathematician, and most of his books until then had been on mathematics.Footnote127 Yet he decided to write a book about anatomy because, as he writes in the preface, ‘animals are bodies … and bodies are the subject of mathematics’.Footnote128 Thus, Borelli reveals in writing a greater philosophical commitment to submitting animals to the rules of mathematics than Pecquet and Steno, who only used insights from hydrostatics as complementary.
Just like others before him, Borelli was aware that Harvey had left several aspects of the circulation without an explanation, including venous return. Therefore, Borelli began with a proposition on the ‘mechanical reason’ by which blood flows from the arteries into the veins’.Footnote129 He argued that ‘blood makes its way into the capillary veins by means of an action similar to filtration’.Footnote130 For him, the connection point between arteries and veins functioned like a filter or a sponge in which blood moves due to the impulse of its own weight. Pecquet had also said that the blood that moves from the arteries to the veins is lighter or, as he says, has ‘less serosity’, because it loses some of its components.Footnote131 Borelli was thus building upon Pecquet’s explanation by adding a filter analogy.
But Borelli, like Pecquet, knew that this filtration was not enough to maintain the flow in larger veins. As smaller veins converge into larger ones, the ‘initial impulse is weakened so that blood needs help to be pushed further’.Footnote132 What then pushes the blood through the veins? In the final lines of this proposition, almost as if leading into the following one, Borelli provides a succinct list of ‘helping hands’, as he phrased it, that push the blood forward in the veins:
These are, in the first place, the peristaltic force, with which the same circular fibres of the veins are contracted … [This contraction is] also helped by the compression of the surrounding and internal inspired air, its weight and elastic force, and finally by the inflation of the muscles and several motions of the organs and fluids that run through the body.Footnote133
The valves in the veins had already been thoroughly studied by Fabricius d’Acquapendente (1533–1619), William Harvey’s professor at the University of Padua. Fabricius’ discovery of the valves in the veins was one of the triggers behind Harvey’s discovery of the circulation. In an entire proposition dedicated to the valves, Borelli expanded upon Fabricius and Harvey by proposing a geometrical disposition for the valves. He used it to explain the unidirectional blood flow towards the heart and to argue that valves contribute to propelling the blood forward in the veins (). He explained that when the veins contract, the blood is forced to move up and down, in both directions. But because of the closed valves, the blood that was pushed down bounces back up, ‘not different from a sports’ ball hitting a wall’, he writes.Footnote134 For this reason, ‘a double quantity of fluid is expelled at the same time through the same orifice’.Footnote135 Thus, ‘the mechanism [machina] of the valves [and] of the compression of the veins’ contribute to propel the blood towards the heart ‘by a quicker motion’.Footnote136 Ultimately, for Borelli, venous return is a consequence of the complex interaction between the compression of the veins’ walls and the geometrical disposition of the valves in the veins.
Figure 5. Borelli’s geometrical representation of the action of the valves in the veins. Here as represented in Borelli, De motu animalium (Leiden, 1685), Tabula decima sexta, Figura 1. Max Planck Institute for the History of Science, Berlin.
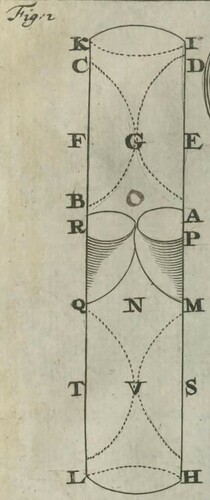
But where did Borelli stand with regards to the role of the air in the compression of the veins, first suggested by Pecquet in 1651 and explained by Steno in 1663? In De motu animalium, Borelli left a clue on what he thought was important to discuss about the circulation. He wanted to write ‘only [about] what has been omitted or not systematically explained by others concerning the causes, mechanical ways, and circumstances of this [circular] motion’.Footnote137 Therefore, since Borelli did not explore the action of air pressure on venous return, he did not consider it as much in need of an explanation as the action of the valves. In part, this was also because Borelli thought that inspired air played other, more-important physiological roles, which he investigated further. In a later chapter on respiration, Borelli explained that the inspired air had a tripartite role in the circulation: that of dividing the blood into its components, helping the particles of air mixing with blood particles, and contributing to the making of blood itself.Footnote138 But here Borelli was intervening in the already-mentioned debate on respiration and pulmonary circulation which involved several anatomists across Europe.Footnote139 Moreover, his discussion of particles of air betrays a greater commitment to corpuscularianism than Pecquet and Steno had. The problem of the action of air on venous return, however, was left untouched.
Most likely, Borelli was unaware of Steno’s explanation of venous return because Steno never published his explanation in his books. Instead, it remained hidden in two letters to Thomas Bartholin. Even if Borelli read Bartholin’s fourth volume of correspondence, published in Copenhagen in 1667, it was easy for a reader to miss Steno’s theory of venous return because Bartholin chose headlines for Steno’s letters that drew the reader’s attention away from it.Footnote140 The first letter’s title was a sample list of anatomical observations that did not include venous return.Footnote141 The second letter, where Steno developed the mechanical explanation of the elasticity of the air, was described as being about ‘the new fabric of the muscles and the heart’.Footnote142 Indeed, it was not until Albrecht von Haller (1708–1777) that the effect of respiration on venous return became a relevant physiological problem.Footnote143 Not surprisingly, Von Haller had mastered Steno’s anatomical works, including the letters to Bartholin. Tellingly, Von Haller’s description of Steno’s first letter to Bartholin in his Bibliotheca anatomica (Zurich, 1774) included a reference to ‘the evacuation of the vena cava in the abdominal by means of inspiration’.Footnote144
Conclusion
There is no doubt that in the second half of the seventeenth century, the circulation of the blood became a complex physiological process. Anatomists and mathematicians alike tried to solve some of Harvey’s open questions and, in the process, uncovered new physiological problems.Footnote145 This article showed how they tackled one of these problems with ideas and methods from physico-mathematics. In a recent review article, Sophie Roux proposed to study mechanical philosophies through controversies. A study by controversies shows ‘what mechanical philosophers expected from their own explanations’ and uncovers the varieties of mechanical angles.Footnote146 My analysis complements this approach by suggesting that looking at commonalities is also helpful. Pecquet, Steno, and Borelli did not differ much in their mechanism of venous return.Footnote147 But their small differences brought to the fore important aspects of the mechanization of anatomy. They revealed that observations played an implicit role in developing mechanisms and that mechanical analogies had deep links to the physico-mathematical disciplines.
Jean Pecquet was one of the first to suggest that the entry of air into the lungs contributed to venous return. He and Borelli also suggested that the motion of the surrounding organs, including the muscles, also contributed to venous return. But neither of them provided further details on this matter, even though they did so on other topics, such as the flow of chyle and the function of the valves. Their silence on this matter, however, speaks to the important role of observations in their research. The main difference between Pecquet and Borelli on one side and Nicolaus Steno on the other was Steno’s unique observation of the action of the diaphragm on the inferior vena cava. Based on this observation, Steno developed a mechanism of how the air’s elasticity, triggered by the motion of intercostal muscles, contracted the walls of the veins, which in turn pushed the blood into the heart. In short, the lack of observations stopped Pecquet from developing his explanation further, whereas an observation gave Steno room to develop a sophisticated mechanism. As for Borelli, unaware of Steno’s observation, he shifted his attention from the air to the valves. The fact that Steno’s mechanism relies on the motion of the diaphragm and intercostal muscles, which is one of the mechanisms proposed in modern physiology, further confirms the accuracy of Pecquet and Steno’s insight.Footnote148 Therefore, Richard Westfall was mistaken when writing that mechanical analogies ‘contributed almost nothing towards understanding what was seen’.Footnote149 In short, as historians of the mechanical philosophy had already suggested, mechanisms had important cognitive benefits for anatomists and mathematicians alike.
Pecquet and Steno’s mechanisms also have weak links to the mechanical philosophies of Descartes and others, in so far as corpuscles, the explicit rejection of Aristotelianism, and the reduction of the body to a machine are concerned. This does not mean that Pecquet and Steno were not atomists. But reading them on their own terms helps to discern that they were applying ideas from physico-mathematics to anatomy. Borelli’s mechanisms, on the other hand, especially the part of blood bouncing back on closed valves are more similar to his studies of particles in motion.Footnote150 Yet, mathematization remained Borelli’s main goal. Indeed, these actors’ recourse to physico-mathematical disciplines, although different among themselves, shows that their work was not as novel as it may seem. Their methods were similar to what Galen and Harvey had been doing. The difference is in the larger availability of objects and new ideas in the specific field of seventeenth-century mechanics.
This study of the physico-mathematics in the life sciences also invites historians to reconsider Eduard Dijksterhuis’ claim that ‘the mechanization of the world-picture … marks the beginning of the mathematization of science’.Footnote151 Indeed, throughout this article, I argued that the mechanization of venous return should be understood as mechanical only in so far as it drew from the physico-mathematical discipline of mechanics. Therefore, rather than distinct, mechanization and mathematization were the same in venous return and, if further studies confirm it, in other mechanisms in the life sciences.
Acknowledgments
I presented early versions of this article at the ‘Borelli, Reloaded’ workshop at Sapienza University, Rome, in September 2021 and at the colloquium of the Premodern Sciences Research Group at the Max Planck Institute for the History of Science, in Berlin in January 2022. I thank all those who offered comments and suggestions there. I am also grateful to Sophie Roux, Antonio Clericuzio, Evan Ragland, Peter Distelzweig, Katja Krause, and the two anonymous referees, who read and commented on early versions of this article.
Disclosure statement
No potential conflict of interest was reported by the author(s).
Additional information
Funding
Notes
1 On Harvey and the circulation see Robert Frank, Harvey and the Oxford Physiologists: Scientific Ideas and Social Interaction (Berkeley, CA: University of California Press, 1980); Roger French, William Harvey’s Natural Philosophy (Cambridge: Cambridge University Press, 1994); Marjorie Grene, ‘The Heart and the Blood: Descartes, Plemp and Harvey’, in Essays on the Philosophy and Science of René Descartes, ed. by Stephen Voss (Oxford: Oxford Univ. Press, 1992), pp. 324–36; Thomas Fuchs, The Mechanization of the Heart: Harvey and Descartes (Rochester, NY, 2001; original German edition, 1992); Anita Guerrini, ‘Experiments, Causation, and the Uses of Vivisection in the First Half of the Seventeenth Century’, Journal of the History of Biology, 46 (2013), 227–54; Evan Ragland, ‘Mechanism, the Senses, and Reason: Franciscus Sylvius and Leiden Debates Over Anatomical Knowledge After Harvey and Descartes’, in Early Modern Medicine and Natural Philosophy, ed. by Peter Distelzweig, Benjamin Goldberg, and Evan Ragland (New York: Springer, 2016), pp. 173–206.
2 There are a few exceptions, of course. In 1670s France, physicians still lectured against the circulation of the blood, see A. Guerrini, The Courtiers’ Anatomists: Animals and Humans in Louis XIV’s Paris (Chicago: University of Chicago Press, 2015), pp. 207–9. See also Thomas Bartholin, Epistolarum medicinalium centuria III (Copenhagen, 1667), pp. 308–11: ‘De sanguinis circulatione dissensus;’ and Mattia Mantovani, ‘Descartes’ Man Under Construction: The Circulatory Statue of Salomon Reisel, 1680’, Early Science and Medicine, 25 (2020), 101–34. For a short account of the circulation’s success see Gideon Mannig, ‘Circulation and the New Physiology’, in The Cambridge History of Philosophy of the Scientific Revolution, ed. by David M. Miller and Dana Jalobeanu (Cambridge: Cambridge Univ. Press, 2022), pp. 369–86.
3 There are entire research journals on circulation, such as those published by the American Heart Association, namely Circulation.
4 See John Hall and Michael Hall, Guyton and Hall Textbook of Medical Physiology, 14th ed. (Philadelphia, PA: Elsevier, 2021); and the list of contributors in Cardiovascular Biomechanics, ed. by Peter Hoskins, Patricia Lawford and Barry Doyle (Cham: Springer, 2017), p. ix.
5 This remains the case even while acknowledging that early modern anatomy encompassed questions that are today answered by physiology. On physiology as part of early modern anatomy, see Peter Distelzweig and Evan Ragland, ‘Medicine and the Science of the Living Body’, in The Cambridge History of Philosophy of the Scientific Revolution (2022), pp. 201–21 (pp. 202–4). For an early study of this topic, see Domenico Bertoloni Meli, ‘The Collaboration between Anatomists and Mathematicians in the mid-Seventeenth Century with a Study of Images as Experiments and Galileo’s Role in Steno’s Myology’, Early Science and Medicine, 13 (2008), 665–709.
6 On iatromechanics see the still-unsurpassable Theodore Brown, The Mechanical Philosophy and the ‘Animal Economy’ (New York: Arno Press, 1981) and Ugo Baldini, ‘Animal Motion Before Borelli, 1600–1680’, in Marcello Malpighi: Anatomist and Physician, ed. by Domenico Bertoloni Meli (Florence: Leo Olschki Editore, 1997), pp. 193–246. See also the recent overview Antonio Clericuzio and Maria Conforti, ‘Iatrochemistry and Iatromechanism in the Early Modern Era’, in Encyclopedia of Early Modern Philosophy and the Sciences, ed. by Dana Jalobeanu and Charles Wolfe (Cham: Springer Nature, 2021).
7 Venous return relates to multiple circulatory diseases and modern practices of cardiac resuscitation. See Joshua Beckman, ‘Diseases of the Veins’, Circulation, 106 (2002), 2170–72; Guyton and Hall Textbook of Medical Physiology, 249–50; and G. J. Noordergraaf and others, ‘The Donders Model of the Circulation in Normo- and Pathophysiology’, Cardiovascular Engineering, 6 (2006), 53–72 (p. 54).
8 On Borelli’s impact on modern biomechanics see Andreas Mayer, The Science of Walking: Investigations into Locomotion in the Long Nineteenth Century, trans. by Robin Blanton and Tilman Skowroneck (Chicago: The University of Chicago Press, 2020), p. 5.
9 Richard Westfall, The Construction of Modern Science: Mechanics and Mechanisms (Cambridge: Cambridge Univ. Press, 1971), pp. 86–104. More recent versions of this narrative can be found in Andrew Wear, ‘Early Modern Europe, 1500–1700’, in The Western Medical Tradition: 800 BC to AD 1800, ed. by Lawrence Conrad, Michael Neve, Vivian Nutton, and Roy Porter (Cambridge: Cambridge University Press, 1995), pp. 215–362 (pp. 333–57); and Roger French, Medicine Before Science: The Business of Medicine from the Middle Ages to the Enlightenment (Cambridge: Cambridge Univ. Press, 2003), pp. 182–84, 215–21.
10 Westfall, p. 94.
11 Westfall, p. 104.
12 Westfall, p. 41; On the epistemic weaknesses of the mechanical philosophies, see Daniel Garber and Sophie Roux, ‘Introduction’, in The Mechanization of Natural Philosophy, ed. by D. Garber and S. Roux (Dordrecht: Springer, 2013), pp. xi–xviii (p. xii); Margaret Osler, ‘How Mechanical was Mechanical Philosophy? Non-Epicurean Aspects of Gassendi’s Philosophy of Nature’, in Late Medieval and Early Modern Corpuscular Matter Theories, ed. by Christoph Lüthy, John Murdoch, and William Newman (Leiden: Brill, 2001), pp. 423–39 (pp. 438–39); Alan Gabbey, ‘Mechanical Philosophies and Their Explanations’, in idem, pp. 440–65 (p. 462); and A. Gabbey, ‘Henry More and the Limits of Mechanism’, in Henry More (1614–1687): Tercentenary Studies, ed. by Sarah Hutton (Dordrecht: Kluwer, 1990), pp. 19–35.
13 Westfall, p. 104.
14 Garber and Roux, pp. xi–xviii.
15 Helen Hattab, ‘The Mechanical Philosophy’, in Oxford Handbook of Philosophy in Early Modern Europe, ed. by Desmond Clarke and Catherine Wilson (Oxford: Oxford University Press, 2011), pp. 71–95 (p. 72); Domenico Bertoloni Meli, Mechanism: A Visual, Lexical and Conceptual History (Pittsburgh, PA: University of Pittsburgh Press, 2019), p. ix; Delphine Bellis, ‘The Later Sects: Cartesians, Gassendists, Leibnizians, and Newtonians’, in The Cambridge History of Philosophy of the Scientific Revolution, pp. 96–110; Sophie Roux, ‘What To Do With the Mechanical Philosophy?’ in The Cambridge History of Philosophy of the Scientific Revolution (2022), pp. 75–95 (p. 83).
16 Sophie Roux, ‘Quelles machines pour quels animaux? Jacques Rohault, Claude Perrault, Giovanni Alfonso Borelli’, in L'automate: Machine, métaphore, modèle, merveille, ed. by de Aurélia Gaillard, Jean-Yves Goffi, Bernard Roukhomovsky, and Sophie Roux (Bordeaux: Presses Universitaires de Bordeaux, 2012), pp. 69–113.
17 Roux, ‘Quelles machines pour quels animaux?’ pp. 76–79. On chemical reactions as mechanism see Antonio Clericuzio, ‘Chemical and Mechanical Theories of Digestion in Early Modern Medicine’, Studies in History and Philosophy of Biological and Biomedical Sciences, 43 (2012), 329–37; and Carmen Schmechel, ‘Descartes on Fermentation in Digestion: Iatromechanism, Analogy and Teleology’, The British Journal for the History of Science (2022), 1–16.
18 Dennis Des Chene, ‘Mechanisms of Life in the Seventeenth-Century: Borelli, Perrault, Régis’, Studies in History and Philosophy of Biological and Biomedical Sciences, 36 (2005), 245–60 (pp. 246–47).
19 On Descartes, see Dennis Des Chene, Spirits and Clocks: Machine and Organism in Descartes (Ithaca, NY: Cornell University Press, 2001); Gideon Manning, ‘Descartes’ Healthy Machines and the Human Exception’, in The Mechanization of Natural Philosophy, pp. 237–62; and Peter Distelzweig, ‘The Use of Usus and the Function of Functio: Teleology and its Limits in Descartes’ Physiology’, Journal of the History of Philosophy, 53.3 (2015), 377–400. On mechanization of the body more generally, see François Duchesneau, Les modèles du vivant de Descartes à Leibniz (Paris: Vrin, 1998); and Raphaële Andrault, La raison des corps: Mécanisme et sciences médicales (Paris: Vrin, 2016).
20 Bertoloni Meli, Mechanism, p. 109.
21 Bertoloni Meli, Mechanism, pp. ix, 109. In this way, Bertoloni Meli follows Roux’s detachment of mechanistic analogies from mechanical philosophies, but broadens the concept of mechanistic analogies beyond the three types of machines that Roux mentioned.
22 Bertoloni Meli, Mechanism, p. 140.
23 On the importance of actors’ terms when discussing mechanical philosophies, see Daniel Garber, ‘Remarks on the Pre-history of the Mechanical Philosophy’, in The Mechanization of Natural Philosophy, pp. 3–26.
24 Michael Boylan, ‘Mechanism and Teleology in Aristotle’s Biology’, Apeiron, 15.2 (1981), 96–102; Iain Lonie, ‘Hippocrates the Iatromechanist’, Medical History, 25 (1981), 113–50; Sylvia Berryman, ‘Galen and the Mechanical Philosophy’, Apeiron, 35 (2011), 235–53. See also Bertoloni Meli, Mechanism, pp. 11–16.
25 Treatises authored by Ctesibius (c. 285–222 b.C.), Vitruvius (c. 80–15 b.C.), and Hero of Alexandria (c. 10–70 AD). See Berryman, pp. 240–41, 245–46.
26 Peter Distelzweig, ‘“Mechanics” and Mechanism in William Harvey’s Anatomy: Varieties and Limits’, in Early Modern Medicine and Natural Philosophy, pp. 117–40.
27 Distelzweig, , p. 134. On the Mechanical Questions, which we know today not to have been authored by Aristotle, see Paul Rose and Stillman Drake, ‘The Pseudo-Aristotelian Questions of Mechanics in Renaissance Culture’, Studies in the Renaissance, 18 (1971), 65–104.
28 Matteo Valleriani, ‘The Transformation of Aristotle’s Mechanical Questions: A Bridge Between the Italian Renaissance Architects and Galileo’s First New Science’, Annals of Science, 66.2 (2009), 183–208; Helen Hattab, Descartes on Forms and Mechanisms (Cambridge: Cambridge University Press, 2009), pp. 12, 85–153. Hattab showed that Descartes’ metaphysical claims, and not just his interest in mathematics, derived from the Aristotelian Quæstions Mechanicae.
29 See Domenico Bertoloni Meli, Mechanism, Experiment, Disease: Marcello Malpighi and Seventeenth-Century Anatomy (Baltimore, MD: Johns Hopkins University Press, 2011).
30 This was the case even within natural philosophy, see Dmitri Levitin, ‘The Uses of Ancient Philosophy’, in The Cambridge History of Philosophy of the Scientific Revolution (2022), pp. 15–34; Charles Schmitt, Aristotle and the Renaissance (Cambridge, MA: Harvard University Press, 1983); Daniel Garber, ‘Physics and Foundations’, in The Cambridge History of Science, vol. 3: Early Modern Science, ed. by Katharine Park and Lorraine Daston (Cambridge: Cambridge Univ. Press, 2006), pp. 21–69; Domenico Bertoloni Meli, Thinking with Objects: The Transformation of Mechanics in the Seventeenth Century (Baltimore, MD: Johns Hopkins Univ. Press, 2006), pp. 135–40.
31 Ragland, ‘Mechanism, the Senses, and Reason’, p. 201.
32 Justin Smith, Divine Machines: Leibniz and the Sciences of Life (Princeton, NJ: Princeton Univ. Press, 2011), pp. 59–93 (pp. 65, 84). Ragland and Smith also mention chymistry as another source for mechanism.
33 See Peter Dear, Discipline and Experience: The Mathematical Way in the Scientific Revolution (Chicago: Chicago University Press, 1995); Peter Dear, ‘Mixed Mathematics’, in Wrestling with Nature: From Omens to Science, ed. by Peter Harrison, Ronald Numbers, and Michael Shank (Chicago: Chicago University Press, 2011), pp. 149–72; and Sophie Roux, ‘Forms of Mathematization (14th–17th Centuries)’, Early Science and Medicine, 15 (2010), 319–37. In this article I use the terms ‘physico-mathematics’ and ‘mixed mathematics’ interchangeably.
34 On mechanics see D. Bertoloni Meli, Thinking with Objects. On the impact on physiology, see D. Bertoloni Meli, ‘Machines of the Body in the Seventeenth Century’, in Early Modern Medicine and Natural Philosophy, pp. 91–116.
35 Dear, Discipline and Experience, pp. 32–62, 151–79; P. Dear, ‘The Meanings of Experience’, in The Cambridge History of Science, vol. 3: Early Modern Science, pp. 106–31 (pp. 119–26); and Luciano Boschiero, Experiment and Natural Philosophy in Seventeenth-Century Tuscany (Dordrecht: Springer, 2007), pp. 6, 27–35.
36 See Sophie Roux, ‘Was There a Cartesian Experimentalism in 1660s France?’ in Cartesian Empiricisms, ed. by Mihnea Dobre and Tammy Nyden (Dordrecht: Springer, 2013), pp. 47–88 and other chapters in the same book. See also Saul Fisher, Pierre Gassendi’s Philosophy and Science: Atomism for Empiricists (Leiden: Brill, 2005).
37 Antonio Clericuzio, Elements, Principles and Corpuscles: A Study of Atomism and Chemistry in the Seventeenth Century (Dordrecht: Kluwer Academic Publishers, 2000), p. 213. See also William Newman, Atoms and Alchemy: Chymistry and the Experimental Origins of the Scientific Revolution (Chicago: University of Chicago Press, 2006); Lawrence Principe, ‘Wilhelm Homberg: Chymical Corpuscularianism and Chrysopoeia in the Early Eighteenth Century’, in Late Medieval and Early Modern Corpuscular Matter Theories, pp. 535–56.
38 See Ragland, ‘Mechanism, the Senses, and Reason’, pp. 176–77; and Evan Ragland, ‘Between Certain Metaphysics and the Senses: Cataloguing and Evaluating Cartesian Empiricisms’, Journal of Early Modern Studies, 2 (2014), 119–39.
39 See Dear, Discipline and Experience, pp. 151–79, 210–15; Bertoloni Meli, Thinking with Objects, pp. 138–40; and Alan Chalmers, One Hundred Years of Pressure: Hydrostatics from Stevin to Newton (Cham: Springer, 2017), pp. 10–13, 60–62.
40 Sophie Roux, ‘What To Do With the Mechanical Philosophy?’, pp. 78–83; Chalmers, One Hundred Years of Pressure, pp. 111–33.
41 See Marcus Hellyer, Catholic Physics: Jesuit Natural Philosophy in Early Modern Germany (South Bend, IN: University of Notre Dame Press, 2005); Michael Gorman, The Scientific Counter-Revolution: The Jesuits and the Invention of Modern Science (London: Bloomsbury Academic, 2020).
42 French, William Harvey’s Natural Philosophy, pp. 75–93.
43 William Harvey, An Anatomical Disputation Concerning the Movement of the Heart and Blood in Living Creatures, trans. by Gweneth Whitteridge (Oxford: Blackwell Scientific Publications, 1976), pp. 71–72 (De motu cordis, chapter 7), and p. 123 (De motu cordis, chapter 17).
44 In humans and most other animals a superior and inferior vena cava converge into the heart’s atrium. Throughout this article the term vena cava is most often understood as inferior vena cava.
45 On Pecquet see Pierre Huard and Marie-José Imbault-Huart, ‘Pecquet, Jean’, in Complete Dictionary of Scientific Biography, vol. 10 (Detroit, MI: Charles Scribner’s Sons, 2008), pp. 476–78; Sarah Lewis, ‘Jean Pecquet (1622–1674) and the Thoracic Duct: The Controversy Over the Circulation of the Blood and Lymph in Seventeenth-century Europe’ (unpublished doctoral dissertation, Yale University, 2003); and Guerrini, The Courtiers’ Anatomists, pp. 46–49.
46 For an account of this discovery and its impact, see Bertoloni Meli, Mechanics, Experiment, Disease, pp. 31–55.
47 The English edition is Jean Pecquet, New Anatomical Experiments (London, 1653). The second Latin edition was published in Paris in 1654 with additions by Pecquet and letters addressed to him. All citations in this article are from the 1654 edition. Translations are the author’s.
48 Jean Pecquet, Experimenta nova anatomica (Paris, 1654), p. 25: ‘Dissertatio anatomica de circulatione sanguinis et chyli motu’.
49 Pecquet, Experimenta, p. 47 (chapter vii): ‘Circulari sanguinis motui sola systoles impulso non sufficit’.
50 Pecquet, Experimenta, p. 43: ‘Vel proprio ruit incitabulo sanguis, vel impellitur alieno. Proprium quidem ipsi [sanguine] nullum præter gravitatem agnoscimus, cuius virtutem subiectis rationibus libet evanidam demonstrare’.
51 Branko Furst, The Heart and Circulation: An Integrative Model, 2nd ed. (Cham: Springer, 2020), p. 324.
52 See French, William Harvey’s Natural Philosophy, pp. 260–61. For modern studies, see Roger Seymour and Kjell Johansen, ‘Blood Flow Uphill and Downhill: Does a Siphon Facilitate Circulation above the Heart?’ Comparative Biochemistry and Physiology Part A: Physiology, 88 (1987), 167–70; and Furst, pp. 324–29.
53 Pecquet, Experimenta, pp. 43–44.
54 Pecquet, Experimenta, p. 44. Borelli also addressed the problem of the blood’s interrupted flow, in Borelli, De motu animaliu pars secunda, p. 56: ‘Sanguis motus est continuus, licet in corde sit interruptus’.
55 Pecquet, Experimenta, pp. 44–45.
56 Hermann Conring, De sanguinis generatione et motu naturali (Leiden, 1646), p. 432: ‘Fit enim hic quodammodo idem atque in siphone inflexo’.
57 Conring cited the first theorem of Hero’s Pneumatics and the first book of Cardano’s De subtilitate (Nuremberg, 1550), both about siphons. Hero, unlike Aristotle, believed in an interstitial vacuum, but he denied the existence a continuous vacuum. See Hero, Spiritalium liber, trans. by Federico Commandino (Urbino, 1570), p. 10: ‘locus omnino vacuus esse non potest’. For more on early modern pneumatics see Matteo Valleriani, ‘Ancient Pneumatics Transformed during the Early Modern Period’, Nuncius, 29 (2014), 127–73.
58 Conring, pp. 432–33. See also Edward Grant, Much Ado About Nothing: Theories of Space and Vacuum from the Middle Ages to the Scientific Revolution (Cambridge: Cambridge University Press, 1981).
59 See William Shea, Designing Experiments and Games of Chance: The Unconventional Science of Blaise Pascal (Canton, MA: Science History Publications, 2003); Léon Auger, Un savant méconnu: Gilles Personne de Roberval (1602–1675). Son activité intellectuelle dans les domaines mathématique, physique, mécanique et philosophique (Paris: Librairie scientifique A. Blanchard, 1962); Robert McKeon, ‘Auzout, Adrien’, in Complete Dictionary of Scientific Biography, vol. 1 (Detroit, MI: Charles Scribner’s Sons, 2008), pp. 341–42.
60 Federica Favino, ‘Torricelli, Evangelista’, Dizionario Biografico degli Italiani, vol. 96 (2019) <https://www.treccani.it/enciclopedia/evangelista-torricelli_(Dizionario-Biografico)>.
61 These meetings were organized by the physician Pierre Bourdelot (1610–1685), in the tradition of Marin Mersenne’s (1588–1648) meetings. On Pecquet’s participation in this group, including Auzout’s assistance to Pecquet’s dissections, see Guerrini, The Courtiers’ Anatomists, pp. 44–47, 77, 273 (pp. 46–47), and René Taton, Les Origines de l’Académie Royale des Sciences (Paris: Palais de la découverte, 1966), pp. 16–17.
62 Dear, Discipline and Experience, pp. 193–96; Shea, Designing Experiments, pp. 88–91.
63 On the Puy-de-Dôme experiment see Shea, Designing Experiments, pp. 105–27.
64 William Shea refers to ‘Roberval’s dilatoriness’, in Designing Experiments, p. 88. Pecquet was the first to use the term in print, see Pecquet, Experimenta, p. 26.
65 Dear, Discipline and Experience, p. 202. For the year in which Pecquet wrote the work, see the book’s imprimatur (March 1651) and the dedicatory epistle (January 1651).
66 Shea, Designing Experiments, pp. 54–58.
67 Pecquet, Experimenta, p. 48: ‘Diastole solum obsequii vices conferente’.
68 On Pecquet’s early interests in hydrostatics, see Lewis, ‘Jean Pecquet’, pp. 46–50.
69 For Pecquet, fear of a vacuum was part of the broader ‘opinion of attraction’. Pecquet, Experimenta, pp. 62–66.
70 French, William Harvey’s Natural Philosophy, pp. 361–68.
71 French, William Harvey’s Natural Philosophy, p. 368.
72 Pecquet, Experimenta, p. 25: ‘Summa dissertationis: Sanguinis motus … demonstratur. Eiusdem motus causa indicatur. Attractionis opinio Hydraulicis exploditur machinis’.
73 Pecquet, Experimenta, p. 49 (chapter viii): ‘Esse non pondus tantum, sed et rarefactorium aeri elaterem, experimentis demonstratur’.
74 Pierre Guiffart, Discours sur le vide (Rouen, 1647). See also Shea, Designing Experiments, pp. 46–47 and Dear, Discipline and Experience, pp. 190–92. Guiffart would later comment extensively on Pecquet’s work, see Lewis ‘Jean Pecquet’, pp. 80–84.
75 Pecquet, Experimenta, p. 51: ‘Experimenta physico-mathematica de vacuo’.
76 Guerrini, The Courtiers’ Anatomists, pp. 46–47. See also Taton, Les Origines de l’Académie Royale des Sciences, pp. 16–17. On Pecquet’s novelty in publication see Bertoloni Meli, ‘The Collaboration between Anatomists and Mathematicians’, p. 672 (n. 8).
77 Pecquet, Experimenta, p. 73: ‘Caput X. Veræ sanguinei motus causæ aperiuntur’.
78 Pecquet, Experimenta, p. 73: ‘ut vasorum constrictionem, et eorumdem à vicinarum partium agitatione, vel etiam pondere, compressionem expendamus’.
79 Pecquet, Experimenta, p. 74: ‘vasorum tunica innatum excitat ad naturalis capacitatis modum Elaterem’.
80 Pecquet, Experimenta, pp. 73–75 (p. 74): ‘De violenta vasorum contractione. Hanc autem excitant, vel humoris acrioris asperitas … ’
81 Pecquet, Experimenta, p. 75: ‘Quod ad compressionem vasorum coarctatoriam spectat, eandem perfici dico et aspirati intra pulmonem aeris pressura, et adjacentium canalibus musculorum, dum agitantur, connixu, et ponderosarum qualibet ex causa partium gravamine’.
82 Borelli, De motu animalium pars secunda, p. 59: ‘peristaltica vi’.
83 Bertoloni Meli, ‘The Collaboration between Anatomists and Mathematicians’, pp. 671–72.
84 Pecquet, Experimenta, p. 82: ‘Sed ad respirationem redeo, in qua dum pulmones dilatantur etiam deorsum diaphragma premunt, jecurque … ’
85 Pecquet, Experimenta, p. 82: ‘quod tum pistillum agens succutientis per intervalla molis gravamine, non solum adigit chymum è ventriculo per pylorum in intestina secodere, sed et eorundem distendit porulos, illacque subtilissimam impellit inlacteas alimenti substantiam’.
86 Pecquet, Experimenta, p. 12: ‘Caput V: Ignotum hactenus chyli receptaculum inquiritur’.
87 Pecquet, Experimenta, p. 84: ‘sicut distendi nequeant, quin et ipusm pariter receptaculum distendentes, quem continet chylum adigant emittere’.
88 Pecquet, Experimenta, p. 84: ‘quem continet chylum adigant emittere’.
89 August Ziggelaar, ‘Commentary’, in Chaos: Niels Stensen’s Chaos-manuscript with Introduction, Notes and Commentary, ed. by A. Ziggelaar (Copenhagen: Danish Library of Science and Medicine, 1997), pp. 459–81 (pp. 473–74). On Steno’s interests in the mechanical philosophy see Antonio Clericuzio, ‘The Other Side of the Accademia del Cimento: Borelli’s Chemical Investigation’, in The Accademia del Cimento and its European Context, ed. by Marco Beretta, Antonio Clericuzio, and Lawrence Principe, (Sagamore Beach, MA: Science History Publications, 2009), pp. 17–30; A. Clericuzio, ‘Meccanismo ed empirismo nell’opera di Stensen’, in Scienza, Filosofia e Religione nell’Opera di Niels Steensen, ed. by María Vitoria and Francisco Gómez (Florence: Pagnini Editore, 2020), pp. 123–38.
90 Chaos, p. 122. Kircher held the mathematics chair at the Jesuit Colleggio Romano, see Gorman, The Scientific Counter-Revolution, pp. 206–7.
91 Henry Gray, Anatomy of the Human Body, 20th ed. (Philadelphia, Lea & Febiger, 1918), p. 1134.
92 Nuno Castel-Branco, ‘Dissecting with Numbers: Mathematics in Nicolaus Steno’s Early Anatomical Writings, 1661–64’, Substantia, 5 (2021), 29–42 (pp. 32–35).
93 Nicolaus Steno, Elementorum myologiæ specimen, seu musculi descriptio geometrica (Florence, 1667).
94 Steno, Elementorum myologiæ specimen, pp. iii–iv: ‘Et quidni musculis id daremus, quod cœlo astronomi, quod terræ geographi, et, ut ex microcosmo exemplum adducam, quod oculis rei opticæ scriptores concessere?’
95 For Steno’s circles in Leiden see Eric Jorink, ‘Modus politicus vivendi: Nicolaus Steno and the Dutch (Swammerdam, Spinoza and Other Friends), 1660–1664’, in Steno and the Philosophers, ed. by Raphaële Andrault and Mogens Laerke (Leiden: Brill, 2018), pp. 13–44.
96 N. Steno to Thomas Bartholin, 26 August 1662, in T. Bartholin, Epistolarum medicinalium centuria IV (Copenhagen, 1667), pp. 115–20 (p. 109): ‘non cor sed proximam cordi cavæ partem ultimo mori’.
97 Steno to Bartholin, 26 August 1662, in Bartholin, Epistolarum centuria IV, p. 109: ‘auriculæ patuit motus, et cavæ à motu cordis manifestissime distinctus’.
98 Steno to Bartholin, 26 August 1662, in Bartholin, Epistolarum centuria IV, pp. 109–10: ‘continuavit in ramo cavæ descendente;’ ‘sola cava … visa est moveri’.
99 Steno to Bartholin, 26 August 1662, in Bartholin, Epistolarum centuria IV, p. 112: ‘de causa motuum horum modoque necdum vel cogitare ausus sum’.
100 Bartholin to Steno, 7 September 1662, in Bartholin, Epistolarum centuria IV, pp. 113–17 (p. 115): ‘A vena cava motus initium esse, Walæus ante observaverit Epist. I etiam corde resecto’.
101 On Sylvius and Walæus, see Ragland, ‘Mechanism, the Senses, and Reason’.
102 Bartholin to Steno, 7 September 1662, in Bartholin, Epistolarum centuria IV, pp. 115–16: ‘Nec mirum hic motus initium et finem quærendum, si quidem universis corporis sanguis hac via cor ingreditur salvo animali, vel ingredi conatur, etiam illo deficiente’.
103 Steno to Bartholin, 5 March 1663, in Bartholin, Epistolarum centuria IV, pp. 348–359 (p. 351): ‘Sed hæc frequentia, rarum quod ingeniosissimus juvenis et in anatomicis exercitiis assiduus Dominus Swammerdamius mihi exhibuit, aerem, per medios pulmones in cor propellens’. Swammerdam’s research was published in Jan Swammerdam, Tractatus physico-anatomico-medicus de respiratione usuque pulmonum (Leiden, 1667).
104 Steno to Bartholin, 5 March 1663, in Bartholin, Epistolarum centuria IV, pp. 351–52: ‘Memoratu quoque dignum videtur, quod … quotiescunque inspirando thoracem distenderit animal evacuari et in collo et in abdomine cavam’.
105 For a brief account of the salivary duct dispute see Harald Moe, ‘When Steno Brought New Esteem to Glands’, in Nicolaus Steno 1638-1686: A Re-consideration by Danish Scientists, ed. by J. Poulsen and E. Snorrason (Gentofte, Denmark: Nordisk Insulinlaboratorium, 1986), pp. 51–96.
106 Steno to Bartholin, 5 March 1663, in Bartholin, Epistolarum centuria IV, p. 352: ‘si vacui fugam ad partes vocare liceret’
107 Steno to Bartholin, 5 March 1663, in Bartholin, Epistolarum centuria IV, p. 352: ‘thoracis dilatatione distentus pulmo aerem fugit quasi, et atrahit … dilatatam … cavæ portionem, attrahere illum sanguinem’
108 Steno to Bartholin, 5 March 1663, in Bartholin, Epistolarum centuria IV, p. 352: ‘Sed cum vacui fuga fere in totu devicta in vacuum aufugisse videatur, verior mihi illorum apparet mens, qui per solam pulsionem similia phænomena exponere solent’.
109 Steno to Bartholin, 5 March 1663, in Bartholin, Epistolarum centuria IV, p. 353: ‘thorax dum musculorum ope dilatatus circumstantia quælibet eo pellit, ubi minor est resistentia, uno eodemque tempore et in pulmones aerem, et inferiora versus abdominis viscera, et in partem cavæ intra thoracem, qui in cava extra thoracem est, sanguinem propellit’.
110 Steno to Thomas Bartholin, 5 March 1663, in Bartholin, Epistolarum centuria IV, p. 353: ‘pateret ulterior respirationis in circulatione sanguinis usus’.
111 Frank, Harvey and the Oxford Physiologists, pp. 16–20; and Leonard Wilson, ‘The Transformation of Ancient Concepts of Respiration in the Seventeenth Century’, Isis, 51 (1960), 161–72 (pp. 162–64).
112 On pulmonary circulation and respiration, see Frank, Harvey and the Oxford Physiologists, pp. 193–205; Wilson, ‘The Transformation of Ancient Concepts of Respiration’, p. 163; and Bertoloni Meli, Mechanism, Experiment, Disease, pp. 52–54.
113 Bartholin to Steno, 7 April 1663, in Bartholin, Epistolarum centuria IV, pp. 359–63 (p. 360): ‘Sed ad plures observationes suspendendum est judicium’.
114 Steno to Bartholin, 30 April 1663, in Bartholin, Epistolarum centuria IV, pp. 414–21 (pp. 418–19): ‘Cavæ motum inspirationi respondentem a Domino van der Lahr credideram inventum, sed inde tribus illam deberi inventionem didici, Padburgio, van der Lahr, et Beckero, qui simul sectioni tum temporis institutæ intererant’.
115 Steno to Bartholin, 30 April 1663, in Bartholin, Epistolarum centuria IV, p. 419: ‘mechanica docet’ (full quotation below).
116 Steno to Bartholin, 30 April 1663, in Bartholin, Epistolarum centuria IV, pp. 419–20: ‘diductæ à se mutuo costæ, descendensque diaphragma externo aeri vim inferunt’.
117 Steno to Bartholin, 30 April 1663, in Bartholin, Epistolarum centuria IV, p. 420: ‘quantum exterior superficies aerem premit, tantum ei cedant quædam in thorace contentæ partes’.
118 Steno to Bartholin, 30 April 1663, in Bartholin, Epistolarum centuria IV, p. 419: ‘Extra omnem namque controversiam esse puto, aerem cum à pellente quocunque vim patitur, vel densari, eodem, in quo est, loco, vel alium in locum propelli: ad condensandum autem magnam requiri vim mechanica docet, et experientia evincit condensationem nunquam succedere, nisi pressum aerem ambientia corpora omnia ad resistendum satis fuerint valida; quod si autem vel minima pars ambientis resistendo fuerit impar, illico à trudente causa propellius aer, qua elabatur, invenit rimam’.
119 Steno to Bartholin, 30 April 1663, in Bartholin, Epistolarum centuria IV, p. 420: ‘necessarium existimarem non densari aerem sed eo pelli, ubi minorem invenit resistentiam. Quæ autem cedunt, illa sunt, quæ thorace distento dilatantur, ut pulmones, et thoracem intra existens cavæ truncus’.
120 Steno to Bartholin, 30 April 1663, in Bartholin, Epistolarum centuria IV, p. 420: ‘sic et extra thoracem exsistens sanguis cedentem cavæ intra thoracem tunicam ut distendat, eò tanquam versus locum sibi non resistentem propellitur’.
121 See, for example, Marin Mersenne, Hydraulica, pneumatica, … et mechanica phænomena (Paris, 1644); Gaspar Schott, Mechanica Hydraulico-Pneumatica (Frankfurt, 1657); and especially Schott, Cursus Mathematicus (Frankfurt, 1661), pp. 424–64.
122 Steno to Bartholin, 5 March 1663, in Bartholin, Epistolarum centuria IV, p. 353: ‘si ulterior experientia idem confirmarit’.
123 Dear, Discipline and Experience, pp. 21–25; Dear, ‘The Meanings of Experience’, p. 106.
124 Evan Ragland, ‘“Making Trials” in Sixteenth- and Early Seventeenth-Century European Academic Medicine’, Isis, 108 (2017), 503–28 (p. 511); Elaine Leong and Alisha Rankin, ‘Testing Drugs and Trying Cures: Experiment and Medicine in Medieval and Early Modern Europe’, Bulletin of the History of Medicine, 91 (2017), 157–82.
125 See, for example, Steno, Observationes anatomicæ, pp. 43–44, 59. Further research should be done on Steno’s uses of experimentum and experientia.
126 Interestingly, Steno also based his comments on the condensation of the air on what ‘experience shows’. See Steno to Bartholin, 30 April 1663, in Bartholin, Epistolarum centuria IV, p. 419: ‘et experientia evincit’.
127 For more on Borelli see Thomas Settle, ‘Borelli, Giovanni Alfonso’, in Complete Dictionary of Scientific Biography, vol. 2 (Detroit, MI: Charles Scribner's Sons, 2008), pp. 306–14; and Luciano Boschiero, Experiment and Natural Philosophy in Seventeenth-Century Tuscany: The History of the Accademia del Cimento (Dordrecht: Springer, 2007), pp. 59–91.
128 Borelli, De motu animalium (Rome, 1680), vol. 1, Preface to Queen Christina: ‘Cum enim Animalia corpora sint … sintque corpora et motus subiectum Mathematicæ’.
129 Borelli, De motu animalius pars secunda, p. 57: ‘Ratio mechanica continuati cursus per venas exponitur’.
130 Borelli, De motu animalium pars secunda, p. 58: ‘et proinde actione simili filtrationi sanguis intra capillares venulas insinuetur’.
131 Pecquet, Experimenta nova anatomica, pp. 38–42. Pecquet alluded to the fact that blood lost some of its components at the intersection between arteries and veins.
132 Borelli, De motu animalium pars secunda, pp. 58–59: ‘Postea, quia, collectis pluribus venulis, latiorem ductum conficiunt, in eoque sensim motus sanguinis retardatur, et languet vis pristina impulsiva, ideo deinceps auxiliaribus manibus indiget, ut promoveri ulterius possit’.
133 Borelli, De motu animalium pars secunda, p. 59: ‘Et hæ quidem sunt primo loco vis, qua fibræ circulares venarum contrahuntur, peristaltica vi eisdem fibris insita, à qua vasa constringuntur, adiuvante etiam ambientis et interni aeris inspirati compressione, à pondere et elastica vi eius; nec non ab inflatione musculorum, et à motionibus variis viscerum et fluorum, per corpus animalis discurrentium, ut inferius dicemus’.
134 Borelli, De motu animalium pars secunda, p. 60: ‘non secus ac pila lusoria parieti illisa’.
135 Borelli, De motu animalium pars secunda, p. 60: ‘dupla fluidi moles eodem tempore per idem orificium emittitur’.
136 Borelli, De motu animalium pars secunda, p. 60: ‘Igitur per machinam valvularum compressionis venarum dupla velociori motu sanguinem versus cor protrudunt non fluxu continuo’.
137 Borelli, De motu animalium pars secunda, p. 56: ‘et ideo attingam solummodo ea, quæ ab aliis omissa, aut non rite exposita fuerunt, circa causas, modos mechanics et circumstantias huius motus’.
138 See Bertoloni Meli, Mechanism, Experiment, Disease, pp. 282–83.
139 Wilson, ‘The Transformation of Ancient Concepts of Respiration’, p. 163.
140 It is possible that Borelli did not read this specific volume. The Biblioteca Nazionale Centrale di Firenze, whose Magliabechi collection benefitted Borelli until 1667, and most Italian libraries do not have the fourth volume of Bartholin’s correspondence.
141 Bartholin, Epistolarum centuria IV, p. 348: ‘Epist. LV: De vesiculis in pulmone. Anatome cuniculi prægnatis. In pulmonibus experimenta. De lacteis mammarum. In cygno observationes, etc’. The ‘pulmonibus experimenta’ are Swammerdam’s observations as reported by Steno.
142 Bartholin, Epistolarum centuria IV, p. 414: ‘Epist. LXX: Nova musculorum et cordis fabrica’.
143 Lord Cohen of Birkenhead, ‘On the Motion of Blood in the Veins’, British Medical Journal, 3 (1971), pp. 551–57 (p. 553). Haller quotes Steno’s letter in Haller, Dissertationem inauguralem de motu sanguinis per cor (Gottingen, 1737), pp. 407–8. Haller’s work on venous return became widely known when published in English in Haller, ‘A Dissertation on the Motion of the Blood, and on the Effects of Bleeding’, in Monthly Review, or, Literary Journal (Aug 1757): 122–27.
144 Albrecht Von Haller, Bibliotheca anatomica, tomus I (Zurich, 1774), p. 492.
145 New anatomical questions involved the composition of blood, animal motion, and the functioning of glands.
146 Roux, ‘What To Do With the Mechanical Philosophy?’ p. 83.
147 Other scholars also wrote on peristaltic forces before the 1680s, such as Joseph-Guichard Duverney (1648–1730) and Claude Perrault (1613–1688), see Guerrini, The Courtiers’ Anatomists, pp. 165–200.
148 Furst, pp. 199–202: ‘17.1.2. Skeletal Muscle Pump Hypothesis;’ and Andrew Narracot, ‘The Venous System’, in Cardiovascular Biomechanics, pp. 127–42.
149 Westfall, p. 104.
150 G. Borelli, De vi percussionis (Bologna, 1667). See also Bertoloni Meli, Thinking with Objects, pp. 229–31.
151 Eduard Dijksterhuis, The Mechanization of the World Picture, trans. by C. Dikshoorn (Oxford: Oxford University Press, 1961), p. 501.