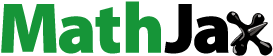
ABSTRACT
Objective
In this study, we investigated the advantages of attention allocation and spatial orientation among video game players (VGPs) and non-video game players (NVGPs) and explored the performance differences between the two groups in flight simulations.
Method
Thirty candidates from a Chinese university were categorised as VGPs and NVGPs before participating in all tests.
Results
The comparison of flight performance and eye movement indicators between the two groups showed that the flight performance of VGPs was significantly better than that of NVGPs. We then found that an attention shift task and group factors predicted flight performance during take-off, while spatial orientation and tracking tasks have direct and indirect effects on cruise task performance, respectively. Eye movement indicators can directly predict flight performance to a certain extent.
Conclusion
We believe that the transfer effect of game experience in simulated flight tasks assisted VGPs in using top-down processing strategies in the flight process, and in better allocating cognitive resources.
KEY POINTS
What is already known about this topic:
The advantages of attention allocation and spatial orientation among video game players (VGPs) and non-video game players (NVGPs). This difference not only caused by gaming experience, but also comes from the interaction of gaming experience and individual differences.
The VGPs perform better on simulated flight mission. We speculated that gaming practice had assisted VGPs in using top-down processing strategies in the flight process, and in better allocating cognitive resources.
VGPs did not depend solely on low-level cognitive abilities to finish tasks, but rather could rapidly understand the relevant situation and task in order to choose a proper attention allocation strategy, that visual search patterns must be adapted according to different situations, as opposed to reliance on a single parallel or sequence search.
What this topic adds:
The promotional effects of gaming experience occurred in high-level cognitive processing, but this effect was also observed in low-level ability tests. The key to promoting and maintaining excellent long-term performance are comprehensive cognitive skills, advanced cognitive skills, the establishment of mental models, and adaptive top-down processing strategies.
The aviation training system design should not stop at elemental training to improve general cognitive abilities, but should be a comprehensive ability-training game in order to improve training efficiency for pilots.
In order to establish a cognitive ability video game training system for pilots in the future, further exploration is needed to understand whether the transfer effect would occur in real world flight missions.
Introduction
Over the past decade, the potential cognitive benefits and training effects of playing video games have drawn tremendous attention from researchers (Feng et al., Citation2007; Green & Bavelier, Citation2003, Citation2006b, Citation2006c, Citation2007). Green and Bavelier (Citation2015) compared and analysed related studies of video game players (VGPs) and non-video game players (NVPGs), pointing out that gaming experience has brought about numerous improvements in cognitive abilities (Green & Bavelier, Citation2015). Owing to the action video game experience, basic perception skills in the ability to utilise selective attention and cognitive flexibility have been significantly enhanced.
This is partly because most video games require efficient allocation of attentional resources in the visual environment and the rapid and accurate identification of stimuli. These positive changes are observed not only in video game experts, but also in the development of action video game training (Green & Bavelier, Citation2015). Long-term gaming experience can improve the cognitive ability (Feng et al., Citation2007; Green & Bavelier, Citation2003, Citation2006a, Citation2006b, Citation2006c, Citation2007; Li et al., Citation2009; Spence et al., Citation2009). Other researchers have found transfer effects of video gaming experience in different fields of professional skills (Connolly et al., Citation2012; Franceschini et al., Citation2013, Citation2017; Girard et al., Citation2013; Lehtonen et al., Citation2017). An in-depth study of the effects of video gaming experience on cognitive abilities can be used as a theoretical basis for designing game-based training systems that may improve cognitive abilities required for different professional skills while being portable, low-cost, and efficient (Achtman et al., Citation2008).
Evidence of video game experiences promoting attention ability
VGPs must possess strong visual information processing and attention allocation capabilities, as well as task accuracy (Spence & Feng, Citation2010). Researchers have found that action video games might enhance gamers’ attention allocation and multitasking capabilities, improving performance in visual search tasks of varying difficulty (Hubert‐Wallander et al., Citation2011). Green et al. (Citation2010) established that VGPs performed better than NVGPs in classic attention allocation tasks, while Brooks et al. (Citation2015) found a similar result with sustained attention tasks. Dye et al. (Citation2009) found that VGPs’ attention management and distribution advantages persisted across different ages. Moreover, Feng et al. (Citation2007) showed that after 10 hours of action video game training, performance in attention and spatial tasks was significantly improved. These results imply that gaming can also improve visual performance in spatial tasks, which are traditionally the most difficult to train (Spence & Feng, Citation2010).
David (Citation2012) also proposed that the transfer effect observed in game training is influenced by the similarity between the training and test tasks; however, complex spatial capabilities, such as spatial visualisation, are more difficult to train. The results of this study are consistent with previous findings (Barlett et al., Citation2009; Terlecki et al., Citation2008). However, Sala et al.’s (Citation2018) meta-analysis showed that, in randomised control trials, video game playing had very weak effects of video games on cognitive abilities, but theories predicting no far-transfer effects are corroborated; however, they still suggested that video game training provides another possibility for cognition augmentation. Thus, VGPs can use this information better; that this improved cognitive ability induced better performance of cross-tasks (Dan et al., Citation2012).
Bediou’s et al.’s (Citation2018) analysis also shows that action video games enhance top-down attention and the field of spatial cognition, and researchers believe that more longitudinal intervention studies should be encouraged in this field. Thus, there is a possibility that the superiority of VGPs over NVGPs may be due to selection effects (e.g., more intelligent people are more likely to engage in video game playing) or to other factors such as motivation. Therefore, we chose a flight task (with some similarities to video game operations) and opted to measure attention and spatial orientation abilities, both critical to flight tasks.
Transfer effects of video games during missions
In addition to developing cognitive abilities, video gaming experiences can also be transferred to many professional fields. The results show that specific video game training promotes performance in related professional tasks. (Connolly et al., Citation2012; Franceschini et al., Citation2013, Citation2017; Girard et al., Citation2013; Lehtonen et al., Citation2017). Transfer effects occur when tasks require similar cognitive requirements (McKinley et al., Citation2011). Gopher et al. (Citation1992, Citation1994) determined that video simulation training can promote pilot performance in real flight missions. This lays a theoretical basis for the future development of a serious game training system aimed at improving pilots’ cognitive skills and performance. Cho et al. (Citation2012) directly compared the performance differences between video gamers and non-gamers on simulated flight missions. They observed that the performance of participants with extensive video gaming experience was significantly better in all simulated flight sections than that of participants who lacked video gaming experience. They attributed this performance difference to the improvement in attention allocation capabilities resulting from gaming experience. McKinley et al. (Citation2011) found similar results for an unmanned aerial vehicle (UAV) manipulation task. They compared the results of the pilot’s cognitive test with those of the VGP and NVGP groups. The results showed that although the pilots’ performance on several cognitive tests was significantly better than that of both VGPs and NVGPs, VGPs exhibited the strongest advantages in visual capture, recognition, and target tracking performance. In addition, VGPs and professional pilots had similar performance levels in UAV landing operations. This study suggests that the cognitive improvement obtained in the video game environment may be transferrable to UAV operating environments. VGPs may also have unique advantages in operating visual-related tasks because of their experience in rich visual environments.
Research hypothesis
At the onset of our study, we hypothesised that the transfer effect occurs when the action video game and professional task share the same cognitive processing ability requirements. For example, in the field of aviation, Carretta et al. (Citation1996) found that attention allocation and spatial orientation capabilities not only form the basis for mission execution, but also predict pilot performance. With 75% of the information involved in the flight process being visual in nature, visual attention capabilities are indispensable for successful piloting (Endsley & Bolstad, Citation1994). Good spatial orientation capabilities assist pilots in quickly determining their own position and understanding current information, facilitating situational awareness (SA) and flight safety. Thus, visual information processing capabilities are the most important cognitive characteristics of pilots (Kock & Schlechter, Citation2009).
Previous research has suggested that video gaming can promote attention and spatial capabilities. There has been a tentative exploration in the field of aviation research exploring the transfer effects from simulated flight missions. However, to establish a cognitive ability video game training system for pilots in the future, further exploration is needed to understand whether the transfer effect would occur in real-world flight missions. It is particularly important to understand the mechanism of the transfer effect to apply it to training system development. Therefore, we set up a simulated flight task, tested the attention and spatial orientation skills of the participants, and tracked participants’ eye movements throughout the task. This process aimed to determine whether the gaming transfer effect exists in this context by objectively analysing information processing and eye movements during flight. We also hypothesised that a relationship exists between transfer effect, attention allocation, attention switching, and spatial capabilities.
Materials and methods
Participants
This research complied with the China Psychological Association Code of Ethics and was approved by the Institutional Review Board of Shaanxi Normal University. Informed consent was obtained from all participants. We used Castel et al. (Citation2005) as a reference for the number of participants and grouping standard. Only male participants were selected because demographic data suggest that most pilots are male. The 30 participants were college students with normal vision. These participants were placed into one of two groups (n = 15 per group). The VGP group consisted of 15 males with an average gameplay time of 20 hours per week over a 3-year period. The mean age of participants was 22.1 years (SD = 1.79).
Participants reported playing video games that met the criteria established by Green and Bavelier (Citation2003); they required fast movement, tracking of multiple targets, and constant monitoring of environmental stimuli. The NVGPs included students who had little video game playing experience (average gameplay time of 2 hours per week within a 3-year period). The mean age of the NVGPs was 21.7 years (SD = 1.76). None of the participants from either group had participated in a similar experiment or any simulated flight game. The two groups of participants were separated from each other to avoid the influence of motivation on VGPs.
Materials
Simulated flight task
Three simulated flight scenarios were developed in simulated flight experiment systems in a key laboratory of behavioural and cognitive neurology located within Shaanxi Normal University. Participants were asked to fly three phases of simulated integrated flight tasks (fixed-wing aircraft) under visual flight rules (VFR) conditions on a sunny day without wind ().
Phase 1 consisted of a take-off and climbing phase. Participants were required to complete the take-off operation and fly to 5000 m, with flight performance recorded as completion time. The position and setting of each participant’s take-off were the same. Successful completion of this task was required for progression to Phase 2.
Phase 2 consisted of a search, target, and cruise phase. After taking off, participants were required to quickly search Target A, displayed in the out-view, and successfully fly to it. Targets A, B, and C appeared in order of difficulty, requiring participants to search for and successfully fly to each target. The completion time of each target’s search and flight task was recorded as flight performance.
Phase 3 was a landing task. The system presented a descent guide upon successful completion of Phase 2. Due to the difficulty of this landing task, all participants failed to successfully complete Phase 3. Therefore, only Phase 1 and 2 task performances were analysed in this study.
Attention allocation test
Inspired by the time-sharing test from Bolstad et al. (Citation2010), this paper analysed tasks of attention monitoring and attention tracking at the same time to examine participants’ attention allocation skills (
). The interface was evenly divided into two parts, the tracking task on the left and the monitoring task on the right.The tracking task required participants to track a ball (1 cm in diameter) moving in a random direction at 0.5 cm/s, using a computer mouse cursor, which was a round frame with a diameter of 2 cm. Tracking was considered successful when the moving ball had remained within the cursor frame throughout the task. As tracking time increased, ball speed increased (level 1–10), and the tracking success state increased by one level for every 10s. Ball speed increased by 0.1 cm/s at each difficulty level. Task performance was recorded as the highest difficulty level reached by a participant.
The monitoring task of the interface presented three watch dials and pointers, each of which rotated from a random mark. Participants were asked to press any key in order to reset the watch dial pointers when any of the three pointers moved to between 12 and 1, enabling the pointer to move on. Task performance for each dial was successful if any key was pressed while the pointer was between 12 and 1; it was unsuccessful otherwise. This test included 20 trials, each lasting 1 minute.
Attention shift test
We chose the Schulte table to test the participants’ attention skills (). It had been used to assess the efficiency and speed of visual search movements. We used a 6 × 6 number table in this test, asking participants to click on each number (from 1–36) in numerical order as soon as possible. Efficiency was measured by completion speed and using an eye movement index.
Spatial orientation test (Kozhevnikov & Hegarty, Citation2001)
In a total of 12 scenarios, participants were asked to indicate an azimuth to Target C, given a starting position and initial direction, by drawing another arrow from the starting position to the target object (e.g., “imagine you are standing near the car, facing the cat; point to the tree, shown in ). The participants were prevented from physically rotating their answer sheets. The absolute value of the difference between the participant’s answer and the correct angle was the participant’s score on a given question, and the mean deviation of all questions was the participant’s total score.
Apparatus
SMI eye tracking system
Eye movements were recorded at 120 Hz with SMI’s BeGaze software as fixations and saccades with a dispersion-based event detection algorithm. Fixations and saccades were determined using a displacement threshold of 0.1 deg. The dispersion was 100 px with a minimum fixation duration of 80°/s. Fixations slower than 100 m/s are commonly used to explore visual search patterns. We used a 5-point calibration to ensure that recordings had a mean spatial error of less than 0.8 deg. During the flight task, the participants sat in the simulation modules, 30 cm away from the screen. Small head movements were permitted, as long as participants remained continually fixated on the screen.
Procedure
First, the participants completed the spatial orientation test in paper, attention allocation and attention shift test in computer. Before completing the simulated flight mission, the researcher explained the requirements of the flight mission and the procedures to be performed. Each participant had no related experience with flight tasks. They were given 45 minutes to learn the operation of the simulated flight mission. At the beginning of the formal experiment, the participants completed the three flight missions designed by the system – take-off, cruise, and landing- in sequence and according to the requirements. The eye tracker monitored and recorded the participants’ real-time eye movements throughout the process.
Data analysis
According to the mission interface, the flight display interface is divided into three areas of interest: out view (OV) area, speed display (SD) area, and location display (LD) area. These three areas provide the visual information, speed information, and location information. During data processing, the participants’ eye movement information was compared according to the three areas of interest (AOI). In transportation-related research, the entropy stands for randomness of the eye movement scanning mode of participants and ranges between 0 and 1 (Harris et al., Citation1986). The formula was calculated, using R Studio, with reference to Peng et al. (Citation2014). MATLAB Neural Network Toolbox was used for neural network pattern recognition model. The data was divided into three parts, training set (70%), validation set (15%) and testing set (15%). We built a three-layer BP network model, including one input layer, one hidden layer, and one output layer. The number of hidden neurons was all set to the default value of 10. The training algorithm was Scaled Conjugate Gradient, the loss function used cross-entropy (CE), and the network performance was evaluated according to the confusion matrix and ROC curve.
Results
The difference of flight performance between VGPs and NVGPs
shows each participant’s performance. There is a significant difference between VGPs and NVGPs in terms of flight performance by t’-test. This difference was shown in the completion time of take-off (8.16 ± 0.89 vs 10.75 ± 1.17, t (28) = −6.84, p < 0.001, 95%CI (−3.37, −1.82), d = −2.59) and cruise tasks (38.69 ± 11.13 vs 66.19 ± 46.91, t (15.57)A = −2.21, p A < 0.05, 95%CI (−53.95, −1.06), d = −1.12; 63.26 ± 8.56 vs 99.86 ± 39.16, t(13.24)B = −3.29, p B < 0.01, 95%CI (−60.61, −12.58), d = −1.81; 52.14 ± 4.13 vs 69.31 ± 39.44, t(21)C = −1.50, p C > 0.05) between the two groups.
The difference of eye movement indicators between VGPs and NVGPs during the flight
shows the t’-test of the eye movement index. During the take-off task, the saccade count in the VGP group was less than that in the NVGP group (t (21) = −2.35, p < 0.05, 95%CI (−7.80, −0.48), d = −1.03), and the saccade time of VGPs was shorter than that of NVGPs (t (15.42) = −2.49, p < 0.05, 95%CI (−387.00, −30.37), d = −1.27). The VGPs had lower saccade amplitudes (t (11.83) = −2.40, p < 0.05, 95%CI (−32.70, −1.54), d = −1.40) and slower saccade velocities (t (21) = −2.17, p < 0.05, 95%CI (−840.81, −17.38), d = −0.95). There was no significant difference in saccade frequency between the two groups (t (22) = −0.98, p > 0.05).
Table 1. Descriptive statistics for the t-test for differences in eye movement during the flight between VGPs and NVGPs (M(SD))
In the cruising task, the NVGP group had higher saccade velocity (t (18) = −2.37, p < 0.05, 95%CI (−21085.99, −1255.10), d = −1.12), saccade frequency (t (21) = −2.40, p < 0.05, 95%CI (−0.51, −0.04), d = −1.05), saccade duration(t (15.20) = −2.14, p < 0.05, 95%CI (−10359.51, −31.47), d = −1.10), and saccade amplitude(t (16) = −2.44, p < 0.05, 95%CI (−707.32, −49.99), d = −1.22). No significant difference was observed in the saccade counts between the two groups (t (21) = −0.68, p > 0.05).
In the cruising task, the NVGP group had higher saccade velocity (t (18) = −2.37, p < 0.05, 95%CI (−21085.99, −1255.10), d = −1.12), higher saccade frequency (t (21) = −2.40, p < 0.05, 95%CI (−0.51, −0.04), d = −1.05), longer saccade duration (t (15.20) = −2.14, p < 0.05, 95%CI (−10359.51, −31.47), d = −1.10), and more saccade amplitude (t (16) = −2.44, p < 0.05, 95%CI (−707.32, −49.99), d = −1.22). No significant difference was observed in the saccade count between the two groups (t (21) = −0.68, p > 0.05).
Differences in attention allocation during the flight mission between VGPs and NVGPs
As shown in , the fixation count in the take-off task ( (a,b)) revealed significant relationships among the main effects of the groups (F (1,72) = 5.26, p < 0.05, η2 = 0.07). The main effect of AOIs was significant (F (2,72) = 71.86, p < 0.001, η2 = 0.67). The interaction effect between AOI and Group was significant (F (2,72) = 5.10, p < 0.01, η2 = 0.12). A further simple effect showed a difference between the two groups, mainly in the OV vs. LD and SD groups (p < 0.001).
Figure 6. (a) Fixation count in different area of interest (Take-off). (b) Fixation time in different area of interest (Take-off).
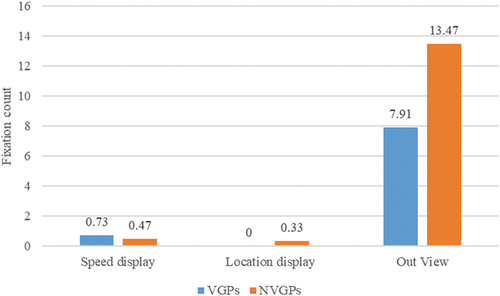
Figure 7. (a) Fixation count in different area of interest (Cruise). (b) Fixation time in different area of interest (Cruise).
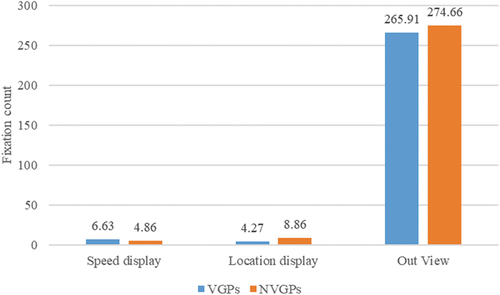
For fixation time, no significant relationship was found for the main effect of the groups (F (1, 72) = 2.67, p > 0.05). The interaction effect between AOI and Group was significant (F (2,72) = 3.14, p < 0.05, η2 = 0.80). A further simple effect showed a difference between the two groups, mainly in the OV vs. LD and SD groups (p < 0.001).
In the cruising task ( (a,b)), the main effects of fixation count (F (1,72) = 0.03, p > 0.05, η2 = 0.00) and fixation time (F (1,72) = 0.01, p > 0.05) between groups were insignificant.
The eye movement entropy of VGPs and NVGPs
According to the calculation method of entropy rate by Peng et al. (Citation2014). ,
(where D is the amount of AOI), E refers to entropy information,
(Pxi is the probability of fixation in each AOI), Txi refers to the average fixation time of the participants in a certain area, and i is the AOI number. Given the degrees of freedom, we used the Mann–Whitney U Test of entropy rate of the two groups of participants was conducted, and the results showed a significant difference in entropy rates between VGPs and NVGPs in the take-off and cruise tasks, as shown in .
Table 2. Descriptive statistics for the Mann-Whitney U test for differences in entropy between VGPs and NVGPs
Differences in spatial orientation and attention skills between VGPs and NVGPs
As shown in , the t’-test results showed that the attention shift of VGPs was faster than that of NVGP. As shown in , the t’-test results showed that the attention shift of VGPs was faster than that of NVGP (t (28) = −3.07, p < 0.01, 95%CI (−45.80, −9.13), d = −1.16). The VGP angular deviation of the spatial orientation test was significantly lower than that of the NVGPs (t (14.60) = −2.86, p < 0.05, 95%CI (−33.59, −4.83), d = −1.50). The Mann–Whitney U test results of attention allocation displayed higher difficulty levels for VGPs than NVGPs in the tracking task (W = 23.50, p < 0.001), but no significant differences in the monitoring task (W = 72.50, p > 0.05).
Table 3. Descriptive statistics for the spatial orientation and attention skills test between VGPs and NVGPs
Effects of spatial orientation and attention ability on flight performance
The regression analysis used group and attention shift as independent variables and flight performance (take-off, cruise A, B, C) as the attributive variables. As shown in , we conducted a hierarchical regression to determine which factors were predictive of flight performance. The regression results show that in the take-off task, the attention shift task explained a significant 43% of the variance in model 1, r = 0.65, r2 = 0.43, F (1,28) = 20.95, p < 0.01; and the group (VGPs or NVGPs) significantly increased the amount of variance accounted for by 29% in Model 2, r = 0.85, r2 = 0.72, F (2, 27) = 33.75, p < 0.01, r2 Change = 0.29, F Change (1, 27) = 27.05, p < 0.01.
Table 4. The influence of attention shift and group on flight performance
Using the Hayes Process v3.0 with the bootstrapping strategy (N = 5000), we only found the following mediation model in cruise B task (), that is, group was an independent variable, and spatial orientation and tracking task were mediator variables affecting flight performance in cruise (Target B). The direct effect of group (VGPs or NVGPs) on spatial orientation was significant (B = −12.23, SE = 5.35, t = −2.29, p < 0.05, 95%CI = (−23.29, −1.17)), and the group influenced cruise B performance indirectly through the direct effect of spatial orientation on cruise B performance (B = 1.23, SE = 0.30, t = 4.09, p < 0.01, 95%CI = (0.61, 1.85)). Meanwhile, the direct effect of group (VGPs or NVGPs) on the tracking task was significant (B = 2.49, SE = 0.56, t = 4.47, p < 0.01, 95%CI = (1.34, 3.64)), and the group influenced the cruise B performance indirectly through the direct effect of the tracking task on cruise B performance (B = −8.26, SE = 2.34, t = −3.52, p < 0.01, 95%CI = (−13.12, −3.40)). In this mediation model, spatial orientation had a full mediation effect on the relationship between group and cruise B performance, Indirect Effect = −15.04, Bootstrap SE = 8.82, 95% Bootstrap CI = (−35.38, −0.65); and the tracking task also played a full mediation effect between group and cruise B performance, Indirect Effect = −20.54, Bootstrap SE = 7.39, 95% Bootstrap CI = (−35.62, −6.52).
Prediction models of eye movement indicators on flight performance
(1) Neural pattern recognition model of eye movement indicators on flight performance in the take-off phase
First, we divided the flight performance in the take-off phrase into high flight ability group and low flight ability group by using K-means cluster analysis. After 4 iterations to achieve clustering convergence, the final grouping result was: 13 people in the low-level group, with a cluster centre of 8.32; 11 people in the high-level group, with a cluster centre of 11.20. Then we used the eye movement indicators in the take-off phase as the input index of the input layer, and the high and low groups of flight performance in the take-off phase as the output index of the output layer. The neural network pattern recognition results showed that the set accuracy requirements were achieved after 30 iterations, the training could be stopped in a short time, and the prediction accuracy of the testing set was 75% (
Table 5. The fitting index of neural network model
(2) Neural pattern recognition model of eye movement indicators on flight performance in the cruise phase
Firstly, we used the eye movement indicators in the cruise phase as the input index of the input layer, and whether the participant could complete the Target B task as the output index of the output layer. The neural network pattern recognition results showed that the set accuracy requirements were achieved after 4 iterations, the training could be stopped in a short time, and the prediction accuracy of the testing set was 100% (
Table 6. The fitting index of neural network model
Subsequently, we used the eye movement indicators in the cruise phase as the input index of the input layer, and whether the participant could complete the Target C task as the output index of the output layer. The neural network pattern recognition results showed that the set accuracy requirements were achieved after 14 iterations, the training could be stopped in a short time, and the prediction accuracy of the testing set was 100% (
Table 7. The fitting index of neural network model
Discussion
This study investigated the performance advantages of VGPs for simulated flight missions. The results verified that the spatial orientation, attention allocation, and attention shift skills of VGPs were more accurate and quicker than those of NVGPs, which was consistent with the results of existing research. Second, we used eye movement data to compare the attention processes between the two participant groups. We found that the simulated flight performance of VGPs exceeded that of NVGPs. We speculate that the reason for this finding is that VGPs would match the situation with an existing mental model in their long-term memory, according to their understanding of task targets and the environment. This process could promote information-processing efficiency and reduce attentional resources (McGloin et al., Citation2016). These conclusions are consistent with the hypothesis that prior experience can shorten visual information-processing time (Gegenfurtner et al., Citation2011). That is, they could judge and focus on effective target information according to experience, optimising the efficiency of attentional resources. This may be attributed to the experience accumulated by VGPs during the execution of similar tasks or to task transfer in action video games (McKinley et al., Citation2011).
We further explored the predictive factors for flight task performance. The results of hierarchical regression showed that gaming experiences and attention shift skills could predict take-off performance, and poorer attention shift skills predicted worse take-off performance. Also, the VGP group found it easier to achieve better flight performance. Additionally, although independent variables cannot predict cruise performance directly, we found a mediation effect between gaming experiences and cruise performance. Grouping could impact cruise B performance through the mediation effect of spatial orientation and attention allocation rank. Moreover, from the performance prediction results of eye movement data, attention behaviour can more accurately predict the level of task completion. This was consistent with our hypothesis and supported by the conclusion of Green and Bavelier (Citation2015). That is, the games may teach the meta-skill of attentional control by requiring VGPs to employ attention in a flexible manner across a wide variety of levels of resolution.
Analysis of a simulated flight task based on eye movement indicators
First, we found that VGPs showed a lower saccade count in two flight tasks with short saccade duration time and a low saccade amplitude, which is consistent with the research results of Azizi et al. (Citation2017). Researchers believe that prior experience can shorten the processing course of visual information (Gegenfurtner et al., Citation2011). When visual information in each AOI needs to be increased simultaneously, the saccade duration time should be shortened (Ball et al., Citation1988). Every stare of VGPs could obtain more information, resulting in a low saccade amplitude and saccade count. Therefore, they can accurately select and directly focus on the target information according to experience. In contrast to the VGPs in our study, NVGPs had quicker, and more frequent attention shifts with poorer flight performance. They were more likely to process visual information from bottom to top and to conduct parallel scanning of each visual stimulus to select useful information, thus increasing cognitive loads and leading to low efficiency of information processing.
Therefore, at least in the flight tasks of video games, VGPs have stronger attention selection and concentration skills because of their visual information-processing expertise accumulated during related tasks. A comparison of eye saccades between the two groups during flight simulation further supported our hypothesis. We analysed their attention allocation strategies by comparing the fixation time and fixation counts for each AOI. The flight simulation performance of the two groups supported the results of similar studies (Cho et al., Citation2012; McKinley et al., Citation2011).
Analysis of attention allocation and eye movement mode based on AOI information and entropy
During the take-off task, the VGPs spent less fixation time on each AOI, the greatest of which was the OV area. They finished information collection by glancing at the LD area only, with fewer fixation counts, meeting the eye movement requirements for pilots during flight (Niu et al., Citation2013).With no demand for heading control at the beginning of this task, VGPs adopted corresponding economical attention allocation strategies to complete tasks during cruising tasks, and there were significant differences in the attention allocation of VGPs at each AOI, indicating that they had adopted different allocation strategies. However, NVGPs maintained the same allocation strategy across AOIs.
The two groups showed no significant difference in cruising tasks; they mainly adjusted their attention allocation strategy according to their understanding of targets and environments and were seldom impacted by low-level visual features (Itti & Koch, Citation2000). The strategy conversion of VGPs between the two tasks can be explained in accordance with the visual search guided by targets (Ehinger et al., Citation2009; Kanan et al., Citation2009; Torralba et al., Citation2006). We directly compared the eye movement modes of the two groups using eye movement entropy. The results indicated that the eye movement mode of VGPs was highly strategic and of low randomness. Within unit time, they could obtain more key information, whereas the information collection efficiency of NVGPs was low. Again, this result supports the previous deduction that VGPs were relatively strategic in their use of attention shifting and allocation (Druker & Anderson, Citation2010).
The role of attention skills and spatial orientation
Upon conducting a direct comparison of attention skills and spatial orientation between the two groups, we determined that the spatial orientation of VGPs was more accurate than that of NVGPs, with better attention allocation and quicker shifting. This finding resonates with previous studies (Gardien, Citation2011; Vallett et al., Citation2013), and researchers speculated that the difference might be caused by higher cognitive abilities among VGPs. However, as far as the results of this study are concerned, we believe that this observed difference in cognitive abilities has a role in promoting game experience.
Analysis of the factors affecting the flight performance of the two groups
To summarise, the difference between VGPs and NVGPs in attention processing may be one cause for their differences in flight performance. However, according to attention processing analyses among VGPs and NVGPs, we consider that the transfer effect of gaming experience on flight simulation is not from the unidirectional process of some promoted low-level cognitive ability, based on the results of the regression equation. The influences of spatial orientation and attention skills on flight performance among VGPs and NVGPs were completely different. We further explored the predictive factors for flight task performance. First, attention shift skills and gaming experience could predict better flight performance. However, the predictability relationship of data may not be equal to the transfer effect of gaming experience on flight tasks. This may be because, in the take-off stage, they needed to pay attention to various types of visual information, allocating attention to the OV, LD, and SD areas to be able to complete the operation. In this process, they mainly depended on bottom-top processing, and information was obtained through sequential scanning. Moreover, they might have adopted the same attention allocation strategy without adjusting targets and scenes in accordance with different flight tasks. Hence, the completion times for take-off were restricted by the attention allocation capabilities. For the observed advantage of VGPs in this task, although this is completed by faster attention shift skills, the transfer effect of gaming experience could not be excluded. As mentioned in previous research, the mechanism of the inner transfer effect may be more likely to be a result of “learning to learn” where action games teach skills and knowledge that allow new tasks to be learned more quickly. Essentially, games may teach players the meta-skill of attentional control. Second, from the mediation effect of attention allocation rank and spatial orientation to cruise performance of these two groups, VGPs had more accurate spatial orientation judgment more easily and could predict higher cruise B performance. Additionally, VGPs could predict higher tracking task levels, but tracking task levels may predict cruise B performance in reverse. The final prediction result of the neural pattern recognition model verifies the predictive power of eye movement behaviour (attention mode) on task performance. Thus, it can be seen that, at least in this task, there were differences between the mechanisms of these two skills in cruising tasks, and between the interaction effect between these two skills with gaming experience. This supports our hypothesis that the performance of VGPs in flight tasks might be influenced by prior gaming experience, resulting from high-level cognitive control. This gaming experience transfer effect guided attention selection and strategies top-down. As a result, VGPs did not depend solely on low-level cognitive abilities to finish tasks, but rather could rapidly understand the relevant situation and task to choose an appropriate attention allocation strategy, following the cognitive economic principle (Wolfe, Citation2003): visual search patterns must be adapted according to different situations, as opposed to reliance on a single parallel or sequence search.
Conclusion
Our findings support our belief that the transfer effect occurred at the high-level control processing stage of VGPs which was a process of comprehensive promotion, which is consistent with the deduction of (Schmitt et al., Citation2015). Schmitt et al. (Citation2015) showed that the promotional effects of gaming experience occurred in high-level cognitive processing, but this effect was also observed in low-level ability tests. The key to promoting and maintaining excellent long-term performance are comprehensive cognitive skills, advanced cognitive skills, the establishment of mental models, and adaptive top-down processing strategies (Scalf et al., Citation2013). Based on this, we believe that aviation training system design should not stop at elemental training to improve general cognitive abilities, but should be a comprehensive ability-training game in order to improve training efficiency for pilots.
Data availability
The datasets analysed during the current study are not publicly available due to confidentiality agreement, but are available from the corresponding author on reasonable request.
Ethical approval
This research complied with the China Psychological 200 Association Code of Ethics and was approved by the Institutional Review Board of Shaanxi Normal University.
Informed consent
Informed consent was given to all participants in order to get their allowance for this study.
Disclosure statement
No potential conflict of interest was reported by the author(s).
Additional information
Funding
References
- Achtman, R. L., Green, C. S., & Bavelier, D. (2008). Video games as a tool to train visual skills. Restorative Neurology and Neuroscience, 26(4,5), 435–446. https://www.researchgate.net/publication/23461858_Video_games_as_a_tool_to_train_visual_skill
- Azizi, E., Abel, L. A., & Stainer, M. J. (2017). The influence of action video game playing on eye movement behaviour during visual search in abstract, in-game and natural scenes. Attention, Perception & Psychophysics, 79(2), 484–497. https://doi.org/10.3758/s13414-016-1256-7
- Ball, K. K., Beard, B. L., Roenker, D. L., Miller, R. L., & Griggs, D. S. (1988). Age and visual search: Expanding the useful field of view. JOSA A, 5(12), 2210–2219. https://doi.org/10.1364/JOSAA.5.002210
- Barlett, C. P., Anderson, C. A., & Swing, E. L. (2009). Video game effects—confirmed, suspected, and speculative: A review of the evidence. Simulation & Gaming, 40(3), 377–403. https://doi.org/10.1177/1046878108327539
- Bediou, B., Adams, D. M., Mayer, R. E., Tipton, E., Green, C. S., & Bavelier, D. (2018). Meta-analysis of action video game impact on perceptual, attentional, and cognitive skills. Psychological Bulletin, 144(1), 77–110. https://doi.org/10.1037/bul0000130
- Bolstad, C. A., Endsley, M. R., Costello, A. M., & Howell, C. D. (2010). Evaluation of computer-based situation awareness training for general aviation pilots. The International Journal of Aviation Psychology, 20(3), 269–294. https://doi.org/10.1080/10508414.2010.487013
- Brooks, J., Speelman, C. P., & Campitelli, G. (2015). Video game experience improves sustained and divided attention but does not affect resistance to cognitive fatigue (No. e1729). PeerJ PrePrints.
- Carretta, T. S., Perry, D. C., Jr, & Ree, M. J. (1996). Prediction of situational awareness in F-15 pilots. The International Journal of Aviation Psychology, 6(1), 21–41. https://doi.org/10.1207/s15327108ijap0601_2
- Castel, A. D., Pratt, J., & Drummond, E. (2005). The effects of action video game experience on the time course of inhibition of return and the efficiency of visual search. Acta Psychologica, 119(2), 217–230. https://doi.org/10.1016/j.actpsy.2005.02.004
- Cho, B. K., Aghazade, F., & Al-Qaisi, S. (2012). Effect of video-game experience and position of flight stick controller on simulated-flight performance. International Journal of Occupational Safety and Ergonomics, 18(3), 429–441. https://doi.org/10.1080/10803548.2012.11076942
- Connolly, T. M., Boyle, E. A., MacArthur, E., Hainey, T., & Boyle, J. M. (2012). A systematic literature review of empirical evidence on computer games and serious games. Computers & Education, 59(2), 661–686. https://doi.org/10.1016/j.compedu.2012.03.004
- Dan, C., Conger, M., Liao, J., Caldwell, J. L., & Vu, K. (2012). Improving multi-tasking ability through action videogames. Applied Ergonomics, 44(2). https://doi.org/10.1016/j.apergo.2012.08.002
- David, L. T. (2012). Training effects on mental rotation, spatial orientation and spatial visualisation depending on the initial level of spatial abilities. Procedia - Social and Behavioral Sciences, 33(33), 328–332. https://doi.org/10.1016/j.sbspro.2012.01.137
- Druker, M., & Anderson, B. (2010). Spatial probability aids visual stimulus discrimination. Frontiers in Human Neuroscience, 4, 63. https://doi.org/10.3389/fnhum.2010.00063
- Dye, M. W., Green, C. S., & Bavelier, D. (2009). The development of attention skills in action video game players. Neuropsychologia, 47(8–9), 1780–1789. https://doi.org/10.1016/j.neuropsychologia.2009.02.002
- Ehinger, K. A., Hidalgo-Sotelo, B., Torralba, A., & Oliva, A. (2009). Modelling search for people in 900 scenes: A combined source model of eye guidance. Visual Cognition, 17(6–7), 945–978. https://doi.org/10.1080/13506280902834720
- Endsley, M. R., & Bolstad, C. A. (1994). Individual differences in pilot situation awareness. The International Journal of Aviation Psychology, 4(3), 241–264. https://doi.org/10.1207/s15327108ijap0403_3
- Feng, J., Spence, I., & Pratt, J. (2007). Playing an action video game reduces gender differences in spatial cognition. Psychological Science, 18(10), 850–855. https://doi.org/10.1111/j.1467-9280.2007.01990.x
- Franceschini, S., Gori, S., Ruffino, M., Viola, S., Molteni, M., & Facoetti, A. (2013). Action video games make dyslexic children read better. Current Biology, 23(6), 462–466. https://doi.org/10.1016/j.cub.2013.01.044
- Franceschini, S., Trevisan, P., Ronconi, L., Bertoni, S., Colmar, S., Double, K., Facoetti, A., & Gori, S. (2017). Action video games improve reading abilities and visual-to-auditory attentional shifting in English-speaking children with dyslexia. Scientific Reports, 7(1), 5863. https://doi.org/10.1038/s41598-017-05826-8
- Gardien, R. (2011). Enhancement of attention through video game play: The effects of video game experience on attentional resources [Bachelor’s thesis].
- Gegenfurtner, A., Lehtinen, E., & Säljö, R. (2011). Expertise differences in the comprehension of visualizations: A meta-analysis of eye-tracking research in professional domains. Educational Psychology Review, 23(4), 523–552. https://doi.org/10.1007/s10648-011-9174-7
- Girard, C., Ecalle, J., & Magnan, A. (2013). Serious games as new educational tools: How effective are they? A meta‐analysis of recent studies. Journal of Computer Assisted Learning, 29(3), 207–219. https://doi.org/10.1111/j.1365-2729.2012.00489.x
- Gopher, D., Weil, M., & Bareket, T. (1992, October). The transfer of skill from a computer game trainer to actual flight. In Proceedings of the Human Factors and Ergonomics Society Annual Meeting (Vol. 36 (17), pp. 1285–1290). SAGE Publications.
- Gopher, D., Well, M., & Bareket, T. (1994). Transfer of skill from a computer game trainer to flight. Human Factors, 36(3), 387–405. https://doi.org/10.1177/001872089403600301
- Green, C. S., & Bavelier, D. (2006c). The cognitive neuroscience of video games. Digital Media: Transformations in Human Communication, 1(1), 211–223. http://citeseerx.ist.psu.edu/viewdoc/download;jsessionid=1CDAFF207C70D546E0246D736CCB9856?doi=10.1.1.118.6363&rep=rep1&type=pdf
- Green, C. S., & Bavelier, D. (2003). Action video game modifies visual selective attention. Nature, 423(6939), 534. https://doi.org/10.1038/nature01647
- Green, C. S., & Bavelier, D. (2006a). Effect of action video games on the spatial distribution of visuospatial attention. Journal of Experimental Psychology. Human Perception and Performance, 32(6), 1465. https://doi.org/10.1037/0096-1523.32.6.1465
- Green, C. S., & Bavelier, D. (2006b). Enumeration versus multiple object tracking: The case of action video game players. Cognition, 101(1), 217–245. https://doi.org/10.1016/j.cognition.2005.10.004
- Green, C. S., & Bavelier, D. (2007). Action-video-game experience alters the spatial resolution of vision. Psychological Science, 18(1), 88–94. https://doi.org/10.1111/j.1467-9280.2007.01853.x
- Green, C. S., & Bavelier, D. (2015). Action video game training for cognitive enhancement. Current Opinion in Behavioral Sciences, 4, 103–108. https://doi.org/10.1016/j.cobeha.2015.04.012
- Green, C. S., Li, R., & Bavelier, D. (2010). Perceptual learning during action video game playing. Topics in Cognitive Science, 2(2), 202–216. https://doi.org/10.1111/j.1756-8765.2009.01054.x
- Harris, R. L., Glover, B. L., & Spady, A. A. (1986). Analytical techniques of pilot scanning behavior and their application (NASA Technical Paper 2525).
- Hubert‐Wallander, B., Green, C. S., & Bavelier, D. (2011). Stretching the limits of visual attention: The case of action video games. Wiley Interdisciplinary Reviews. Cognitive Science, 2(2), 222–230. https://doi.org/10.1002/wcs.116
- Itti, L., & Koch, C. (2000). A saliency-based search mechanism for overt and covert shifts of visual attention. Vision Research, 40(10–12), 1489–1506. https://doi.org/10.1016/S0042-6989(99)00163-7
- Kanan, C., Tong, M. H., Zhang, L., & Cottrell, G. W. (2009). SUN: Top-down saliency using natural statistics. Visual Cognition, 17(6–7), 979–1003. https://doi.org/10.1080/13506280902771138
- Kock, F. D., & Schlechter, A. (2009). Fluid intelligence and spatial reasoning as predictors of pilot training performance in the South African Air Force (SAAF). SA Journal of Industrial Psychology, 35(1), 31–38. https://doi.org/10.4102/sajip.v35i1.753
- Kozhevnikov, M., & Hegarty, M. (2001). A dissociation between object manipulation spatial ability and spatial orientation ability. Memory & Cognition, 29(5), 745–756. https://doi.org/10.3758/BF03200477
- Lehtonen, E., Sahlberg, H., Rovamo, E., & Summala, H. (2017). Learning game for training child bicyclists’ situation awareness. Accident Analysis & Prevention, 105, 72–83. https://doi.org/10.1016/j.aap.2016.07.036
- Li, R., Polat, U., Makous, W., & Bavelier, D. (2009). Enhancing the contrast sensitivity function through action video game training. Nature Neuroscience, 12(5), 549. https://doi.org/10.1038/nn.2296
- McGloin, R., Farrar, K. M., Krcmar, M., Park, S., & Fishlock, J. (2016). Modeling outcomes of violent video game play: Applying mental models and model matching to explain the relationship between user differences, game characteristics, enjoyment, and aggressive intentions. Computers in Human Behavior, 62, 442–451. https://doi.org/10.1016/j.chb.2016.04.018
- McKinley, R. A., McIntire, L. K., & Funke, M. A. (2011). Operator selection for unmanned aerial systems: Comparing video game players and pilots. Aviation, Space, and Environmental Medicine, 82(6), 635–642. https://doi.org/10.3357/ASEM.2958.2011
- Niu, S. F., Lou, Z. S., & Lu, T. J. (2013). Analysis on eye movement indices based on simulated flight task of fighter pilots. Progress in Modern Biomedicine, 13(34). https://doi.org/10.13241/j.cnki.pmb.2013.34.003
- Peng, J. C., Gao, C. C., & Guo, Y. S. (2014). Drivers’ visual characteristics and metal load based one entropy rates. Journal of Chongqing Jiaotong University (Nature Science), 2(33), 118–121. https://doi.org/10.3969/j.issn.1674-0696.2014.02.26
- Sala, G., Aksayli, N. D., Tatlidil, K. S., Tatsumi, T., Gondo, Y., & Gobet, F. (2019). Near and far transfer in cognitive training: A second-order meta-analysis. Collabra: Psychology, 5(1), 18. https://doi.org/10.1525/collabra.203
- Scalf, P. E., Torralbo, A., Tapia, E., & Beck, D. M. (2013). Competition explains limited attention and perceptual resources: Implications for perceptual load and dilution theories. Frontiers in Psychology, 4, 243. https://doi.org/10.3389/fpsyg.2013.00243
- Schmitt, H., Kray, J., Schmitz, M., & Akbal, M. (2015, October). Training while playing?: Enhancing cognitive control through digital games. In Proceedings of the 2015 Annual Symposium on Computer-Human Interaction in Play (pp. 685–690). ACM.
- Spence, I., & Feng, J. (2010). Video games and spatial cognition. Review of General Psychology, 14(2), 92–104. https://doi.org/10.1037/a0019491
- Spence, I., Yu, J. J., Feng, J., & Marshman, J. (2009). Women match men when learning a spatial skill. Journal of Experimental Psychology. Learning, Memory, and Cognition, 35(4), 1097. https://doi.org/10.1037/a0015641
- Terlecki, M. S., Newcombe, N. S., & Little, M. (2008). Durable and generalized effects of spatial experience on mental rotation: Gender differences in growth patterns. Applied Cognitive Psychology: The Official Journal of the Society for Applied Research in Memory and Cognition, 22(7), 996–1013. https://doi.org/10.1002/acp.1420
- Torralba, A., Murphy, K. P., & Freeman, W. T. (2006). Shared features for multiclass object detection. In Toward Category-Level Object Recognition (pp. 345–361). Springer. https://doi.org/10.1007/11957959_18
- Vallett, D. B., Lamb, R. L., & Annetta, L. A. (2013). The gorilla in the room: The impacts of video-game play on visual attention. Computers in Human Behavior, 29(6), 2183–2187. https://doi.org/10.1016/j.chb.2013.05.001
- Wolfe, J. M. (2003). Moving towards solutions to some enduring controversies in visual search. Trends in Cognitive Sciences, 7(2), 70–76. https://doi.org/10.1016/s1364-6613(02)00024-4