Abstract
Capsule Wing length and age independently predicted spring arrival timing in a long-distance migratory warbler, but there was no association with mass or tarsus length.
Aims To test a range of biometric features and other measures of fitness for their importance in determining spring arrival patterns in a long-distance migratory landbird, and to investigate why these features may be important.
Methods Fifty-one males with known spring arrival dates were caught and key biometrics were measured at breeding sites across Norfolk, UK, during April and May 2012. Blood samples were also taken in order to consider any effect of infection with blood parasites.
Results Early-arriving males were more likely to be adults and to have longer wings. Mass and tarsus length had no significant relationship with arrival timing. Haemosporidian prevalence was low (∼10%) compared to other studies of this species, and so it was not possible to determine the effect of infection on arrival date with confidence.
Conclusions Wing length, rather than other measures that simply measure structural body size, is probably important in arrival timing due to either the advantage it confers by increasing flight efficiency or because it reflects past condition during feather growth.
Early spring arrival on breeding grounds has demonstrated reproductive benefits for migratory birds (Bensch & Hasslequist Citation1991, Smith & Moore Citation2005, Norris et al. Citation2004, Gunnarsson et al. Citation2005). However, migration is energetically costly (Sillett & Holmes Citation2001), and it is therefore expected that experienced and fitter birds are more able to endure these costs and arrive before the average individual in the population. Although there is evidence that early-arriving males may be older (Francis & Cooke Citation1986, Stewart et al. Citation2002), of larger body size (Francis & Cooke Citation1986), or in better body condition (Marra et al. Citation1998) than later-arriving males, there still exists some uncertainty as to which fitness measures, if any, are the most important in determining arrival timing. High levels of correlation between many morphological features associated with fitness make distinguishing between the effect of structural size and condition, and their relationships with age difficult. Many studies instead use condition indices derived from various morphological features to calculate a summarized measure of condition while controlling for body size (Reist Citation1985, Krebs & Singleton Citation1993); however these not only risk producing spurious results (Green Citation2001), but also lose much of the detail of the original measures.
This study aimed to investigate the role of age and commonly measured biometrics in determining individual spring arrival timing at breeding territory for a long-distance migratory warbler, and to explore why these features may be important. It is also aimed to test whether using condition indices instead of mass as a measure of fitness improved modelling arrival patterns, as these have been found to be associated with arrival timing in some studies (Marra et al. Citation1998). As blood parasites have been found to play an important role in host population dynamics and fitness (Ricklefs Citation1992, Dyrcz et al. Citation2005, Marzal et al. Citation2005) and possibly spring arrival (Rätti et al. Citation1993, Møller et al. Citation2004), infection status was also included in this study as a measure of fitness.
Although studies of the spring arrival patterns of passerines are fairly numerous, the data are often collected at coastal migration ringing stations, where large sample sizes can be obtained. Such studies are useful for large scale analyses, but are limited by the mixing of separate breeding populations and the fact that the final breeding destination is unknown. Additionally, tarsus length, an important measure of structural body size (Freeman & Jackson Citation1990, Senar & Pascual Citation1997), is generally not measured, and the collection of biometrics by numerous people may result in unstandardized data. Studies focusing on arrival at the eventual breeding site have also often been limited to certain well-studied species, such as the Barn Swallow Hirundo rustica (Møller et al. Citation2004, Saino et al. Citation2004), the Pied Flyatcher Ficedula hypoleuca (Potti Citation1998, Both & Visser Citation2001) and the American Redstart Setophaga ruticilla (Lozano et al. Citation1996, Marra et al. Citation1998). This study, therefore, attempts not only to test current theory on arrival timing using an unstudied species, but also to use good quality biometric data, standardized through the collection by one person, to investigate the relationships between these measures.
Based on the studies cited above, it is expected that early-arriving males will be older, longer winged, structurally larger (i.e. with longer tarsi) and heavy for their size. It is also predicted that infected individuals will arrive later than healthy ones. The study species, the Common Whitethroat, Sylvia communis (hereafter Whitethroat) is a medium-sized warbler (∼12–17 g) which winters in tropical Africa and breeds across Europe and temperate Asia (Cramp & Brook Citation1992).
METHODS
Study site
Field work was carried out at five locations in Norfolk. Three sites around Norwich: Whitlingham Marsh (52°37′05″N, 1°22′19″E; 25 ha), a private nature reserve dominated by Phragmites reed beds interspersed with bramble patches (Rubus spp.) and deciduous woodland. Bowthorpe Common (52°38′04″N, 1°13′28″E; 35 ha), a grassy public common edged with bramble, mixed hedgerows and woodland, and a third site encompassing the River Yare riparian zone running through the campus of University of East Anglia (52°37′14″N, 1°13′59″E; 30 ha). The remaining two sites were near Thetford: Mayday Farm (52°25′02″N, 0°37′23″E; 24 ha) and Croxton Heath (52°27′21″N, 0°46′09″E; 7 ha), both of which consisted of stump rows (upended trees from forest clearance) within open areas of bracken and scrub surrounded by managed pine plantation stands (Pinus nigra and P. sylvestris).
Measuring individual arrival dates
Field work was conducted between the 7 April and 28 May 2012. Each site was monitored every two to three days between dawn and dusk to locate newly arrived Whitethroats in order to determine individual arrival dates. Monitoring was avoided during periods of bad weather, as males were likely to be less detectable at these times (Slater Citation1994). However, during periods of persistently bad weather, where monitoring was delayed by a day, sites were also monitored during light rain. Although heavy rain affected Whitethroat behaviour, males still sang and responded to conspecific playback during light rain (pers. obs.), and therefore it was judged unlikely to have affected detection. Days of consistent rain were rare, however, and so monitoring was possible almost every day.
At each site, a set route which covered all suitable Whitethroat breeding habitat (described in Cramp & Brook Citation1992) was walked slowly (∼1 mph), pausing regularly. A short burst of Whitethroat song mixed with female calls was played using loudspeakers every 2 to 3 min to induce a response from any nearby males. Male Whitethroats are highly detectable from when they first arrive on the breeding grounds, because they sing regularly throughout the day and perform song flights when a female is nearby (Cramp & Brook Citation1992), and males will readily sing and perform song flights in response to playback (pers. obs.). Monitoring ceased after no new males were detected over a four-day period in late May.
Arrival date was estimated as the middle point between the date the site was last monitored and the date that the male was first detected. Estimated arrival dates are presented as the number of days after the arrival of the first detected Whitethroat on 16 April 2012 (day zero). Males for which the estimated arrival date was unknown or uncertain (n = 8) were excluded from analyses on arrival timing; however, they were included in analyses exploring morphological relationships and infection prevalence. This occurred if the bird was located at the edge of monitoring areas, or if located in a small area where there was high Whitethroat density and no clearly discernible territory boundaries. In these cases, if a bird was not caught very soon after arrival then confusion could arise when other males arrived at the same location and they could not be distinguished.
Catching and marking individuals
Once detected, males were caught with playback of conspecific song and female calls using mist nets, ringed with an aluminium ring and colour ringed with an individual colour combination so that they could be identified over the future monitoring period. Males were caught within four days of detection if possible. If capture took place after this time period due to previous failed attempts, individuals were only included in arrival analysis if a male had been consistently detected singing in that territory. Any error resulting from this delay was minimized by repeating all analyses including only birds captured within four days of detection.
Individual measurements
Flattened wing length (to the nearest 0.5 mm) was measured from the carpal joint to the top of the longest primary feather, tarsus length (to 0.1 mm) was measured from the top of the flattened foot to the inter-tarsal notch using digital callipers, and body mass (to 0.1 g) was measured using digital scales. These methods are described in more detail in the BTO Ringers' Manual (Redfern & Clark Citation2001). All biometrics were taken by one observer (A.R.). Whitethroats were aged as either second years (hatched the previous year; EURING code 5) or adults (hatched before the previous year; EURING code 6), using a combination of features described by Svensson (Citation1992) and Jenni & Winkler (Citation1994), such as colour and wear, shape and colouration of tail, greater covert and head feathers. Detailed photographs were taken of all birds, and ages were independently corroborated by a second observer (I.B). Not all Whitethroats can be aged with confidence due to intermediate features, and in this study four males were not assigned ages (EURING code 4).
Screening blood samples for malaria
A blood sample was obtained from each bird as a by-product of taking a claw clipping for stable isotope analysis (data not included in this study) using scissors. Birds were released immediately after claw clipping to reduce stress, and any noticeably stressed birds were released without a blood sample being taken (n = 1). The blood sample (ca. 5 µl) was stored in 700 µl of 100% ethanol. DNA extraction was performed using the salt method (Aljanabi & Martinez Citation1997). Individuals were molecularly sexed to check DNA viability using the methods described by Griffiths et al. (Citation1998).
Malaria screening was performed using a nested polymerase chain reaction (PCR) technique described by Hellgren et al. (Citation2004) and Waldenström et al. (Citation2004) which amplifies a fragment of the malarial cytochrome b gene. This method has been found to be more accurate at detecting malaria infection than traditional blood smears (Perkins et al. Citation1998, Richard et al. Citation2002). Reactions in the initial PCR, which amplifies a 570 bp region, were set up in 10 µl total volumes using 5 µl TopTaq DNA polymerase solution (QIAGEN), 3.2 µl water, 0.4 µl (10 mM) of each primer HAEMNF1 and HAEMNR3 (Hellgren et al. Citation2004), and 1.0 µl of DNA (25 ng/µl). Reaction conditions described by Waldenström et al. (Citation2004) were used. All reactions included positive controls from individuals known to be infected with the strain being tested for and negative controls of double-distilled water.
The PCR amplicon from this reaction was then used in two subsequent nested PCRs, one to detect Plasmodium and Haemoproteus spp. (480 bp), the other to detect Leucocytozoon spp. (478 bp). Both reactions used the same volumes from the initial PCR, with primers HAEMF and HAEMR2 (Waldenström et al. Citation2004) for Plasmodium/Haemoproteus and primers HAEMFL and HAEMR2L (Hellgren et al. Citation2004) for Leucocytozoon. Reaction conditions described by Waldenström et al. (Citation2004) were used. All samples were tested once for Leucocytozoon, and three times for Plasmodium and Haemoproteus spp.
Statistical analyses and model selection
General linear models predicting arrival timing were compared using corrected Akaike's Information Criterion (AICc; Burnham & Anderson Citation2002). Age, wing length, tarsus length, mass and infection status were included as main effects. Time of day at capture was included only in models containing mass, because mass (but not other biometrics) varies throughout the day. Site of capture was also included to identify any effects it may have had. Birds of unknown age (n = 4) were included in the models as a separate age group. Birds with missing biometric data were not included in the model selection analysis (n = 2). Interaction terms were not included due to small sample sizes, especially in the case of age interactions which analyse subsets of the data. Models were compared using the dredge function of the MuMIn package in R version 2.15.21 (Bartoń Citation2013, R Core Team Citation2012).
Model selection was repeated using only birds caught within four days of detection (n = 41) and because this did not make any substantial difference to model selection (the best model still included only age and wing, and had a ΔAIC value 1.5 units lower than the next-ranking model) the model results including all birds are presented. Model selection was repeated again using three measures of condition indices instead of body mass to test whether these would change model ranking or variable importance. Body condition indices were expressed as the residuals from linear regressions of body mass against (1) tarsus, (2) wing length and (3) tarsus and wing length, with time of day included in all models. Student's t-tests and Pearson's correlations between age groups and biometric measures are also presented in order to elucidate the interpretation of the models.
RESULTS
Fifty-one males of known arrival date were captured, as well as eight additional males without arrival dates which were included in analysis of biometrics. Sixty per cent of males were caught within one day of detection, 70% within three, and 90% were caught within six days. The earliest and latest detected male arrivals were 16 April and 16 May 2012, respectively.
Adult males had significantly longer wings than second years (t 55 = 3.0, P < 0.01), with an average difference of 1.4 mm. There was no significant difference between tarsus (t 50 = 0.4, P = 0.7) or body mass (controlled for time of day captured; t 55 = 0.7, P = 0.5) between the two groups. Overall mass was correlated with both wing length and tarsus (Pearson's correlation: mass with wing: r 59 = 0.45, P < 0.001; mass with tarsus: r 54 = 0.28, P < 0.05) but there was no correlation between wing length and tarsus (r 54 = 0.15, P = 0.27). However, there were marked differences in morphological correlations between the two age groups. Adults had moderate correlations between all morphological features (mass with wing: r 27 = 0.44, P < 0.05; mass with tarsus: r 26 = 0.44, P < 0.05; wing with tarsus: r 26 = 0.53, P < 0.01); however, second years only had significant correlation between mass and wing length (mass with wing: r 28 = 0.53, P < 0.01; mass with tarsus: r 24 = 0.15, P = 0.47; wing with tarsus: r 24 = 0.03, P = 0.88).
Comparing all possible models predicting arrival timing showed all top candidate models included age and wing length and the top-ranking model contained only age and wing length (). Lower-ranking models included mass and tarsus length; however, model averaging of all candidate models showed that age and wing length were the most important predictors of earlier arrival (). Models including tarsus length predicted individuals with longer tarsi arrive later.
Table 1. Competing candidate models to explain spring arrival date of 49 Common Whitethroats in terms of wing length, age, mass, time of weight, tarsus length and malaria infection.
Table 2. Relative importance and estimates of variables hypothesized to influence spring arrival date in Common Whitethroats.
The top-ranking model's Akaike weight shows that it is 2.4 times likelier than the next-ranking model (ω = 0.43 versus 0.18). The additional parameters in the lower-ranked models can be considered especially uninformative, because adding even uninformative ‘nuisance’ parameters can ‘improve’ a model's AIC by ≤ 2 ΔAIC (Arnold Citation2010). Therefore, the model including only age and wing length as predictors is a clear optimum model for predicting spring arrival data in these Whitethroats.
The selected model predicted that adults were more likely to arrive before second years, arriving on average six days earlier, and individuals with longer wings were more likely to arrive earlier than those with shorter wings within age groups (). Each additional millimetre of wing length conferred an average advance in arrival of 1.5 days for all age groups (adults, second years and un-aged birds; ). According to this model, birds of unknown ages were very similar in distribution to second years, suggesting these individuals were possibly second year birds that had undergone extensive moult before spring departure. Neither mass nor tarsus length was an important predictor of arrival date. Infection with avian malaria did not appear to affect arrival date (); however, the small sample sizes of parasitized birds (n = 5 with arrival date) limit the power of this study to test this meaningfully.
Figure 1. Wing length predicts relative spring arrival date (days after the earliest arrival) for un-aged (diamonds; solid line), second year (circles; dotted line) and adult (triangles; dashed line) Common Whitethroats. See for model details. Birds infected with malaria have black-filled shapes (n = 5); one bird with unknown infection status has a grey-filled shape. Points are jittered to avoid overlapping.
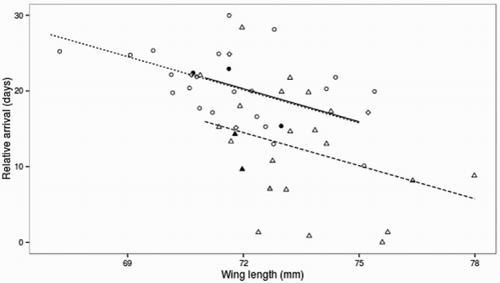
Table 3. The optimum general linear model identified by AICc (Table 1) was re-estimated using 51 birds with complete data, including birds of unknown age (n = 4).
Including body condition indices instead of mass (and excluding the biometric measure or measures used to calculate them) did not improve models, with the top-ranked models still retaining wing and age, or just age if wing was excluded from selection variables, for all three condition index measures. The next-ranking models had ΔAICc values of 1.6–2.1, and model averaging confirmed that age and wing were still the most important variables, with relative variable importance (ωi) of all three measures of condition averaged over all models between 0.22 and 0.24.
Parasitized birds were both adults and second years, and infection did not appear to affect mass, although statistical power was low due to small sample size (two-way anova including time of day; infection: F = 0.09, P = 0.8, n (infected) = 6, n (uninfected) = 52; full model: F2,58 = 2.6, R2 = 0.09, P = 0.09).
DISCUSSION
Age and wing length are shown to be the only important predictors of arrival in this study, with both being included in all top candidate models. The best model included age and wing length only, and predicted that early-arriving males were more likely to be adults and more likely to have longer wings than other individuals of the same age, with each millimetre of wing length conferring an average advance of 1.5 days in arrival. The correlation between arrival timing and wing length has been found in other studies (Francis & Cooke Citation1986, Stolt & Fransson Citation1995, Potti Citation1998). However, these studies did not always differentiate between ages or take tarsus measurements, and therefore it was not possible for them to explore the effect of wing length while controlling for those variables. More recently, wing length has been either used to create a measure of condition or included in a principal component analysis, and so the independent effect of wing length has not been presented.
The comparison of candidate models predicting arrival timing showed that age and wing length were not masking similar effects of mass or tarsus. Interestingly, tarsus length, which is considered along with mass to be the best predictor of true skeletal size (Freeman & Jackson Citation1990, Senar & Pascual Citation1997) had no relationship with arrival in this study, which is what would be expected if skeletal size were important. This concurs with other studies, which have not reported any link between arrival timing and tarsus size. The relationship between tarsus and body size was not clear in this study, because tarsus length was significantly correlated with mass and wing in adults, but had no correlation with either in second years. Further sampling is needed to clarify whether this reflects true patterns in this species and others, or is an effect of small sample sizes.
Mass did not have a significant association with arrival timing in this study, although interpreting this measure is problematic due to the fact it represents both body size as well as fat deposition and muscle tissue. Replacing it with a measure of condition did not improve its explanatory power, and indeed excluding wing length (where this was used to control for body size) resulted in much poorer models. Results of other studies of male passerines are extremely mixed, with some finding no relationship between arrival and body mass or condition (Forstmeier Citation2002, Norris et al. Citation2004, Mitrus Citation2007), and others reporting the earlier arrival of birds in better condition (Arvidson & Neergaard Citation1991, Marra et al. Citation1998, Ninni et al. Citation2004). It is likely that this is due to trade-offs between condition and arrival timing which may be different between years and species, and may be related to migration conditions.
The importance of long wings may be due to the possible advantage they confer in flight speed and efficiency (Ellegren Citation1993, Yong & Moore Citation1994), especially over large distances which may amplify the effects of even small morphological differences. Wing length may also reflect condition at the time of feather growth, particularly under challenging environmental conditions or heavy parasite infection which can lead to constrained feather growth in adults (Hall & Fransson Citation2000, Dawson et al. Citation2000, Vágási et al. Citation2012) and nestlings (Øyan & Anker-Nilssen Citation1996, Benowitz-Fredericks et al. Citation2006). Thus, any relationships between wing length and arrival timing could reflect carry-over effects of conditions experienced by individuals at earlier points in their life. This relationship between past condition and spring arrival timing has been demonstrated clearly by Marra et al. (Citation1998) and Studds & Marra (Citation2005) in American Redstarts, Setophaga ruticilla.
Age was also an important factor in determining arrival timing in this study. Age-related differential timing of spring migration is a common phenomenon in many migrant passerine bird species, with adults typically arriving before second years in both males and females (Lozano et al. Citation1996, Smith and Moore Citation2005, Cooper et al. Citation2009). Age-related differences in wing length have been suggested as a possible cause of this pattern (Stewart et al. Citation2002), with adults typically having longer wings in many species (Alatalo et al. Citation1984). Additionally, adults may be more likely to occupy good quality winter sites which may facilitate earlier departure on spring migration (Marra et al. Citation1998, Studds & Marra Citation2005). However, although differences in wing length and condition may account for some or most of the variation in arrival time, it is likely that other age-dependent factors such as previous experience of spring migration may be important, and there is indeed some evidence that individual birds arrive earlier in their second spring migration (Saino et al. Citation2004); however, it is not known for certain whether this is independent of age-related changes in morphology.
No association between haemosporidian infection and arrival date was found in this study, though few parasitized individuals were found. As arrival date may be seen as a measure of fitness, one might expect to observe an association between parasite infection and arrival date in populations with higher prevalence, and there is some evidence that such an association with blood parasites may exist in populations of Pied Flycatcher (Rätti et al. Citation1993) and Barn Swallow (Møller et al. Citation2004). However, it appears to be a weak association limited to certain strains or populations and recent studies have found no link (Asghar et al. Citation2011, López et al. Citation2013), showing the need for further research into this ecological relationship.
This study highlights the need to explore biometric data fully before deciding the most appropriate way to treat them in analyses. Relationships between biometrics may differ between species and age-related differences in biometric correlations within a species, as found in this study, may mean separate analyses for each age class would be appropriate, if sample sizes allow. Although it is recognized that other measures of morphology such as wing loading and pointedness (Bowlin & Wikelski Citation2008) and accurate fat measures (Sandberg Citation1996, Smith & Moore Citation2003) may add further understanding to arrival patterns, this study informs the best use of the most commonly collected biometrics. The results of this study suggest that wing length is important in arrival timing due to some factor other than just structural body size, conceivably either its association with flight efficiency or its relationship with past condition, and studies focusing on arrival timing should take this into account.
ACKNOWLEDGEMENTS
Thanks to Neal Armour-Chelu at the Forestry Commission for permission to work in Thetford Forest and Greg Conway of Thetford Forest Ringing Group, as well as to those at Anglian Water and Norwich City Council who gave permission to ring at various sites across Norwich. Special thanks to Kate Risely, Lucy Ryan and Mike Toms for assistance in the field and with the manuscript. We are grateful to Jenny Gill and Dave Leech for helpful comments on the early manuscript, and to Will Cresswell and two anonymous reviewers for their comments which significantly improved the final article.
REFERENCES
- Alatalo, R.V., Gustafsson, L. & Lundberg, A. 1984. Why do young passerine birds have shorter wings than older birds? Ibis 126: 410–415. doi: 10.1111/j.1474-919X.1984.tb00264.x
- Aljanabi, S.M. & Martinez, I. 1997. Universal and rapid salt extraction of high quality DNA for PCR-based techniques. Nucleic Acids Res. 25: 4692–4693. doi: 10.1093/nar/25.22.4692
- Arnold, T.W. 2010. Uninformative parameters and model selection using Akaike's information criterion. J. Wildlife Manage. 74: 1175–1178.
- Arvidson, B.L. & Neergaard, R. 1991. Mate choice in the Willow Warbler – a field experiment. Behav. Ecol. Sociobiol. 29: 225–229. doi: 10.1007/BF00166406
- Asghar, M., Hasselquist, D. & Bensch, S. 2011. Are chronic avian haemosporidian infections costly in wild birds? J. Avian Biol. 42: 530–537. doi: 10.1111/j.1600-048X.2011.05281.x
- Bartoń, K. 2013. MuMIn: multi-model inference. R package version 1.9.5. Available from: http://CRAN.R-project.org/package=MuMIn
- Benowitz-Fredericks, Z.M., Kitaysky, A.S. & Thompson, C.W. 2006. Growth and allocation in captive Common Murre (Uria aalge) chicks. Auk 123: 722–734. doi: 10.1642/0004-8038(2006)123[722:GAAICC]2.0.CO;2
- Bensch, S. & Hasselquist, D. 1991. Territory infidelity in the polygynous Great Reed Warbler Acrocephalus arundinaceus: the effect of variation in territory attractiveness. J. Anim. Ecol. 60: 857–871. doi: 10.2307/5418
- Both, C. & Visser, V.E. 2001. Adjustment to climate change is constrained by arrival date in a long-distance migrant bird. Nature 411: 296–298. doi: 10.1038/35077063
- Bowlin, M.S. & Wikelski, M. 2008. Pointed wings, low wingloading and calm air reduce migratory flight costs in songbirds. PLoS ONE 3: e2154. doi:10.1371/journal.pone.0002154. doi: 10.1371/journal.pone.0002154
- Burnham, K.P. & Anderson, D.R. 2002. Model Selection and Multimodel Inference, 2nd edn. Springer, New York.
- Cooper, N.W., Murphy, M.T. & Redmond, L.J. 2009. Age- and sex-dependent spring arrival dates of Eastern Kingbirds. J. Field Ornithol. 80: 35–41. doi: 10.1111/j.1557-9263.2009.00203.x
- Cramp, S. & Brook, D.J. 1992. The Birds of the Western Palearctic, Vol. 6. Oxford University Press, London.
- Dawson, A., Hinsley, S.A., Ferns, P.N., Bonser, R.H.C. & Eccleston, L. 2000. Rate of moult affects feather quality: a mechanism linking current reproductive effort to future survival. Proc. R. Soc. B 267: 2093–2098. doi: 10.1098/rspb.2000.1254
- Dyrcz, A., Wink, M., Kruszewicz, A. & Leisler, B. 2005. Male reproductive success is correlated with blood parasite levels and body condition in the promiscuous Aquatic Warbler (Acrocephalus paludicola). Auk 122: 558–565. doi: 10.1642/0004-8038(2005)122[0558:MRSICW]2.0.CO;2
- Ellegren, H. 1993. Speed of migration and migratory flight lengths of passerine birds ringed during autumn migration in Sweden. Ornis Scand. 24: 220–228. doi: 10.2307/3676737
- Forstmeier, W. 2002. Benefits of early arrival at breeding grounds vary between males. J. Anim. Ecol. 71: 1–9. doi: 10.1046/j.0021-8790.2001.00569.x
- Francis, C.M. & Cooke, F. 1986. Differential timing of spring migration in wood warblers (Parulinae). Auk 103: 548–556.
- Freeman, S. & Jackson, W.M. 1990. Univariate metrics are not adequate to measure avian body size. Auk 107: 69–74.
- Green, A.J. 2001. Mass/length residuals: measures of body condition or generators of spurious results? Ecology 82: 1473–1483. doi: 10.1890/0012-9658(2001)082[1473:MLRMOB]2.0.CO;2
- Griffiths, R., Double, M.C., Orr, K. & Dawson, R.J.G. 1998. A DNA test to sex most birds. Mol. Ecol. 7: 1071–1076. doi: 10.1046/j.1365-294x.1998.00389.x
- Gunnarsson, T.G., Gill, J.A., Newton, J., Potts, P.M. & Sutherland W.J. 2005. Seasonal matching of habitat quality and fitness in a migratory bird. Proc. R. Soc. B 272: 2319–2323. doi: 10.1098/rspb.2005.3214
- Hall, K.S.S. & Fransson, T. 2000. Lesser Whitethroats under time-constraint moult more rapidly and grow shorter wing feathers. J. Avian Biol. 31: 583–587. doi: 10.1034/j.1600-048X.2000.310419.x
- Hellgren, O., Waldenström, J. & Bensch, S. 2004. A new PCR assay for simultaneous studies of Leucocytozoon, Plasmodium, and Haemoproteus from avian blood. J. Parasitol. 90: 797–802. doi: 10.1645/GE-184R1
- Jenni, L. & Winkler, R. 1994. Moult and Ageing of European Passerines. Academic Press, London.
- Krebs, C.J. & Singleton, G.R. 1993. Indexes of condition for small mammals. Aust. J. Zool. 41: 317–323. doi: 10.1071/ZO9930317
- López, G., Muñoz, J., Soriguer, R. & Figuerola, J. 2013. Increased endoparasite infection in late-arriving individuals of a trans-Saharan passerine migrant bird. PLoS ONE 8: e61236. doi: 10.1371/journal.pone.0061236
- Lozano, G.A., Perreault, S. & Lemon, R.E. 1996. Age, arrival date and reproductive success of male American Redstarts Setophaga ruticilla. J. Avian Biol. 27: 164–170. doi: 10.2307/3677146
- Marra, P.P., Hobson, K.A. & Holmes, R.T. 1998. Linking winter and summer events in a migratory bird by using stable-carbon isotopes. Science 282: 1884–1886. doi: 10.1126/science.282.5395.1884
- Marzal, A., de Lope, F., Navarro, C. & Møller, A.P. 2005. Malarial parasites decrease reproductive success: an experimental study in a passerine bird. Oecologia 142: 541–545. doi: 10.1007/s00442-004-1757-2
- Mitrus, C. 2007. Is the later arrival of young male red-breasted flycatchers (Ficedula parva) related to their physical condition? J. Ornithol. 148: 53–58. doi: 10.1007/s10336-006-0099-8
- Møller, A.P., de Lope, F. & Saino, N. 2004. Parasitism, immunity and arrival date in a migratory bird, the Barn Swallow. Ecology 85: 206–219. doi: 10.1890/02-0451
- Ninni, P., De Lope, F., Saino, N., Haussy, C. & Møller, A.P. 2004. Antioxidants and condition-dependence of arrival date in a migratory passerine. Oikos 105: 55–64. doi: 10.1111/j.0030-1299.2004.12516.x
- Norris, D.R., Marra, P.P., Kyser, T.K., Sherry, T.W. & Ratcliffe, L.M. 2004. Tropical winter habitat limits reproductive success on the temperate breeding grounds in a migratory bird. Proc. R. Soc. B 271: 59–64. doi: 10.1098/rspb.2003.2569
- Øyan, H.S. & Anker-Nilssen, T. 1996. Allocation of growth in food stressed Atlantic puffin chicks. Auk 113: 830–841. doi: 10.2307/4088861
- Perkins, S.L., Osgood, S.M. & Schall, J.J. 1998. Use of PCR for detection of subpatent infections of lizard malaria: Implications for epizootiology. Mol. Ecol. 7: 1587–1590. doi: 10.1046/j.1365-294x.1998.00496.x
- Potti, J. 1998. Arrival time from spring migration in male Pied Flycatchers: individual consistency and familial resemblance. Condor 100: 702–708. doi: 10.2307/1369752
- Rätti, O., Dufva, R. & Alatalo, R.V. 1993. Blood parasites and male fitness in the Pied Flycatcher. Oecologia 96: 410–414. doi: 10.1007/BF00317512
- R Core Team. 2012. R: A Language and Environment for Statistical Computing. R Foundation for Statistical Computing, Vienna, Austria. ISBN 3-900051-07-0. Available from: http://www.R-project.org/
- Redfern, C.P.F. & Clark, J.A. 2001. Ringers’ Manual, 4th edn. British Trust for Ornithology, Thetford.
- Reist, J.D. 1985. An empirical evaluation of several univariate methods that adjust for size variation in morphometric data. Can. J. Zoolog. 63: 1429–1439. doi: 10.1139/z85-213
- Richard, F.A., Sehgal, R.N., Jones, H.I. & Smith, T.B. 2002. A comparative analysis of PCR-based detection methods for avian malaria. J. Parasitol. 88: 819–822.
- Ricklefs, R.E. 1992. Embryonic development period and the prevalence of avian malaria parasites. Proc. Natl. Acad. Sci. USA 89: 4722–4725. doi: 10.1073/pnas.89.10.4722
- Saino, N., Szép, T., Romano, M., Rubolini, D., Spina, F. & Møller, A.P. 2004. Ecological conditions during winter predict arrival date at the breeding quarters in a trans-Saharan migratory bird. Ecol. Lett. 7: 21–25. doi: 10.1046/j.1461-0248.2003.00553.x
- Sandberg, R. 1996. Fat reserves of migrating passerines at arrival on the breeding grounds in Swedish Lapland. Ibis 138: 514–524. doi: 10.1111/j.1474-919X.1996.tb08072.x
- Senar, J.C. & Pascual, J. 1997. Keel and tarsus length may provide a good predictor of avian body size. Ardea 85: 269–274.
- Sillett, T.S. & Holmes, R.T. 2001. Variation in survivorship of a migratory songbird throughout its annual cycle. J. Anim. Ecol. 71: 296–308. doi: 10.1046/j.1365-2656.2002.00599.x
- Slater, P.J. 1994. Factors affecting the efficiency of the area search method of censusing birds in open forests and woodlands. Emu 94: 9–16. doi: 10.1071/MU9940009
- Smith, R.J. & Moore, F.R. 2003. Arrival fat and reproductive performance in a long-distance passerine migrant. Oecologia 134: 325–331.
- Smith, R.J. & Moore, F.R. 2005. Arrival timing and seasonal reproductive performance in a long-distance migratory landbird. Behav. Ecol. Sociobiol. 57: 231–239. doi: 10.1007/s00265-004-0855-9
- Stewart, R.L.M., Francis, C.M. & Massey, C. 2002. Age-related differential timing of spring migration within sexes in passerines. Wilson Bull. 114: 264–271. doi: 10.1676/0043-5643(2002)114[0264:ARDTOS]2.0.CO;2
- Stolt, B.O. & Fransson, T. 1995. Body mass, wing length and spring arrival of the Ortolan Bunting Emberiza hortulana. Ornis Fennica 72: 14–18.
- Studds, C.E. & Marra, P.P. 2005. Non-breeding habitat occupancy and population processes: an upgrade experiment with a migratory bird. Ecology 86: 2380–2385. doi: 10.1890/04-1145
- Svensson, L. 1992. Identification Guide to European Passerines, 4th edn. Marstatryck, Stockholm.
- Vágási, C.I., Pap, P.L., Vincze, O., Benkõ, Z., Marton, A. & Barta, Z. 2012. Haste makes waste but condition matters: molt rate – feather quality trade-off in a sedentary songbird. PLoS ONE 7: e40651. doi: 10.1371/journal.pone.0040651
- Waldenström, J., Bensch, S., Hasselquist, D. & Östman, Ö. 2004. A new nested PCR method very efficient in detecting Plasmodium and Haemoproteus infections from avian blood. J. Parasitol. 90: 191–194. doi: 10.1645/GE-3221RN
- Yong, W. & Moore, F.R. 1994. Flight morphology, energetic condition, and the stopover biology of migrating thrushes. Auk 11: 683–692.