Abstract
Capsule Features of the breeding population and temporary settlement area influence the behaviour of Eagle Owls Bubo bubo prospecting for breeding sites during natal dispersal.
Aims To understand how prospecting behaviour during natal dispersal is affected by (i) the main characteristics of the breeding and dispersing portions of the population and (ii) main prey availability.
Methods We explored the ten-year dynamics and characteristics of radio-tagged breeders and dispersers of an Eagle Owl population.
Results During the first years following natal dispersal there was little prospecting behaviour of nesting sites and birds remained mainly within non-breeding settlement areas, bordering the sector occupied by the breeding population. Settlement areas had an abundant food supply, and low intraspecific competition and mortality. We suggest that these features of the settlement areas may reduce the willingness of individuals to search for breeding sites and may have the potential to impact on the viability of breeding populations.
Conclusion From a conservation perspective, the lengthy use of the temporary settlement areas by juvenile Eagle Owls suggests that the sites should be considered as important as the breeding areas when planning conservation strategies. Reducing juvenile mortality in settlement areas may represent an overlooked conservation strategy for long-lived species and may have a crucial effect on the viability some animal populations.
Natal dispersal (hereafter termed ‘dispersal’) refers to the movements of juveniles from their birthplace to their first breeding location. An individual's perception of the physical and social environment during the different stages of dispersal (i.e. starting, wandering and settlement; Clobert et al. Citation2009), as well as the subsequent behavioural decision to settle in a given place, influence dispersal patterns and the resulting population properties (Delibes et al. Citation2001a, Penteriani et al. Citation2005a, Citation2005b, Delgado et al. Citation2009, Penteriani et al. Citation2011, Soutullo et al. Citation2013). Therefore, studies of natal dispersal patterns and dispersal behaviour are crucial to help us understand population dynamics within breeding areas (Penteriani et al. Citation2005a, Citation2005b, Citation2008, Citation2011, Soutullo et al. Citation2008).
It is not uncommon for natural selection acting at the individual level to result in negative effects on the population as a whole (Rankin et al. Citation2007, Delgado et al. Citation2011): individuals cannot always anticipate the consequences of their behaviours for their survival and reproductive success at some later point in time (Hutto Citation1985, Robertson & Hutto Citation2006). However, because empirical data on this issue have rarely been presented, negative population consequences of individual behaviours may be more common than generally expected or observed, i.e. some conditions may preclude naturally selected behaviours from promoting population growth or maintenance (Rankin et al. Citation2007, Delgado et al. Citation2011). The idea that individual choices and strategies can promote and facilitate a decline at a population level has rarely been observed in natural systems (Rankin & López-Sepulcre Citation2005, Delgado et al. Citation2011). In addition, because individual strategies during dispersal and the common good may diverge, we might expect that natural selection acting at the individual level should not necessarily produce optimal dispersal behaviour at the population level (Delgado et al. Citation2011).
Here, we illustrate how prospecting behaviour by dispersing juvenile Eagle Owls Bubo bubo may be constrained by a combination of some intrinsic properties of the population. In particular, a high density of breeders in the reproductive sector of a population (i.e. the geographical area where breeding occurs) and high availability of food in the dispersal, non-breeding settlement areas may interrupt or at least alter the flux of individuals from dispersal to breeding areas. We begin by briefly reviewing the relevant characteristics and spatial dynamics of the study population and, then, we suggest how dispersal with low recruitment may emerge and discuss its consequences for the population.
METHODS
The study system
From 2002 to 2012, we studied both the dispersing and breeding portions of a high-density population (approximately 40 pairs/100 km2; Penteriani & Delgado Citation2011) of Eagle Owls in the Sierra Norte of Seville, Spain (37°30ʹN, 06°03ʹW). We checked the progress of the breeding cycle at 36 breeding sites, where a total of 132 breeding attempts were monitored. From 24 of these sites, we trapped and radio-tagged 34 breeding individuals (24 males and 10 females; more details in Campioni et al. Citation2013), and from 21 of the sites, we radio-tagged 74 juveniles (45 males and 29 females). The mean (±SD) distance between the nearest neighbouring breeding pairs was 982 ± 491 m (range = 250–2729 m). Laying dates ranged from 24 December to 8 April, and the mean number (±SD) of fledglings was 2.2 ± 1.0 per brood (range = 1–4 chicks). Although breeders generally showed high site fidelity (i.e. they occupied the same breeding site during many years or their whole life), we recorded 9 cases of breeding dispersal (5 males and 4 females that shifted from one breeding site to another), corresponding to the 26.5% of the radio-tagged breeders. Breeding dispersal distances were relatively short (mean = 3385 ± 3747 m; range = 633–10 134 m). Breeders and dispersers of the population occupied contiguous areas (Penteriani & Delgado Citation2012, & 2).
Figure 1. Dispersal routes of radio-tagged juvenile Eagle Owls. Nesting sites are represented by black dots, except for those that have been occupied by a juvenile at the beginning of dispersal (grey dots; see text for more details). Grey lines represent individuals that encountered an available breeding site before entering the common settlement area and began to reproduce there. Black lines show the dispersal paths of the other owls, which ended in the common settlement area. Analyses showed that occupancy of a breeding site by a disperser was generally random; directions of dispersal were the only factors slightly favouring the entrance of a juvenile as a new breeder (see Results). The juveniles’ paths within the breeding area show their movements at the beginning of dispersal, when they were still living in the breeding area.
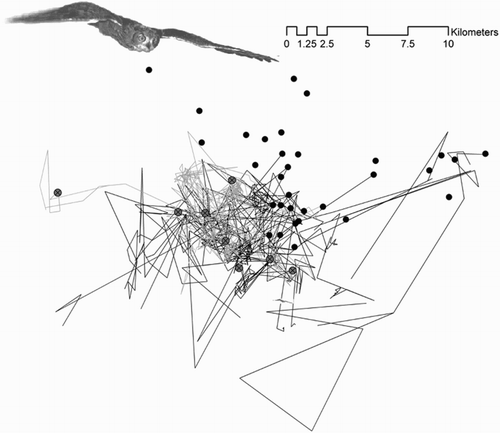
Figure 2. Home ranges of breeders (dark grey) and dispersers (light grey) obtained during continuous radio-tracking sessions (i.e. the following of focal individuals from sunset to sunrise; more details in Methods). Although the breeding and dispersal areas are very close to each other, there is a clear spatial separation between the two fractions of the population. As a consequence of this spatial segregation, dispersers should have not been able to receive information on the availability of reproductive opportunities within the breeding area without active prospecting (which they never did, see Results). Spatial segregation may be the result of breeder's territorial behaviour, which make it safer for dispersers to settle in areas empty of breeders where territoriality and intraspecific competition is lower.
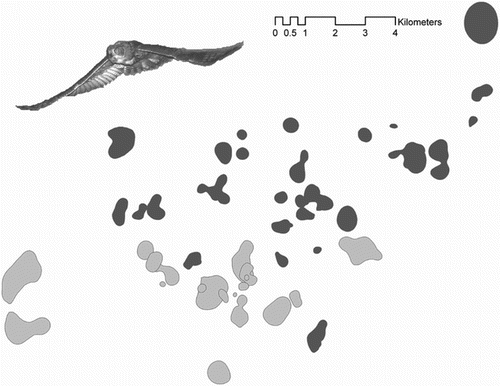
The radio-tracking protocol
Owls were trapped and marked under the Junta de Andalucía–Consejería de Medio Ambiente permit SCFFSAFR/ GGG RS-260 / 02 and SCFFS-AFR/CMM RS-1904 / 02. Each individual was fitted with a 30 g radio transmitter (Biotrack Ltd) secured with a Teflon-ribbon backpack harness. The mass of the backpack was less than 3% of the mass of the smallest adult male (1550 g; mean ± SE = 1667 ± 105 g) in our population (for details on radio-tracking procedures, see Delgado et al. Citation2010, Penteriani & Delgado Citation2012, Campioni et al. Citation2013). By following radio-tagged individuals, we collected detailed information on both dispersal (Delgado et al. Citation2010, Penteriani & Delgado Citation2011) and the breeders’ home-range behaviour (Campioni et al. Citation2013). We followed individual breeders year-round from 1 hour before sunset to 1 hour after sunrise for a total of 3333 hours, during 296 continuous radio-tracking sessions (5298 locations; mean number of locations per radio-tracking session ± SD = 17.2 ± 5.2). Dispersers were radio-tracked following the same procedure as the breeders, except that tagged individuals were tracked at two different temporal scales: nightly (from one hour before sunset to one hour after sunrise, individuals were followed on a rotation basis, one individual per night) and weekly (mainly to record distances of dispersal, the location of each of the tagged juveniles was recorded at its diurnal roosting site). At the nightly scale (n = 163 tracking nights for a total of 2010 hours; mean number of locations per radio-tracking session ± SD = 18.0 ± 4.6), we recorded 3196 locations. For the weekly scale tracking, each owl was located on a weekly basis at its daytime roosting site (total number of locations = 1189). Tagged individuals were followed up to 2.5 years, i.e. until the radio-tagged owls died or the battery of the transmitter failed (Delgado et al. Citation2010, Penteriani & Delgado Citation2012).
Comparing rabbit density between breeding and settlement areas
As the European Rabbit Oryctolagus cuniculus is the main prey of Eagle Owls in the study population (mean biomass percentage of rabbit in the diet = 62.0 ± 19.1%), relative rabbit abundance was estimated in the home ranges of 24 breeders and 13 dispersers using rabbit latrine counts (details in Campioni et al. Citation2013). The survey was conducted in 2009 from the beginning of March to the beginning of May: this period corresponds to the nestling and fledging/post-fledging dependence periods of Eagle Owls, when it is expected that parents exhibit the highest hunting effort. Rabbit density over the years can be considered relatively stable in our study area: rabbit management and frequent releases by hunting societies inside our study area have created extremely favourable and steady trophic conditions (Penteriani & Delgado, unpubl. data).
Statistical analyses
To test whether some variables that affected general dispersal patterns (Delgado et al. Citation2010, Penteriani et al. Citation2011) also influenced whether a dispersing juvenile entered the population as a breeder or not (binomial response variable), we assumed a binomial error structure and used a generalized linear mixed-effects model fitted by the Laplace approximation. This method was used to assess whether the event of occupying an empty nest was dependent on any of the following explanatory variables: (i) sex, (ii) age, (iii) body condition and (iv) directions of dispersal. Body condition was estimated by a reduced major axis regression (Green Citation2001), using log of both body mass (to the nearest 10 g, with 1 kg Pesola scales) and wing length (using a digital calliper, ±0.1 mm). Higher values of body condition represent higher-quality individuals (Green Citation2001). Directions of dispersal were calculated as the direction of net movement from the natal site to the settlement area (angles were rounded to the nearest 30°; Penteriani & Delgado Citation2011). Because we had repeated measures for the same nests over multiple years, we considered birthplace nested in year as a random effect. Model simplification was performed by the backward selection of variables from the full model, and models were compared using likelihood-ratio tests until a minimal adequate model was obtained. For this generalized linear mixed-effects model, we used a slightly different subsample of the data, representing those individuals for which it was possible to collect the specific information sought (i.e. the 12 radio-tagged juveniles that entered an empty breeding site as new breeders vs. 46 dispersers that went to the common settlement area). Statistical analyses were performed in R 2.10.1 statistical software (R Development Core Team Citation2009) with the lme4 (Bates & Maechler Citation2009) package.
RESULTS
Dynamics of the breeding population
The breeding population was characterized by two primary dynamics: (1) dispersers were recruited from within the breeding population only when their dispersal paths crossed an empty breeding site on the edge of the population (see Dispersers: natal dispersal, temporary settlement areas and first breeding) and (2) in the ten cases of breeder replacement recorded in the core of the population, new breeders were either individuals that had previously been trapped at another breeding site (i.e. breeding dispersal) or were relatively old individuals (mean age of trapped breeders = 4 ± 1 years). The mean age was lower at the edges of the population, where dispersers entered as new breeders. Although we marked a total of 185 chicks, using both radio-tags (n = 74) and rings (n = 111), between 2002 and 2009, we never found any tagged individuals breeding in the core of the population. During the study period, at three nesting sites located in the core of the population (out of the 36 regularly checked for breeding, i.e. 8.3%), the owners dispersed or died, and these sites were never filled by new breeders, despite the high productivity of the breeding population generating a large pool of dispersers (see below and Penteriani & Delgado Citation2012). We recorded the cause of mortality for eight breeders, which included shooting (five individuals, two males and three females), intraspecific killing (two males) and fence collision (one male).
Dispersers: natal dispersal, temporary settlement areas and first breeding
Of the radio-tagged dispersers, 19.0% either lost their transmitters or had them fail. We found dispersal distances to range from 1.5 to 34.3 km (mean ± SD = 6.0 ± 4.2 km). In fact, most of the juveniles found a stable settlement close to the natal population, living as floating individuals while they were being tracked. Here, we define floaters as the pool of dispersing individuals independent of age (see also Penteriani et al. Citation2011) given that Eagle Owls are sexually mature at less than 1 year old, and dispersing owls ‘floated’ in the vicinity of the breeding population during dispersal (Delgado & Penteriani Citation2008, Delgado et al. Citation2010).
When in their settlement areas (i.e. zones occupied by dispersing individuals until they die or become owners of a breeding site, Penteriani et al. Citation2011), dispersing owls: (i) showed home ranges (Penteriani & Delgado Citation2012) but never bred there because of the lack of suitable nesting places; and (ii) did not prospect for potential breeding sites, although the natal area was just a few kilometres away (& 2). Most of the radio-tagged juveniles settled in the same settlement area (Delgado et al. Citation2010, Penteriani & Delgado Citation2012). Along with the evidence that juveniles were never recorded prospecting (i.e. dispersers remained floating near the breeding area), the recorded patterns of nesting site occupancy are remarkable. First, 12 of the 74 radio-tagged juveniles (16.2%, 8 males and 4 females) that entered an empty breeding site as a new breeder did so in the first few months after the start of dispersal, while en route to the common temporary settlement area. In fact, some radio-tagged owls began to breed successfully when less than one year old. Second, all dispersers that became new breeders originated from one-third (7 of 21) of the nesting sites where we radio-tagged chicks, all of which were located in the highest density core of the population (see also ). Third, all re-occupied nesting sites were located along the routes linking the birthplaces of the tagged individuals with the common settlement area. Finally, these new nesting sites were always located on the edge of the breeding population, where density is somewhat lower than in the core. In the context of breeder/disperser dynamics, it is notable that, despite the extremely high density of individuals, when a breeding site became vacant, its re-occupancy (if any) was delayed by several years if it was not crossed by a new disperser. This phenomenon appeared to be independent of the quality of the breeding site (based on the fecundity of their owners) because (a) the breeding population was characterized by a predominance of high-quality nesting sites (Delgado et al. Citation2013) and (b) sites in the core of the population, where we recorded the best breeding performances, were not occupied first (and some were still empty more than five years after they became available for new breeders). The finding of a breeding place during dispersal was only slightly affected (z = 1.92, P = 0.055) by the direction followed by the individuals during dispersal (estimate ± SE = 0.019 ± 0.009; intercept: β = 6.344 ± 2.589; P = 0.014).
Finally, the mean age of death calculated for a sample of 21 out of the 74 radio-tagged dispersers was 357 ± 167 days (range = 161–678 days), which indicates that at least 30% of the dispersers died during their first year of life while en route to the temporary settlement area (19.0%) or while they were already there (11.7%). In fact, individuals shifted from the wandering to the settlement phase of dispersal in the middle of March (mean dispersal age at the settlement phase = 395 ± 110 days; range = 181–640 days; Delgado et al. Citation2009, Citation2010). The mortality rate of dispersers (44.6%, 18 males, 11 females and 4 individuals of unknown sex) was quite similar to that of breeders (35.29%, 8 males and 4 females). Such a minimal difference in mortality may be due to the stochasticity of deaths in the study system: the primary cause of mortality for the 12 dispersers was shooting (6 males and 3 females), followed by car collision (1 male), electrocution (1 female) and starvation (1).
Rabbit density: breeding versus settlement areas
We counted a total of 3440 latrines for breeders and 693 for dispersers, corresponding to a mean density (±SE) of 21 ± 12 km−1 (range = 8–46 km−1) for a breeder's home range and a mean density (±SE) of 24 ± 17 km−1 (range = 3–48 km−1) for a disperser's home range. That is, rabbit density can be considered equal in both sectors of the population.
DISCUSSION
The dispersal settlement area is represented by an optimal area that might be difficult to leave for young individuals: food is abundant, intraspecific competition seems to be low (Penteriani & Delgado Citation2012) and mortality is not any higher than in the breeding area. That is, individuals settle in very attractive patches regardless of whether they are the ‘best’ in terms of population growth rates and persistence (Battin Citation2004).
Two contrasting explanations may help to elucidate the recorded lack of prospecting behaviour during dispersal. Firstly, we can hypothesise that those juveniles that reach such settlement areas are ‘trapped’ there. Evolutionary traps occur when individuals make maladaptive choices based on apparently reliable environmental cues (i.e. maladaptive behavioural or life-history choices made despite the availability of higher-quality options, hereafter termed ‘trap’; Schlaepfer et al. Citation2002). The misleading mechanisms and cues associated with traps ensnare individuals in situations or places that reduce their lifetime fitness or survival (Schlaepfer et al. Citation2002). The requisite condition for a trap (that given a suite of choices, an individual selects a place in which fitness, i.e. ability to reproduce, is very low) could fit well with our study system. The dispersal trap acts on the behaviour of individuals, delaying or preventing (if dispersers die before leaving the settlement area) conditions that would increase their fitness and the stability of the whole population. Here, the prediction of Kokko & Sutherland (Citation2001) that natal philopatry provides a highly efficient mechanism for trap avoidance may not be sufficient if individuals continue floating close to the breeding population (increasing the probability of philopatry) but do not prospect for breeding opportunities.
One contrasting explanation for the dispersal patterns we observed may involve the non-mutually exclusive effects of (i) queuing for available/best breeding sites (Kokko & Sutherland Citation1998), which may derive from the saturation of breeding sites (Ekman et al. Citation1999) and (ii) delayed reproduction, which is important for long-lived individuals that may have greater lifetime reproductive success than individuals that breed immediately (Cooper et al. Citation2009, Millon et al. Citation2010). Indeed, it is possible that the young birds that are apparently ‘trapped’ in areas where they cannot breed may simply be delaying prospecting and breeding until such time as they have gained sufficient experience and body condition (Zack & Stutchbury Citation1992). It might well be advantageous for dispersers to wait a number of years, gaining body mass and experience, before attempting to prospect for a nest site, particularly if prospecting leads to antagonistic interactions with territory holders or otherwise risks survival. However, we have recorded some individuals that occupied the first empty nesting site they came across during the early stages of dispersal and started breeding less than one year after hatching. Under this scenario it is difficult to understand why individuals ready to breed and willing to accept the first breeding place they encounter should wait several years before they start checking the breeding population in an apparently ‘conscious’ queuing strategy, as this strategy leads to delayed reproduction. Moreover, delayed reproduction is generally associated with temporal variability in environmental conditions affecting the fitness expectations of commencing reproduction early in life (Tuljapurkar Citation1990, Cooper et al. Citation2009, Millon et al. Citation2010), which does not apply to our population. Additionally, prospecting behaviour is implicit in the concept of queuing given that available/best breeding sites cannot be found if dispersers are trapped in their dispersal settlement areas and never prospect for vacant breeding sites.
However, we cannot discard the possibility that, later in life, some of the survivors inhabiting the settlement area may recommence dispersal towards potential breeding sites, as observed for other large raptors (González et al. Citation2006, Urios et al. Citation2007, Cadahía et al. Citation2009, Citation2010, Muñiz-López et al. Citation2012). Because of the limited life of the transmitter batteries, we have not been able to document this phenomenon, but if it occurs, it does not reduce the risks represented by the mechanism highlighted here. By delaying prospecting for empty breeding sites and available mates, individuals increase their risk of dying before leaving settlement areas. That is, lack of prospecting behaviour may slow the dispersal process, such that the prospecting for a breeding area is less vigorous and occurs later in an individual's lifetime. The general tendency to leave empty nesting sites unoccupied because of the lack of breeding site prospecting has the potential to place animal populations at risk (see for a detailed description of the mechanism). Thus, to prevent potential negative consequences at the population level due to this long settlement of dispersers in their temporary settlement areas, this latter should be considered, from a conservation point of view, as important as breeding areas. Indeed, reducing juvenile mortality in their settlement areas during dispersal may represent a crucial conservation strategy for long-lived species and may have an important effect on the viability of the reproductive sectors of an animal population.
Figure 3. Potential alterations to the natal dispersal dynamics determined by the emergence of a trap in the disperser's settlement areas. (A1) The breeding population (black square) is saturated because of (i) immigration from other populations, (ii) breeder redistribution within the population (i.e. breeding dispersal) and (iii) entrance of dispersing individuals from both the wandering (dark grey square) and the temporary settlement (grey square) areas (see details of the dispersal matrix in the main text). (A2) If the temporary settlement areas used during dispersal form a trap that prevents disperser prospecting for breeding opportunities, the immigration flux from the dispersal to the breeding areas stops. As a consequence, the breeding population decreases due to the loss of a portion of future potential breeders, which are now trapped in higher numbers within the dispersal matrix and do not prospect regularly for vacancies in the breeding area. Actually, after the emergence of the trap, immigration from other populations and from those individuals already settled ceases, and wandering individuals are the only immigration fluxes that breeders receive. (B) An interesting link exists between the trap mechanism we described and the attractive sink (or deceptive source) concept developed by Delibes et al. (Citation2001b). Traps in the settlement areas of dispersers may produce temporal changes within the breeding – settlement areas system that resemble the effects of an attractive sink on source populations, where the settlement areas/attractive sinks absorb most of the potential breeders from the breeding sites/source.
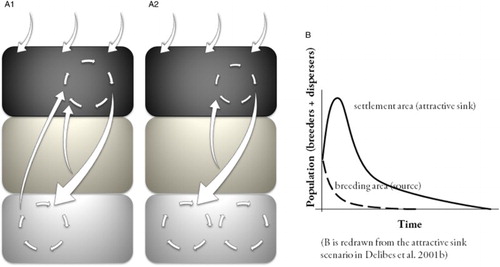
ACKNOWLEDGEMENTS
We thank L. Campioni, R. Lourenço, C. Bettega and P. Bartolommei for their valuable help with fieldwork. We are grateful to R. Alonso for his help with ageing Eagle Owls. The suggestions of two anonymous referees improved the first draft.
Additional information
Funding
References
- Bates, D. & Maechler, M. 2009. lme4: Linear mixed-effects models using S4 classes. Version 0.999375-31. http://lme4.r-forge.r-project.org/.
- Battin, J. 2004. When good animals love bad habitats: ecological traps and the conservation of animal populations. Conserv. Biol. 18: 1482–1491. doi: 10.1111/j.1523-1739.2004.00417.x
- Cadahía, L., López-Lópz, P., Urios, V., Soutullo, A. & Negro, J.J. 2009. Natal dispersal and recruitment of two Bonelli's Eagles Aquila fasciata: a four-year satellite tracking study. Acta Ornithol. 44: 193–198. doi: 10.3161/000164509X482777
- Cadahía, L., López-Lópz, P., Urios, V. & Negro, J.J. 2010. Satellite telemetry reveals individual variation in juvenile Bonelli's Eagle dispersal areas. Eur. J. Wild. Res. 56: 923–930. doi: 10.1007/s10344-010-0391-z
- Campioni, L., Delgado, M. M., Lourenço, R., Bastianelli, G., Fernández, N. & Penteriani, V. 2013. Individual and spatio-temporal variations in the home range behaviour of a long-lived, territorial species. Oecologia 172: 371–385. doi: 10.1007/s00442-012-2493-7
- Clobert, J., Le Galliard, J.-F., Cote, J., Meylan, S. & Massot, M. 2009. Informed dispersal, heterogeneity in animal dispersal syndromes and dynamics of spatially structures populations. Ecol. Lett. 12: 197–209. doi: 10.1111/j.1461-0248.2008.01267.x
- Cooper, N.W., Murphy, M.T., Redmond, L.J. & Dolan, A.C. 2009. Density-dependent age at first reproduction in the eastern kingbird. Oikos 118: 413–419. doi: 10.1111/j.1600-0706.2008.16997.x
- Delgado, M.M. & Penteriani, V. 2008. Behavioral states help translate dispersal movements into spatial distribution patterns of floaters. Am. Nat. 172: 475–485. doi: 10.1086/590964
- Delgado, M.M., Penteriani, V., Nams, V.O. & Campioni, L. 2009. Changes of movement patterns from early dispersal to settlement. Behav. Ecol. Sociobiol. 64: 35–43. doi: 10.1007/s00265-009-0815-5
- Delgado, M.M., Penteriani, V., Revilla, E. & Nams, V.O. 2010. The effect of phenotypic traits and external cues on natal dispersal movements. J. Anim. Ecol. 79: 620–632. doi: 10.1111/j.1365-2656.2009.01655.x
- Delgado, M.M., Ratikainen, I.I. & Kokko, H. 2011. Inertia: the discrepancy between individual and population-level benefit in dispersal and prospecting behaviour. Biol. Rev. 86: 717–732. doi: 10.1111/j.1469-185X.2010.00167.x
- Delgado, M.M., Caferri, E., Méndez, M., Godoy, J.A., Campioni, L. & Penteriani, V. 2013. Population characteristics may reduce the levels of individual call identity. PLoS ONE 8: e77557. doi: 10.1371/journal.pone.0077557
- Delibes, M., Ferreras, P. & Gaona, P. 2001a. Attractive sinks, or how individual behavioural decisions determine source-sink dynamics. Ecol. Lett. 4: 401–403. doi: 10.1046/j.1461-0248.2001.00254.x
- Delibes, M., Gaona, P. & Ferreras, P. 2001b. Effects of an attractive sink leading into maladaptive habitat selection. Am. Nat. 158: 277–285. doi: 10.1086/321319
- Ekman, J., Bylin, A. & Tegelström, H. 1999. Increased lifetime reproductive success for Siberian jay (Perisoreus infaustus) males with delayed dispersal. Proc. R. Soc. Lond. B 266: 911–915. doi: 10.1098/rspb.1999.0723
- González, L.M., Oria, J., Margalida, A., Sánchez, R., Prada, L., Caldera, J., Aranda, A. & Molina, J.I. 2006. Effective natal dispersal and age of maturity in the threatened Spanish Imperial Eagle Aquila adalberti: conservation implications. Bird Study 53: 285–293. doi: 10.1080/00063650609461444
- Green, A. 2001. Mass/length residuals: measures of body condition or generators of spurious results? Ecology 82: 1473–1483. doi: 10.1890/0012-9658(2001)082[1473:MLRMOB]2.0.CO;2
- Hutto, R. 1985. Habitat selection by nonbreeding migratory land birds. In Cody, M. (ed.) Habitat Selection in Birds, 455–476. Academic Press, New York, NY.
- Kokko, H. & Sutherland, W.J. 1998. Optimal floating and queuing strategies: consequences for density dependence and habitat loss. Am. Nat. 152: 354–366. doi: 10.1086/286174
- Kokko, H. & Sutherland, W.J. 2001. Ecological traps in changing environments: ecological and evolutionary consequences of a behaviourally mediated Allee effect. Evol. Ecol. Res. 3: 537–551.
- Millon, A., Petty, S.J. & Lambin, X. 2010. Pulsed resources affect the timing of first breeding and lifetime reproductive success of tawny owls. J. Anim. Ecol. 79: 426–435. doi: 10.1111/j.1365-2656.2009.01637.x
- Muñiz-López, R., Limiñana, R., Cortés, G.D. & Urios, V. 2012. Movements of Harpy Eagles Harpia harpyja during their first two years after hatching. Bird Study 59: 509–514. doi: 10.1080/00063657.2012.722190
- Penteriani, V. & Delgado, M. M. 2011. Birthplace-dependent dispersal: are directions of natal dispersal determined a priori? Ecography 34: 729–737. doi: 10.1111/j.1600-0587.2010.06773.x
- Penteriani, V. & Delgado, M. M. 2012. There is a limbo under the moon: what social interactions tell us about the floaters’ underworld. Behav. Ecol. Sociobiol. 66: 317–327. doi: 10.1007/s00265-011-1279-y
- Penteriani, V., Otalora, F., Sergio, F. & Ferrer, M. 2005a. Environmental stochasticity in dispersal areas can explain the ‘mysterious’ disappearance of breeding populations. Proc. R. Soc. Lond. B. 272: 1265–1269. doi: 10.1098/rspb.2005.3075
- Penteriani, V., Otalora, F. & Ferrer, M. 2005b. Floater survival affects population persistence: the role of prey availability and environmental stochasticity. Oikos 108: 523–534. doi: 10.1111/j.0030-1299.2005.13514.x
- Penteriani, V., Otalora, F. & Ferrer, M. 2008. Floater mortality within settlement areas can explain the Allee effect in breeding populations. Ecol. Modell. 213: 98–104. doi: 10.1016/j.ecolmodel.2007.11.009
- Penteriani, V., Ferrer, M. & Delgado, M. M. 2011. Floater strategies and dynamics in birds, and their importance in conservation biology: towards an understanding of nonbreeders in avian populations. Anim. Conserv. 14: 233–241. doi: 10.1111/j.1469-1795.2010.00433.x
- R Development Core Team. 2009. R: A Language and Environment for Statistical Computing. R Foundation for Statistical Computing, Vienna. www.R-project.org.
- Rankin, D.J. & López-Sepulcre, A. 2005. Can adaptation lead to extinction? Oikos 111: 616–619. doi: 10.1111/j.1600-0706.2005.14541.x
- Rankin, D.J., López-Sepulcre, A., Foster, K.R. & Kokko, H. 2007. Species-level selection reduces selfishness through competitive exclusion. J. Evol. Biol. 20: 1459–1468. doi: 10.1111/j.1420-9101.2007.01337.x
- Robertson, B.A. & Hutto, R. L. 2006. A framework for understanding ecological traps and an evaluation of existing evidence. Ecology 87: 1075–1085. doi: 10.1890/0012-9658(2006)87[1075:AFFUET]2.0.CO;2
- Schlaepfer, M.A., Runge, M.C. & Sherman, P. W. 2002. Ecological and evolutionary traps. Trends Ecol. Evol. 17: 474–480. doi: 10.1016/S0169-5347(02)02580-6
- Soutullo, A., López-López, P. & Urios, V. 2008. Incorporating spatial structure and stochasticity in endangered Bonelli's eagle's population models: implications for conservation and management. Biol. Conserv. 141: 1013–1020. doi: 10.1016/j.biocon.2008.01.011
- Soutullo, A., López-López, P., Cortés, G.D., Urios, V. & Ferrer, M. 2013. Exploring juvenile golden eagles’ dispersal movements at two different temporal scales. Ethol. Ecol. Evol. 25: 117–128. doi: 10.1080/03949370.2012.742463
- Tuljapurkar, S. 1990. Delayed reproduction and fitness in variable environments. Proc. Nat. Acad. Sci. USA 87: 1139–1143. doi: 10.1073/pnas.87.3.1139
- Urios, V., Soutullo, A., López-López, P., Cadahia, L., Limiñana, R. & Ferrer, M. 2007. The first case of successful breeding of a Golden Eagle Aquila chrysaetos tracked from birth by satellite telemetry. Acta Ornithol. 42: 205–209. doi: 10.3161/068.042.0205
- Zack, S. & Stutchbury, B. J. 1992. Delayed breeding in avian social systems: the role of territory quality and ‘floater’ tactics. Behaviour 123: 194–219. doi: 10.1163/156853992X00020