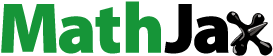
Abstract
In this paper, the adaptive robust fault-tolerant control problem for a class of switched nonlinear systems with parameter uncertainty, disturbances and actuator failures is concerned based on event-triggered control strategy. The adaptive laws based on state-dependent switched strategy are designed to eliminate the effects of actuator faults and parameter uncertainties by using the estimations of the unknown upper bounds of uncertain parameters. Then, the robust
fault-tolerant technique and multiple Lyapunov functions method are used, the designed controller can guarantee that all signals of the switched closed-loop systems are uniformly bounded. Meanwhile, the desired
performance of the systems is promised. Finally, the simulation results are given to illustrate the effectiveness of the proposed design method.
1. Introduction
As the complexity of industrial systems grows, faults are inevitable to occur. Actuator failure is the most common failure in the dynamic system, which will directly reduce the performance of the system and even destroy the stability of the system (1). In addition, the uncertainties and external disturbances also have an important impact on system performance (2–5). Due to the existence of these factors, there is an urgent need for high-reliability methods to ensure acceptable performance in case of failure or external disturbances. Therefore, it is necessary to continue exploring adaptive fault-tolerant control problems.
Adaptive fault-tolerant control is a highly reliable method to ensure system performance, which has made a great contribution to the development of industrial automation system (6–8). In recent years, literature on this issue has been widely concerned, especially in nonlinear control dynamic systems (9–12). Many successful adaptive schemes have been developed to achieve the expected control objective of the closed-loop system in the case of parameter changes and actuator failure. For instance, in Liu et al. (13) linear matrix inequality (LMI) technology was introduced to deal with the uncertainty of mismatch, and adaptive technology was used to compensate actuator fault. A nonlinear fault compensation function was constructed to solve the adaptive fault-tolerant control problem of uncertain switched nonaffine nonlinear systems with actuator faults and time delays in Wu and Park (14). With the in-depth study, fault-tolerant control methods are used in more complex systems, such as multi-agent systems (15), fractional order control systems (16) and linear quantum stochastic systems (17; 18), Takagi–Sugeno systems (19).
Because of its wide applications in practical systems, network control has recently received a large amount of attention (20; 21). Continuous signal input wastes resources and increases the burden on the system in the actual control system. Event-triggered control is used to reduce the need for feedback while ensuring the stability and expected performance level of the closed-loop system (22–24). Mishra et al. (25) presented an optimized algorithm for event-triggered control that keeps the system states on a stable trajectory. Kaneba et al. (26) used a hybrid event-triggered control scheme for the fault-tolerant control system with actuator failure and random parameter uncertainty.
As is known to all, switched system is a hybrid system with coordinated switched rules, which is composed of a group of continuous or discrete subsystems. Even if some subsystems are unstable, choosing appropriate switched rules can stabilize the whole system, which is a remarkable feature of its stability. It is this characteristic that makes the switched system extensive attention in the field of control. Chadli and Darouach (27) gave sufficient conditions for robust admissibility of uncertain switched singular systems in strict linear matrix inequality formulas. The switched rules of switched systems have attracted more attention, such as average dwell time strategy (28), minimum dwell time method (29), arbitrary switched (30) and so on. With the in-depth study of switched systems, based on these basic research methods, the event-triggered strategy was used to study discrete-time switched systems based on piecewise Lyapunov theory and average dwell time control, and the trajectory of the closed-loop system enters the bounded switched region (31). However, there are few research results using fault-tolerant control based on the event-triggered method in switched systems.
Inspired by the above considerations, this paper deals with the problem of adaptive robust fault-tolerant control for a class of uncertain switched nonlinear systems based on event-triggered control. Compared with the existing works, the main contributions of this paper can be summarized as follows:
Different from the existing results (23; 32), an adaptive robust fault-tolerant controller for a class of uncertain switched nonlinear systems is considered in this paper.
An adaptive
fault-tolerant control scheme based on event-triggered control is constructed to reduce the effects of disturbances and parameter uncertainty. At the same time, the adaptive law constructed in this paper can effectively deal with the actuator faults.
The control strategy proposed in this paper can ensure the boundedness of all signals of the switched closed-loop nonlinear systems and reduce the transmission count between the controller and the actuator.
The remainder of this paper is structured as follows. Some preliminaries and problem statement are introduced in Section 2. A robust adaptive switched fault-tolerant controller based on event-triggered control is designed in Section 3. The simulation results are shown in Section 4 to prove the effectiveness of the proposed method. Finally, the conclusions are drawn in Section 5.
2. Preliminaries and problem statement
2.1. System description
The following switched nonlinear system with unknown parameters and actuator failures is considered:
(1)
(1) where
is the state vector,
denotes the time-varying float fault,
is the control input with the switching signal
,
is the system external disturbance,
is the continuous nonlinear function satisfying
and
represents the output of the system. For
,
,
,
and
are known real constant matrices with appropriate dimensions. The switching signal
can be expressed as the following sequence:
when
,
means that the
th subsystem is activated.
In addition, we assume the parameter uncertainty satisfies the condition:
(2)
(2) where
with
being an unknown constant.
2.2. Basic assumptions and lemmas
Definition 2.1
Yang and Ye (33)
Consider the following system:
(3)
(3) where
,
and
are the parameter matrices with approximate dimensions, and
. Let
be a given constant, then the system is said to be with an adaptive
performance index no larger than
, if for any
, the following inequality holds:
(4)
(4)
Assumption 2.1
The time-varying fault function is piecewise continuous bounded, that is satisfies
with
being an unknown constant.
Assumption 2.2
Li et al. (34)
For the nonlinear term of the ith subsystem, there exists a function
such that
and
(5)
(5)
with
and
are unknown positive constants.
Remark 2.1
Assumption 2.1 is a standard assumption that is common in fault-tolerant control references. Assumption 2.2 can be understood that the adaptive laws are designed to compensate nonlinear disturbance effectively, under the condition of matching.
Lemma 2.1
Conte (35)
For any matrices A and B with appropriate dimensions, the following inequality holds:
(6)
(6)
Constructing a robust adaptive control scheme and corresponding rules to ensure the boundedness of all signals of closed-loop nonlinear switched systems is the main control objective of this paper, and the
performance index is not greater than
when there are parameter uncertainty, external interference and nonlinear function.
Remark 2.2
It should be noted that the method proposed by Zhang et al. (36) does not address the nonlinear fault-tolerant control problem of actuator failure. The switched system in this paper is more flexible and robust than the switched uncertain nonlinear systems in Cui and Xiang (37). Compared with Jin et al. (2) and Aouaouda and Chadli (27), the control method proposed in this paper considers a broader range of applications and effectively reduces system redundancy. The control scheme developed in this paper can ensure the boundedness of all signals and the desired performance switched closed-loop system while saving resources.
3. Event-triggered adaptive switched fault-tolerant control
In this section, to achieve the desired control objectives given in Section 2, the adaptive controller is designed as
(7)
(7) where
is a set gain matrix. In addition,
and
are the estimates of
and
, orderly, satisfying
and
,
and
are unknown constants, which are designed in (Equation12
(12)
(12) ) and (Equation13
(13)
(13) ).
is a positive uniform continuous and bounded function satisfying
(8)
(8) The triggering event is defined as
(9)
(9) where
is a positive design parameter,
,
,
,
is the controller update time, the time
will be updated as
when (Equation9
(9)
(9) ) is triggered, at the same time the controller
will be used in the systems (Equation1
(1)
(1) ). For
, if
, there exists a continuous time-varying parameter
, satisfying
,
and
, such that
(10)
(10) where
,
,
is an unknown constant. By Assumption 2.1, there are positive constants
and
satisfying
(11)
(11) And then, the adaptive update control laws are designed as
(12)
(12)
and
are positive designed parameters. Setting
, i = 1, 2, the following equation is established
(13)
(13)
4. Stability analysis
Theorem 4.1
On the basis of Assumptions 2.1 and 2.2, for the given positive design parameters ,
if there exist matrices
,
,
and positive constants
,
such that the following matrix inequality holds:
(14)
(14) where
. Then the adaptive
fault-tolerant controller (Equation7
(7)
(7) ) with adaptive laws (Equation11
(11)
(11) ) and switching law
(15)
(15) can guarantee that signals of the switched close-loop nonlinear system (Equation1
(1)
(1) ) are bounded and the
performance index is no larger than
.
Proof.
Substituting (Equation10(10)
(10) ) into (Equation1
(1)
(1) ), we can obtain the following system:
(16)
(16) Then choose a Lyapunov function candidate as follows:
(17)
(17) If the ith subsystem is active, we have
(18)
(18) With (Equation7
(7)
(7) ) we can get
(19)
(19) From (Equation6
(6)
(6) ), we can obtain the following conclusion
(20)
(20) According to (Equation20
(20)
(20) ) and Assumption 2.2, it can be gained that
(21)
(21) Combining (Equation1
(1)
(1) ) and (Equation14
(14)
(14) ), we have
(22)
(22) By invoking the Schur complement, we can draw the following conclusion
(23)
(23) Applying the switching law (Equation15
(15)
(15) ) and the inequality
, (Equation23
(23)
(23) ) becomes
(24)
(24) where
. If the external
, it can be inferred that the states of the switched closed-loop system are bounded. Arrange and integrate (Equation24
(24)
(24) )
(25)
(25) where
. Setting
where
and
. We can get that the
performance index of the switched closed-loop system (Equation1
(1)
(1) ) is less than or equal to
as in (Equation4
(4)
(4) ).
Remark 4.1
By multiplying both sides of (Equation14(14)
(14) ) by the inverse matrix
it is easy to get the following inequation
where
, with
, and
. The inverse matrix
, matrix
and matrix
can be obtained by solving the corresponding inequalities by using Schur complement theorem.
In addition, there exists a time satisfies
. According to (Equation7
(7)
(7) ) we can get
(26)
(26) Owing to x,
,
are continuous and bounded, which implies
and
are also continuous and bounded functions. Hence, there exists a constant
satisfying
. Considering
,
, and
, the following inequality holds
(27)
(27) we can obtain that there exists the inter-execution intervals
satisfies
, this means the Zeno behaviour is excluded. The proof is completed.
5. Simulation studies
In this section, two examples are used to prove the effectiveness of this method.
Example 5.1
Consider the following uncertain switched nonlinear system with unknown parameters and actuator failures:
where
and
In addition, it is assumed that
By selecting appropriate parameters and solving matrix inequality (Equation14(14)
(14) ), we can get that
In the simulation, the parameters of the system are selected as
,
, i, j = 1, 2,
,
,
,
, where i = 1, 2,
,
,
,
,
, and the
performance index is chosen as
. The simulation results are displayed in Figures . The switched signal is shown in Figure . From Figure , we can see that the adaptive switched fault-tolerant controller can ensure the state
of the system is bounded (
). The boundedness of parameter estimations
and the control signals
, i, j = 1, 2, k = 1, 2, 3 are displayed in Figures –.
Example 5.2
A simple mass-spring-damper switched system model from Long and Zhao (38) is used in this subsection, and the parameter matrices of the corresponding state space equation are ,
,
,
, i = 1, 2
In addition, set
, i = 1, 2.
,
,
,
,
,
,
the simulation parameters are chosen as
,
,
,
,
,
,
,
,
,
,
,
,
.
The simulation results can be seen from Figures . Figure demonstrates the switched signal. It can be easily found that the state , the parameter estimations
and the control signals of the switched system are all bounded in Figures –.
Remark 5.1
Based on event-triggered control strategy, a robust adaptive switched fault-tolerant controller with parameter updated laws is designed. Furthermore, compared with (23; 32) Figures – demonstrate that the proposed adaptive method can guarantee the system's expected performance while effectively reducing system redundancy in the event of failure.
6. Conclusion
For a class of uncertain nonlinear switched systems with parameter uncertainties, actuator failures, and external disturbances, this paper investigates the problem of robust adaptive event-triggered fault-tolerant control. The adaptive controller with the correlative parameter updated laws and state-dependent switching rule is designed to compensate for the actuator faults and eliminate the effects of nonlinear input disturbance by combining the linear matrix inequality and adaptive approach. It has been demonstrated that Zeno behaviour can be avoided and that all signals' boundedness and the expected
performances of switched closed-loop systems' can be guaranteed. Finally, two simulation examples are provided to show the viability of the proposed approach. In future work, for uncertain switched nonlinear systems with unknown control directions and full-state constraints, robust adaptive fault-tolerant tracking control design approaches will be taken into consideration.
Disclosure statement
No potential conflict of interest was reported by the author(s).
Data availability statements
Data sharing not applicable to this article as no datasets were generated or analyzed during the current study.
References
- Wei XJ, Wu ZJ, Reza KH. Disturbance observer-based disturbance attenuation control for a class of stochastic systems. Automatica. 2016;63:21–25. doi:10.1016/j.automatica.2015.10.019
- Jin XZ, Lu SY, Deng C, et al. Distributed adaptive security consensus control for a class of multi-agent systems under network decay and intermittent attacks. Inf Sci. 2021;547(8):88–102. doi:10.1016/j.ins.2020.08.013
- Mohamed K, Chadli M, Chaabane M. Unknown inputs observer for a class of nonlinear uncertain systems: an LMI approach. Int J Autom Comput. 2012;9(3):331–336. doi:10.1007/s11633-012-0652-2
- Zhang HG, Han J, Luo CM, et al. Fault-tolerant control of a nonlinear system based on generalized fuzzy hyperbolic model and adaptive disturbance observer. IEEE Trans Syst Man Cybern Syst. 2017;47(8):2289–2300. doi:10.1109/TSMC.2017.2652499
- Wu LB, Yang GH. Robust adaptive fault-tolerant control for a class of uncertain nonlinear systems with multiple time delays. J Process Control. 2016;41:1–13. doi:10.1016/j.jprocont.2016.02.001
- Qiu JB, Ding SX, Gao HJ, et al. Fuzzy-model-based reliable static output feedback H∞ control of nonlinear hyperbolic PDE systems. IEEE Trans Fuzzy Syst. 2016;24(2):388–400. doi:10.1109/TFUZZ.2015.2457934
- Jun M, Hamid RK, Xiang ZR. Observer-based adaptive consensus for a class of nonlinear multiagent systems. IEEE Trans Syst Man Cybern Syst. 2019;49(9):1893–1900. doi:10.1109/TSMC.6221021
- Pan HH, Li HY, Sun WC, et al. Adaptive fault-tolerant compensation control and its application to nonlinear suspension systems. IEEE Trans Syst Man Cybern Syst. 2020;50(5):1766–1776. doi:10.1109/TSMC.6221021
- Jing YH, Yang GH. Fuzzy adaptive fault-tolerant control for uncertain nonlinear systems with unknown dead-zone and unmodeled dynamics. IEEE Trans Fuzzy Syst. 2019;27(12):2265–2278. doi:10.1109/TFUZZ.91
- Wang F, Zhang XY. Adaptive finite time control of nonlinear systems under time-varying actuator failures. IEEE Trans Syst Man Cybern Syst. 2019;49(9):1845–1852. doi:10.1109/TSMC.6221021
- Liu WH, Li P. Event-triggered adaptive asymptotic tracking control of uncertain MIMO nonlinear systems with actuator faults. IEEE Trans Cybern. 2019;66(11):8681–8691. doi:10.1109/tcyb.2021.3061888.
- Jin X. Fault-tolerant nonrepetitive trajectory tracking for MIMO output constrained nonlinear systems using iterative learning control. IEEE Trans Cybern. 2019;49(8):3180–3190. doi:10.1109/TCYB.6221036
- Liu Y, Yang GH, Li XJ. Fault-tolerant control for uncertain linear systems via adaptive and LMI approaches. Int J Syst Sci. 2016;48(2):347–356. doi:10.1080/00207721.2016.1181225
- Wu LB, Park JH. Adaptive fault-tolerant control of uncertain switched nonaffine nonlinear systems with actuator faults and time delays. IEEE Trans Syst Man Cybern Syst. 2020;50(9):3470–3480. doi:10.1109/TSMC.6221021
- Yadegar M, Meskin N. Fault-tolerant control of nonlinear heterogeneous multi-agent systems. Automatica. 2021;127:109514. doi:10.1016/j.automatica.2021.109514
- Wang CY, Liu XP, Wang HQ. An adaptive fault-tolerant control scheme for a class of fractional-order systems with unknown input dead-zones. Int J Syst Sci. 2020;52(2):291–306. doi:10.1080/00207721.2020.1825874
- Wang S, Dong DY. Fault-tolerant control of linear quantum stochastic systems. IEEE Trans Autom Control. 2017;62(6):2929–2935. doi:10.1109/TAC.2016.2604303
- Liu YN, Dong DY, Petersen LR, et al. Fault-tolerant coherent H∞ control for linear quantum systems. IEEE Trans Autom Control. 2022;67(10):5087–5101. doi:10.1109/TAC.2021.3115843
- Aouaouda S, Chadli M. Robust fault tolerant controller design for Takagi–Sugeno systems under input saturation. Int J Syst Sci. 2019;50(6):1163–1178. doi:10.1080/00207721.2019.1597941
- Hespanha JP, Naghshtabrizi P, Xu YG. A survey of recent results in networked control systems. Proc IEEE. 2007;95(1):138–162. doi:10.1109/JPROC.2006.887288
- Qiu JB, Gao HJ, Ding SX. Recent advances on fuzzy-model-based nonlinear networked control systems: a survey. IEEE Trans Ind Electron. 2016;63(2):1207–1217. doi:10.1109/TIE.2015.2504351
- Heydari A. Optimal codesign of control input and triggering instants for networked control systems using adaptive dynamic programming. IEEE Trans Ind Electron. 2019;66(1):482–490. doi:10.1109/TIE.41
- Gao YF, Liu L. Lyapunov-based triggering mechanisms for event-triggered control. Int J Control Autom Syst. 2019;18(6):1392–1398. doi:10.1007/s12555-019-0340-x
- Yesmin A, Behera AK, Bera MK, et al. Dynamic event-triggering based design of sliding mode control. Int J Robust Nonlinear Control. 2021;31(12):5910–5925. doi:10.1002/rnc.v31.12
- Mishra SK, Jha AV, Verma VK, et al. An optimized triggering algorithm for event-triggered control of networked control systems. Mathematics. 2021;9(11):1262. doi:10.3390/math9111262
- Kaneba CM, Mu XW, Li X, et al. Event-triggered control for fault-tolerant control system with actuator failure and randomly occurring parameter uncertainty. Appl Math Comput. 2022;415:126714. doi:10.1016/j.amc.2021.126714.
- Chadli M, Darouach M. Robust admissibility of uncertain switched singular systems. Int J Control. 2011;84(10):1587–1600. doi:10.1080/00207179.2011.615865
- Li YF, Park JH, Hua CC, et al. Global output feedback tracking control for switched nonlinear systems with deferred prescribed performance. J Frankl Inst. 2021;358(3):1743–1764. doi:10.1016/j.jfranklin.2020.12.012
- Liu H, Zhou GP. Finite-time sampled-data control for switching T–S fuzzy systems. Neurocomputing. 2015;166:294–300. doi:10.1016/j.neucom.2015.04.008
- Lien CH, Yu KW, Chung LY, et al. H∞ performance for uncertain discrete switched systems with interval time-varying delay via switching signal design. Appl Math Model. 2013;37(4):2484–2494. doi:10.1016/j.apm.2012.06.001
- Su XJ, Liu XX, Shi P, et al. Sliding mode control of hybrid switched systems via an event-triggered mechanism. Automatica. 2018;90:294–303. doi:10.1016/j.automatica.2017.12.033
- Wu LB, He XQ, Zhang DQ, et al. Adaptive H∞ fault-tolerant control for a class of uncertain switched nonlinear systems with multiple state time delays. Int J Syst Sci. 2018;49(8):1784–1794. doi:10.1080/00207721.2018.1479004
- Yang GH, Ye D. Reliable H∞ Control of linear systems with adaptive mechanism. IEEE Trans Autom Control. 2010;55(1):242–247. doi:10.1109/TAC.2009.2036293
- Li ZK, Duan ZS, Lewis FL. Distributed robust consensus control of multi-agent systems with heterogeneous matching uncertainties. Automatica. 2014;50(3):883–889. doi:10.1016/j.automatica.2013.12.008
- Conte G. A course in H∞ control theory [Book reviews]. IEEE Trans Autom Control. 1987;32(12):1144–1145. doi:10.1109/TAC.1987.1104520
- Zhang J, Li S, Ahn CK, et al. Decentralized event-triggered adaptive fuzzy control for nonlinear switched large-scale systems with input delay via command-filtered backstepping. IEEE Trans Fuzzy Syst. 2022;30(6):2118–2123. doi:10.1109/TFUZZ.2021.3066297
- Cui D, Xiang Z. Nonsingular fixed-time fault-tolerant fuzzy control for switched uncertain nonlinear systems. IEEE Trans Fuzzy Syst. 2022;31(1):1–10. doi:10.1109/tfuzz.2022.3184048.
- Long LJ, Zhao J. Adaptive output-feedback neural control of switched uncertain nonlinear systems with average dwell time. IEEE Trans Neural Netw Learn Syst. 2015;26(7):1350–1362. doi:10.1109/TNNLS.2014.2341242