Abstract
Capsule Cereal harvesting creates high-quality but ephemeral foraging habitats for invertebrate predators.
Aims To investigate how cereal harvesting affects foraging decisions and hunting success of Lesser Kestrels.
Methods Habitat selection in response to changing availability of cereal fields (as patches being harvested are turned into stubble) was assessed by transects around colonies to count foraging birds. Focal observations of foraging kestrels were performed to assess hunting success and intake rate before and after harvesting. We performed transects to count Orthoptera to evaluate the impact of cereal cutting on prey abundance.
Results Harvesting impacted prey accessibility due to a temporary flush of prey, which resulted in a significant reduction in foraging time and an increase in the intake rate of kestrels. Accordingly, patches being harvested became the most preferred habitat. Nonetheless, harvesting likely caused high orthopteran mortality and dispersal leading to a gradual decline in prey abundance in stubbles. Lower prey abundance increased foraging time and reduced intake rate, and stubbles became avoided by foraging individuals.
Conclusion Although harvesting significantly increases foraging opportunities for Lesser Kestrels through intake rate maximization, patches being harvested represent an ephemeral high-quality habitat and its benefits are relatively short-lived as cereals are converted into low-quality stubbles.
Farmland birds have been suffering population declines due to a shift towards agricultural intensification and mechanization (Chamberlain et al. Citation2000, Donald et al. Citation2002, Newton Citation2004). How farming practices affect the quality of foraging habitats and the survival of bird populations has therefore been the subject of detailed research in the last decades (Johst et al. Citation2001, Tichit et al. Citation2005, Kleijn et al. Citation2010). In farmland landscapes, human land-use generates a patchy and dynamic distribution of food resources with important ecological implications for farmland birds (Milner et al. Citation1989, Johst et al. Citation2001, Butler et al. Citation2010). To maximize their rate of energy intake, foragers should be time minimizers or energy maximizers. Thus, foraging strategies might include preferentially choosing the largest prey, the prey with the shortest handling time or the prey that is easiest to find (Stephens & Krebs Citation1986). In arable landscapes, owing to spatial and temporal heterogeneity in food distribution, these decisions can become complicated, especially to central-place foragers that need to return to the nest between foraging bouts. Here, one main driver of foraging habitat suitability and ultimately of energetic gain is vegetation structure, because it has a major effect on food resource availability and accessibility (Moreira Citation1999, Vickery et al. Citation2001, Atkinson et al. Citation2004; Devereux et al. Citation2004). Vegetation structure is very dependent on agricultural management practices such as grazing and mowing, which may have a dual role on bird populations. On one hand, mechanical ploughing, mowing and harvesting have been suggested as one main driver of the decline of ground-nesting farmland birds through mowing-induced nest destruction and mortality (Ena et al. Citation1987, Green et al. Citation1997, Grüebler et al. Citation2008, Tews et al. Citation2013). However, at the same time, grass and cereal cutting are known to provide good foraging opportunities for many species through increased prey detection (Shrubb Citation1980, Vickery et al. Citation2001, Atkinson et al. Citation2005, Whittingham & Devereux Citation2008). Although freshly mowed fields and cereal stubbles have been previously documented as preferred foraging habitat for many species (Vickery et al. Citation2001, Butler et al. Citation2005, Peggie et al. Citation2011), the impact of cutting the vegetation, presumably due to a trade-off between prey accessibility and prey abundance, for the foraging strategies of birds have been little investigated (but see Peggie et al. Citation2011).
The Lesser Kestrel, a small migratory falcon, inhabits heterogeneous landscapes of high conservation value in Southern Europe (Tucker & Heath Citation1994, Suárez et al. Citation1997, Tucker & Evans Citation1997). In such landscapes, the extensive cultivation of cereals is done on a rotational basis, creating a dynamic mosaic of habitats such as cereal fields, stubbles, ploughed and fallow land. Moreover, the availability of each habitat changes through the year according to the schedule of agricultural practices. Lesser Kestrels forage predominantly on invertebrates and previous research has shown that they depend on the maintenance of a diverse agricultural mosaic (Tella et al. Citation1998, Franco et al. Citation2004). However, whilst many studies have investigated foraging preferences during the breeding period, few have considered the response of Lesser Kestrels to seasonal variation of habitats and prey availability due to agricultural management (but see Rodríguez et al. Citation2013). Each year, during the nestling period when food demand is highest, cereal fields are harvested and subsequently transformed into stubble. Vegetation structure is dramatically transformed and the tall vegetation of cereal crops is replaced by the short vegetation landscape of stubble fields, likely impacting prey availability and foraging decisions of other insectivorous species (Delgado & Moreira Citation2000, Palacín et al. Citation2011). Cereal fields are either selected (Donázar et al. Citation1993, Tella et al. Citation1998) or avoided (Bonal & Aparício Citation2001, Cordeiro et al. Citation2005, Ursúa et al. Citation2005, García et al. Citation2006) by Lesser Kestrels while cereal stubbles, often not included in habitat selection analyses (Donázar et al. Citation1993, García et al. Citation2006), seem to be a preferred habitat for foraging individuals (Bonal & Aparício Citation2001, Franco et al. Citation2004, Cordeiro et al. Citation2005). Nonetheless, these studies did not consider the harvest period (but see Catry et al. Citation2012) and the potential decay in the quality of stubble fields within the season.
In this study, we aimed to investigate how the potential trade-offs of cereal harvesting affects foraging decisions of Lesser Kestrels and how long these effects may last. In particular, we assessed how harvest impacts availability and accessibility of prey and hunting success and ultimately habitat selection of Lesser Kestrels. If harvest increases accessibility to prey due to a temporary prey flush caused by the sudden removal of vegetation cover, we expected that cereals being harvested become a high-quality foraging habitat for Lesser Kestrels, with higher intake rates. However, mechanical harvest is expected to cause a decline in prey abundance owing to an increase in harvest-induced mortality and prey movement due to lack of refuges (Humbert et al. Citation2010, Citation2012). Therefore, after harvest, cereal stubbles are expected to provide reduced intake rates and to be avoided or less used by Lesser Kestrels. Moreover, since Lesser Kestrels are central-place foragers and the distance to the colony is known to play a crucial role in habitat selection (Franco et al. Citation2004, Catry et al. Citation2012, Rodríguez et al. Citation2013), we expect that the benefit of selecting patches being harvested decreases with the distance to the colony.
METHODS
Study area and species
The study was carried out in the Castro Verde Special Protection Area (SPA), a cereal steppe landscape characterized by the extensive cultivation of dry cereals in the south of Portugal. Cereals are cultivated under a low-intensity rotational system in which, after 2 years of cereal cultivation, the land is left uncultivated for 3–5 years (fallows). Therefore, the landscape is dominated by fallow fields (usually over 50% of the area). The fields are ploughed and cultivated in September–November; cereal fields are harvested in May and June and transformed into stubble. The two largest Lesser Kestrel colonies, located in the ruins of abandoned farmhouses and 30 km apart, Belver (n = 70 pairs) and Pardieiro (n = 65 pairs), were selected for this study. Lesser Kestrels arrive from their wintering areas in early February (Catry et al. Citation2011) and typically lay in April and May. Incubation takes 28 days and after hatching both parents feed the chicks for about 37 days (Bustamante & Negro Citation1994). During the chick-rearing period, Lesser Kestrels feed on invertebrates, mainly large Orthoptera (Rodríguez et al. Citation2010) such as Tettigoniidae and Acrididae (I. Catry and A. Franco unpubl. data).
Foraging habitat selection
The area around the two colonies (3 km radius) was scanned uniformly along 3-hour-driven transects (approximately 20 km) between mid-May and mid-July 2008 (n = 9 and 11 transects for Belver and Pardieiro, respectively). All foraging birds were recorded in 1:25 000 maps along with habitat type. Land-use within this radius was classified into five classes: fallow, cereal, cereal being harvested (when a harvesting machine was working), cereal stubble and others (including residual areas of ploughed fields, leguminous crops, forestations, scrubland, holm and cork oak woods, orchards, horticultural areas and water courses). Temporal changes to land-use distribution during the breeding season were recorded when cereal fields started being harvested in order to estimate the proportion of each habitat (cereal, cereal being harvested and cereal stubble) around the colonies when the transects were performed. Habitat selection in each colony and in each sampling period (before and after harvest started) was analysed using the Savage Selectivity Index (Savage Citation1931) , where Ui
is the proportion of observations recorded in a given habitat and pi
is the proportion of that habitat against total available habitat. Selection indices above 1.0 indicate preferences; values less than 1.0 indicate avoidance. Because this index varies from 0 (maximum negative selection) to ∞ (maximum positive selection), Manly et al. (Citation1993) suggest presenting a Standardized Selection Index (Bi),
, that sum 1.0 for all classes of the resource. Index values of (1/number of habitats) indicate no preference; higher values indicate a preference and lower values avoidance. The statistical significance of this index was tested by comparing the statistics
with the corresponding critical value of a chi-squared distribution with one degree of freedom (Manly et al. Citation1993). The standard error of the index
was calculated by
, u
+ being the total number of foraging observations sampled. Statistical significance was obtained after applying the Bonferroni correction for the number of statistical tests. We calculated the Savage Selectivity Index for the period before and after the farmers started harvesting the cereal, with the only difference in habitat availability between the two periods being the amount of unharvested cereal, cereal being harvested and stubble fields. All analyses were implemented using the adehabitat package in R software (Calenge Citation2006, Development Core Team R Citation2010).
Harvesting and Lesser Kestrel behaviour
From mid-June to early July, during the chick-rearing period, we investigated the foraging behaviour of Lesser Kestrels in relation to harvesting activity around the colonies. From high vantage points, we performed observations (2-hour periods on 13 different days when harvesting was taking place) of birds leaving the colony after feeding the chicks, recording the direction of all departures (n = 167). To ensure that the departure direction was a good proxy of a given foraging direction, we only considered direct flights, i.e. from the time the bird left the colony till it started hovering. For all the observation periods we registered the location of the harvesting machine and estimated its distance from the colony. At the Belver colony, for three additional days we compared departure directions (n = 546) before and after a harvesting machine started working (approximately 1 hour before and 1 hour after harvest began) in the surroundings of the colony to assess changes in foraging decisions of Lesser Kestrels in relation to agricultural activities. Furthermore, on eight days we recorded the pattern of arrivals of Lesser Kestrels to the nearest cereal patch being harvested around the colony of Belver. From a good visibility point nearby the patch being harvested we counted, every 3 minutes and during ca. 3 hours, the number of birds present in the selected patch, before (with no machine or with a baler working in an adjacent area) and after the harvesting machine started working. For two of the days, we also continued the counts after the harvesting machine had finished.
All observations were made in the morning (8:00–12:00) and afternoon (16:00–20:00) when kestrels are more active and avoiding unfavourable weather (such as very windy days).
Hunting success, prey biomass and expected intake rate
To assess the time spent capturing a prey in each habitat and the resulting intake rate, hunting sequences of adults known to be feeding chicks were recorded in fallows (n = 265), cereal fields (n = 130), cereal patches being harvested (n = 112) and stubbles (n = 63). For each bird, we recorded (1) the number of seconds necessary to capture a prey from the start of hovering and, whenever possible, (2) prey type and (3) approximate size of captured prey (using bill size for comparison) within three categories: class 1 (<2.5 cm), class 2 (2.5–3.5 cm) and class 3 (>3.5 cm). To determine prey biomass ranges for each class size we measured and weighed fresh Tettigoniidae and Acrididae (Orthoptera) prey items found in nests and sampled in the field during the breeding season (n = 43 and 67, respectively). Prey biomass for each class was set as: class 1 (0.4–0.8 g), class 2 (0.8–1.5 g) and class 3 (1.5–3.0 g). The lower limit of class 1 was set at 0.4 g as no lighter prey were found in nests. The expected prey biomass in each habitat type was calculated as the sum of the proportion of each prey size category per patch multiplied by the mean fresh mass of each prey size category The expected intake rate for each habitat according to its distance from the colony, was calculated as a / (b + c), where a is the expected prey biomass, b the time required to catch a prey item and c the travelling time to the patch and back (Catry et al. Citation2012). Flight speed was assessed using a linear regression to model the relationship between the distance from the colony and the recorded flight time of 54 kestrels returning to the colony, after capturing a prey at a known location (R2 = 0.55, F 1, 52 = 66.02, P < 0.001). Comparisons of time to obtain a prey item and prey size across habitats were assessed using Kruskal–Wallis followed by post hoc Tukey tests.
Prey abundance
Orthoptera relative abundance was measured by direct counts along 40 m walked transects in fallow (n = 60) and stubble patches (n = 127) randomly distributed within the 3 km radius of the colony during sunny days (range 25–30°C). At each randomly chosen location, four perpendicular transects were carried out (N, S, E and W), beginning from a central point. Counts were made within 30 cm either side of the transect line, invertebrates encountered leaving this strip were included, those entering were ignored. To evaluate the effect of time since harvesting on the abundance of invertebrates we distinguished between stubbles with different ages: less than one day since cutting, 1, 2 and 3–7 days since cutting (n = 20, 20, 52 and 35, respectively). Comparisons of Orthoptera abundance in stubbles across time were assessed using Kruskal–Wallis followed by post hoc Tukey tests. In addition, fallows and stubbles were also compared by a Mann–Whitney U test. Prey abundance was not assessed in cereal fields as vegetation height decreases prey detection not allowing the comparison with other habitats using the transect methodology. In patches being harvested, working machinery also affected accurate counts of grasshoppers. Therefore, in cereal fields being harvested we tried to demonstrate the temporary food peak due to harvesting from visual observations. Using binoculars, we choose one fixed point ca. 20 m from the strip being harvested and counted the number of flying grasshoppers in the same field of vision from around 1 minute before to 1 minute after the harvesting machine passed (n = 33). Although this method may be biased towards counting larger individuals, it can give an idea of prey availability during harvesting.
RESULTS
Foraging habitat selection
A total of 611 foraging observations were recorded in the two colonies, 347 before and 264 after the beginning of harvest (n = 157 and 190 before harvest, n = 121 and 143 after harvest for Belver and Pardieiro colonies, respectively). Lesser Kestrels showed different habitat selection depending on the colony and agricultural period. Before harvest, fallows and cereal fields were used in the proportion of their availability in both colonies (, ). From the beginning of harvest, foraging Lesser Kestrels positively and significantly selected cereal fields being harvested whilst stubbles were avoided (, ). During the harvesting period, Lesser Kestrels negatively selected fallows and cereal fields around Belver and Pardieiro colonies, respectively (, ).
Figure 1. Foraging habitat selection of Lesser Kestrels before and after the beginning of harvest in (a, b) Belver and (c, d) Pardieiro colonies, respectively. Bars represent the percentage of available (white) and used (grey) habitats by foraging Lesser Kestrels. Significant values for selection (+) and avoidance (−) according to the Savage Selectivity Index are shown (* P < 0.05; ** P < 0.01; *** P < 0.001).
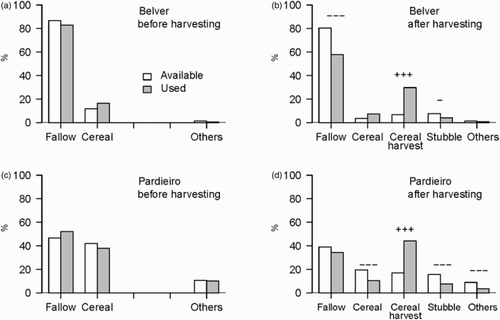
Table 1. Values of Savage Selectivity index (ωi), standard error (se) and significance level (P) for each habitat used by Lesser Kestrels around the two studied colonies before and after the beginning of the harvested season. Standardized Selection Indices (Bi) > 0.20 and 0.33 (1/number of habitats) for the periods before and after harvesting, respectively, indicate preference.
Harvesting and Lesser Kestrel behaviour
The comparison of departure directions in periods before and after the beginning of harvesting show that birds shift their departure patterns, increasing the total departures in the direction of the harvest machine once harvesting starts (χ2 = 30.1, P < 0.001, n = 546; ). shows the arrivals of birds to patches being harvested. Whilst the number of Lesser Kestrels foraging in stubble before the harvesting machine starts working is small, there is a significant and rapid increase in the number of birds foraging as soon as harvest starts. The presence of a baler working seems to attract some birds but not as many as a harvesting machine. The number of birds strongly declines after the harvesting machine stops ().
Figure 2. Shift of directions in departures from the colony before and after the beginning of harvesting. Observations were performed in three different days of June ca. 1 hour before and 1 hour after the beginning of harvesting (n = 546). The harvest machine was located north to the colony.
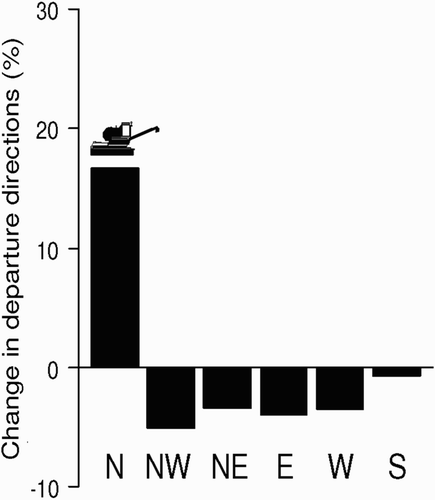
Figure 3. Arrival pattern of foraging Lesser Kestrels to a patch before, during and after harvesting. Time zero represents the start of harvesting and the grey rectangle defines the harvesting period. Dots show the number of foraging Lesser Kestrels counted every 3 minutes in patches half harvested and include three situations: with no working machinery (white dots), with a machine bailing cereal straw in the area harvested the previous day (grey dots) and with a harvesting machine (black dots).
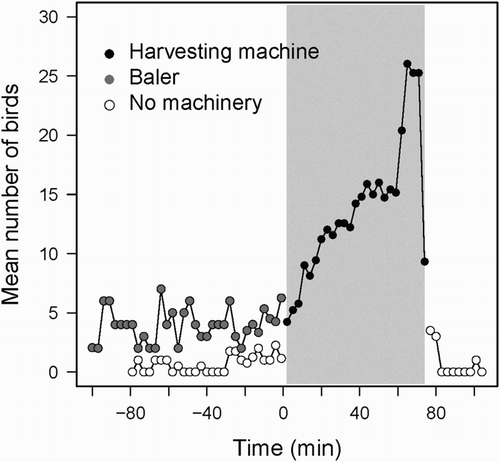
Hunting success, prey biomass and expected intake rate
Time needed to obtain a prey was significantly lower in patches being harvested, followed by fallows, stubbles and cereal fields (, P < 0.001 followed by post hoc Tukey tests; ). Prey biomass also varied with habitat type (
, P < 0.001) being higher in cereal fields (). Close to the colony, final intake rate was considerably higher in patches being harvested, followed by fallows, cereal fields and stubbles. Nonetheless, the advantage of foraging near a harvesting machine falls quickly as the distance from the colony increases (). This is probably the cause for the observed significant decline in the proportion of birds heading towards a patch being harvested as the distance between the colony and the harvesting machine increased (F
1,11 = 56.6, P < 0.001; ).
Prey abundance
Grasshoppers were significantly more abundant in fallows compared to stubble fields (mean grasshopper/transect = 47.0 ± 3.4 and 23.9 ± 0.4 for fallows and stubbles, respectively; W = 1679, P < 0.001). During harvest, the passage of a harvesting machine increased prey accessibility due to a temporary prey flush caused by the sudden removal of vegetation cover (). Nonetheless, time since cereal cutting was shown to negatively impact Orthoptera abundance in stubbles (, P < 0.001, followed by post hoc Tukey tests), because it was lower in older stubbles (more than 3 days since cutting) when compared to freshly harvested stubbles (less than 24 hours; ).
DISCUSSION
Land-use management significantly alters the vegetation structure and cover of farmland landscapes and strongly influences the abundance, quality and distribution of invertebrate prey (Johst et al. Citation2001). The impacts of agricultural activities on prey availability and accessibility for foraging birds can either be positive or negative and more or less transitory. Agricultural activities such as ploughing and harvesting likely increase access to prey for many species (Vickery et al. Citation2001, Peggie et al. Citation2011) and Lesser Kestrels are often observed following farm machinery (Bijlsma et al. Citation1988, Aparicio Citation1990, Franco et al. Citation2004). However, the impact of such activities on the foraging decisions of birds at both short and long temporal scales has been little investigated. Our results show that cereal harvesting impacts the foraging behaviour of Lesser Kestrels by influencing prey accessibility, hunting success and intake rate. Cereal harvesting attracts foraging birds but there is a rapid drop off in stubble use after cutting.
Every year, cereal fields are harvested and consequently transformed into stubble. In this study, we show that cereal cutting results in a temporary flush of grasshopper availability, increasing Lesser Kestrel foraging efficiency (time needed to obtain a prey decreases by one-third in patches being harvested) and intake rate. Optimal foraging theory predicts that trade-offs amongst prey value, searching time and prey handling time affect habitat selection (Stephens & Krebs Citation1986). Accordingly, Lesser Kestrels seem to quickly recognize the increased foraging opportunity from harvesting, adapting their foraging decisions as demonstrated by the shift in departure directions from the colony and the instantaneous increase in arrivals to patches being harvested. As soon as harvest starts Lesser Kestrels flock around harvesting machinery and patches being harvested become the preferred foraging habitat. Nonetheless, a decline in prey availability and accessibility after harvest is quickly perceived by kestrels, as showed by the rapid decline in the number of foraging birds when harvesting stops. Harvesting is likely to cause high direct mortality on orthopterans (up to 85%, Humbert et al. Citation2010) and survivors might disperse quickly to undisturbed habitats (Guido & Gianella Citation2001). Indeed, during harvesting we observed grasshoppers ‘migrating’ from cereal patches being harvested to adjacent fallow fields at a rate of 3.7 grasshoppers/m2 min−1 (pers. obs.). Moreover, the removal of the standing crop alters the soil microclimate (Gardiner & Hassal Citation2009) making stubbles less adequate to orthopterans and likely contributing to the observed decline in their availability over time. As lower prey abundance increases foraging time (the time taken to locate prey) and reduces intake rate, stubbles became less used. Our results seem to agree with the findings from Peggie et al. (Citation2011). These authors highlighted that, although many previous studies showed that a range of farmland species preferred short grass swards to forage, the effects of grass cutting are ephemeral showing a rapid drop off in field use by some species after cutting. As an example, for Common Kestrels Falco tinnunculus, the use of fields after cutting declined by 50% by day 4 (Peggie et al. Citation2011). Similar impacts were described for Montagu's Harriers Circus pygargus (Pilon Citation2005).
Within the chick-rearing period, cereal crops were either used in the proportion of its availability (Ursúa et al. Citation2005) or avoided (Bonal & Aparício Citation2001, García et al. Citation2006) by foraging Lesser Kestrels depending on the colony and period. Rodríguez et al. (Citation2013) pointed out that contradictory results (Donázar et al. Citation1993 and Tella et al. Citation1998 found a positive selection of cereal crops) might be a consequence of differences in the vegetation structure which could be more important than habitat type in the process of patch selection. Invertebrate prey accessibility is likely to be lower in the taller vegetation of cereal fields decreasing foraging efficiency. Nonetheless, tall vegetation may support greater arthropod abundance than short vegetation (Morris Citation2000, Vickery et al. Citation2001) and may act as reservoirs of large prey (Catry et al. Citation2012), increasing energetic gain for Lesser Kestrels. After cutting, short vegetation of stubbles increases prey accessibility but prey size is likely to decrease as larger grasshopper individuals are more seriously impacted by harvesting than smaller grasshoppers (Oppermann et al. Citation2000).
The rapid perception of the location of the patches being harvested suggests that Lesser Kestrels may acquire information on the location of the harvesting machine by monitoring others' interactions with the environment. At the colony, Lesser Kestrels could act as ‘information centres’ in which individuals could extract information from conspecifics to assess resource quality by following successful individuals (Ward & Zahavi Citation1973). Under this assumption, birds leaving on foraging trips should depart in temporal synchrony and should preferential go to similar feeding areas. Indeed, we observed that the relative departure headings of birds leaving within 30 seconds of the immediately antecedent bird differed from chance (, P < 0.001) with the 0° relative headings class (i.e. birds leaving in the same direction) being over-represented. Thus, we suggest further studies to determine whether group-foraging kestrels use public information to help them find high-quality foraging patches.
In conclusion, our results showed that although harvesting significantly increases foraging opportunities for breeding Lesser Kestrels through intake rate maximization (close to the colony intake rate exceeds the value in fallows), cereal patches being harvested represent an ephemeral high-quality habitat and its benefits are relatively short-lived as cereals are converted into low-quality stubbles. Additionally, for central-place foragers such as the Lesser Kestrel, intake rate and ultimately patch choice are also determined by the distance between the foraging areas and the colony (Franco et al. Citation2004, García et al. Citation2006, Catry et al. Citation2012). Here, we show that the high intake rate of patches being harvested decline significantly with distance from the colony and at distances exceeding 1500 m, few birds are attracted by the harvesting machines. Finally, our findings are likely to apply to a range of other farmland birds often seen following harvesting machines (e.g. Common Kestrels F. tinnunculus, White Storks Ciconia and Cattle Egrets Bubulcus ibis), including a few species of high conservation concern (e.g. Rollers Coracias garrulus and Montagu's Harriers Circus pygargus).
ACKNOWLEDGEMENTS
Special thanks to Teresa Catry for helping during fieldwork.
FUNDING
IC was funded by a Portuguese post-doctoral grant from Fundação para a Ciência e Tecnologia [grant number SFRH/BPD/76514/2011].
REFERENCES
- Aparicio, J.M. 1990. Método de caza y balance energético diario de Falco naumanni durante el periodo premigratorio. Ardeola 37: 163–178.
- Atkinson, P.W., Buckingham, D. & Morris, A.J. 2004. What factors determine where invertebrate-feeding birds forage in dry agricultural grasslands? Ibis 146: 99–107.
- Atkinson, P.W., Fuller, R.J., Vickery, J.A., Conway, G.J., Tallowin, J.R.B., Smith, R.E.N., Haysom, K.A., Ings, T.C., Asteraki, E.J. & Brown, V.K. 2005. Influence of agricultural management, sward structure and food resources on grassland field use by birds in lowland England. J. Appl. Ecol. 42: 932–942.
- Bijlsma, S., Hagemeijer, E.J.M., Verkley, G.J.M. & Zollinger, R. 1988. Ecological Aspects of Lesser Kestrel in Extremadura (Spain). Katholieke University of Nijmegen; Werkgroep Dieroecologie, Vakgroep Experimentele Zoologie, Nijmegen, the Netherlands.
- Bonal, R. & Aparício, J.M. 2001. Estudio de la utilizacion del hábitat por parte del cernícalo primilla (Falco naumanni) en una colonia de la comarca de La Mancha. In Garcés, J.F. & Corroto M. (eds) Biología y conservación del cernícalo primilla, 53–63. Conserjería de Medio Ambiente, Dirección General de Promoción y Disciplina Ambiental, Grefa, Madrid.
- Bustamante, J. & Negro, J.J. 1994. The post fledgling dependence period of the Lesser Kestrel in southwestern Spain. J. Raptor Res. 28: 158–163.
- Butler, S.J., Bradbury, R.B. & Whittingham, M.J. 2005. Stubble height affects the use of stubble fields by farmland birds. J. Appl. Ecol. 42: 469–476.
- Butler, S.J., Boccaccio, L., Gregory, R., Vorisek, P. & Norris, K. 2010. Quantifying the impact of land-use change to European farmland bird populations. Agr. Ecosyst. Environ. 137: 348–357.
- Calenge, C. 2006. The package adehabitat for the R software: a tool for the analysis of space and habitat use by animals. Ecol. Model. 197: 516–519.
- Catry, I., Dias, M.P., Catry, T., Afanasyev, V., Fox, J., Franco, A.M.A. & Sutherland, W.J. 2011. Individual variation in migratory movements and winter behaviour of Iberian Lesser Kestrels Falco naumanni revealed by geolocators. Ibis 153: 154–164.
- Catry, I., Amano, T., Franco, A.M.A. & Sutherland, W.J. 2012. Influence of spatial and temporal dynamics of agricultural practices on the Lesser Kestrel. J. Appl. Ecol. 49: 99–108.
- Chamberlain, D.E., Fuller, R.J., Bunce, R.G.H., Duckworth, J.C. & Shrubb, M. 2000. Changes in the abundance of farmland birds in relation to the timing of agricultural intensification in England and Wales. J. Appl. Ecol. 37: 771–788.
- Cordeiro, A.P., Ventim, R., Alcazar, R., Catry, I., Franco, A. & Rocha, P. 2005. Seleccão de habitat de alimentação do Peneireiro-das-torres na colónia OU6 da ZPE de CastroVerde. LIFE Project LIFE2002 / NAT / P8481. LPN, Lisbon.
- Delgado, A. & Moreira, F. 2000. Bird assemblages of an Iberian cereal steppe. Agr. Ecosyst. Environ. 78: 65–76.
- Development Core Team R. 2010. R: a language and environment for statistical computing. R Foundation for Statistical Computing, Vienna.
- Devereux, C.L., McKeever, C.U., Benton, T.G. & Whittingham, M.J. 2004. The effect of sward height and drainage on Common Starlings Sturnus vulgaris and Northern Lapwings Vanellus vanellus foraging in grassland habitats. Ibis 146: 115–122.
- Donald, P.F., Pisano, G., Rayment, M.D. & Pain, D.J. 2002. The Common Agricultural Policy, EU enlargement and the conservation of Europe's farmland birds. Agr. Ecosyst. Environ. 89: 167–182.
- Donázar, J.A., Negro, J.J. & Hiraldo, F. 1993. Foraging habitat selection, land-use changes and population decline in the Lesser Kestrel Falco naumanni. J. Appl. Ecol. 30: 515–522.
- Ena, V., Martínez, A. & Thomas, D.H. 1987. Breeding success of the Great Bustard Otis tarda in Zamora Province, Spain in 1984. Ibis 129: 364–370.
- Franco, A.M.A., Catry, I., Sutherland, W.J. & Palmeirim, J. 2004. Do different habitat preference methods produce the same conservation recommendations for Lesser Kestrels? Anim. Conserv. 7: 291–300.
- García, J.T., Morales, M.B., Martinez, J., Iglesias, L., García de la Morena, E., Suárez, F. & Viñuela, J. 2006. Foraging activity and use of space by Lesser Kestrel Falco naumanni in relation to agrarian management in central Spain. Bird Conserv. Int. 16: 83–95.
- Gardiner, T. & Hassal, M. 2009. Does microclimate affect grasshopper populations after cutting of hay in improved grassland? J. Insect. Conserv. 13: 97–102.
- Green, R.E., Tyler, G.A., Stowe, T.J. & Newton, A.V. 1997. A simulation model of the effect of mowing of agricultural grassland on the breeding success of the corncrake (C. crex). J. Zool. Lond. 243: 81–115.
- Grüebler, M.U., Schuler, H., Müller, M., Spaar, R., Horch, P. & Naef-Daenzer, B. 2008. Female biased mortality caused by anthropogenic nest loss contributes to population decline and adult sex ratio of a meadow bird. Biol. Conserv. 141: 3040–3049.
- Guido, M. & Gianella, D. 2001. Distribution patterns of four Orthoptera species in relation to microhabitat heterogeneity in an ecotonal area. Acta Oecol. 22: 175–185.
- Humbert, J.Y., Ghazoul, J., Richner, N. & Walter, T. 2010. Hay harvesting causes high orthopteran mortality. Agr. Ecossyst. Environ. 139: 522–527.
- Humbert, J.Y., Ghazoul, J., Richner, N. & Walter, T. 2012. Uncut grass refuges mitigate the impact of mechanical meadow harvesting on orthopterans. Biol. Conserv. 152: 96–101.
- Johst, K., Brandl, R. & Pfeifer, R. 2001. Foraging in a patchy and dynamic landscape: human land us and the White stork. Ecol. Appl. 11: 60–69.
- Kleijn, D., Schekkerman, H., Dimmers, W.J., Van Kats, R.J.M., Melman, D. & Teunissen, W.A. 2010. Adverse effects of agricultural intensification and climate change on breeding habitat quality of Blacktailed Godwits Limosa l. limosa in the Netherlands. Ibis 152: 475–486.
- Manly, B.F.J., McDonald, L.L. & Thomas, D.L. 1993. Resource Selection by Animals. Chapman and Hall, London.
- Milner, B.T., Johnston, K. & Forman, R.T.T. 1989. Scale-dependent proximity of wildlife habitat in a spatially-neutral Bayesian model. Landsc. Ecol. 2: 101–110.
- Moreira, F. 1999. Relationships between vegetation structure and breeding bird densities in fallow cereal steppes in Castro Verde, Portugal. Bird Study 46: 309–318.
- Morris, M.G. 2000. The effects of structure and its dynamics on the ecology and conservation of arthropods in British grasslands. Biol. Conserv. 95: 129–142.
- Newton, I. 2004. The recent declines of farmland bird populations in Britain: an appraisal of causal factors and conservation actions. Ibis 146: 579–600.
- Oppermann, R., Handwerk, J., Holsten, M. & Krismann, A. 2000. Naturverträgliche Mähtechnik für das Feuchtgrünland. Voruntersuchung für das E & E – Vorhaben. ILN Singen, Bonn.
- Palacín, C., Alonso, J.C., Martín, C.A. & Alonso, J.A. 2011. The importance of traditional farmland areas for steppe birds: a case study of migrant female Great Bustards Otis tarda in Spain. Ibis 154: 85–95.
- Peggie, C.T., Garratt, C.M. & Whittingham, M.J. 2011. Creating ephemeral resources: how long do the beneficial effects of grass cutting last for birds? Bird Study 58: 390–398.
- Pilon, A. 2005. The effect of vegetation type and mowing on the hunting success of male Montagu's Harriers in east Groningen, the Netherlands. Master Thesis, University of Groningen, Netherlands.
- Rodríguez, C., Tapia, L., Kieny, F. & Bustamante, J. 2010. Temporal changes in Lesser Kestrel (Falco naumanni) diet during the breeding season in southern Spain. J. Raptor Res. 44: 120–128.
- Rodríguez, C., Tapia, L., Ribeiro, E. & Bustamante, J. 2013. Crop vegetation structure is more important than crop type in determining where Lesser Kestrels forage. Bird Conserv. Int. doi:10.1017/S0959270913000129
- Savage, R.E. 1931. The relation between the feeding of the herring off the east coast of England and the plankton of the surrounding waters. Fishery Invest. Lond. Ser. 2. 12: 1–88.
- Shrubb, M. 1980. Farming influences on the food and hunting of Kestrels. Bird Study 27: 109–115.
- Stephens, D.W. & Krebs, J.R. 1986. Foraging Theory. Princeton University Press, Princeton, NJ.
- Suárez, F., Naveso, M.A. & De Juana, E. 1997. Farming in the drylands of Spain: birds of the pseudosteppes. In Pain, D.J. & Pienkowski, M.W. (eds) Farming and Birds in Europe, The Common Agricultural Policy and its Implications for Bird Conservation, 297–330. Academic Press, San Diego.
- Tella, J.L., Forero, M.G., Hiraldo, F. & Donázar, J.A. 1998. Conflicts between Lesser Kestrel conservation and European agricultural policies as identified by habitat use analyses. Conserv. Biol. 12: 593–604.
- Tews, J., Bert, D.G. & Mineau, P. 2013. Estimated mortality of selected migratory bird species from mowing and other mechanical operations in Canadian agriculture. Avian Conserv. Ecol. 8: 8.
- Tichit, M., Renault, O. & Potter, T. 2005. Grazing regime as a tool to assess positive side effects of livestock farming systems on wading birds. Livest. Prod. Sci. 96: 109–117.
- Tucker, G.M. & Evans, M.I. 1997. Habitats for Birds in Europe: A Conservation Strategy for the Wider Environment. BirdLife International, Cambridge.
- Tucker, G.M. & Heath, M.F. 1994. Birds in Europe: Their Conservation Status. BirdLife International, Cambridge.
- Ursúa, E., Serrano, D. & Tella, J.L. 2005. Does land irrigation actually reduce foraging habitat for breeding Lesser Kestrels? The role of crop types. Biol. Conserv. 122: 643–648.
- Vickery, J.A., Tallowin, J.R., Feber, R.E., Asteraki, E.J., Atkinson, P.W., Fuller, R.J. & Brown, V.K. 2001. The management of lowland neutral grasslands in Britain: effects of agricultural practices on birds and their food resources. J. Appl. Ecol. 38: 647–664.
- Ward, P. & Zahavi, A. 1973. The importance of assemblages of birds as ‘information centres’ for food finding. Ibis 115: 517–534.
- Whittingham, M.J. & Devereux, C.L. 2008. Changing grass height alters foraging site selection by wintering farmland birds. Basic Appl. Ecol. 9: 779–788.