ABSTRACT
Capsule: Chick growth rates was sex-dependent in an Atlantic Yellow-legged Gull Larus michahellis population.
Aims: To describe the growth rate of Yellow-legged Gull chicks and sex-associated variations, and obtain a discriminant function to sex them using morphological data.
Methods: Fifty-two Yellow-legged Gull chicks from a colony in northern Iberia were measured for body mass, head and bill length, tarsus length and three other bill-length associated variables, from the hatching date up to the age of 36 days. Birds were sexed using DNA analysis.
Results: Using logistic models, chick growth rates was observed to be similar between the sexes, while the asymptote was higher among males for the majority of the variables. Discriminant analyses showed that the variable head+bill was very reliable to predict the sex of >80% of chicks at an early developmental stage, and 100% of chicks if combined with tarsus length and two more bill-length associated variables at the age of 35 days.
Conclusions: This is the first study using a discriminant analysis to sex Yellow-legged Gull chicks, and also the first to describe the growth function for the species. The growth rate varied between sexes because males showed higher asymptote values within the growth function.
In bird species with sexual dimorphism, the two sexes often reach their fledging mass at the same age (Kalmbach & Benito Citation2007). For that, the smaller sex must grow at a faster rate than the larger sex, especially if the two sexes fledge at similar masses. This is attributed to the fact that the smaller sex is at a disadvantage in relation to its siblings and, therefore, a faster growth rate allows it to have the chance to compete with success for resources such as food provisioned by parents (Bortolotti Citation1986). Accordingly, in dimorphic birds with high intra-brood competition, such as many seabirds (Amundsen & Stockland Citation1988, Velando et al. Citation2000, Kim & Monaghan Citation2006), the smaller sex is expected to grow at a faster rate than the larger one.
Knowing the sex is an important requirement in many studies, because many life history processes and behaviours are sex-dependent (Weimerskirch & Jouventin Citation1987, Cristol et al. Citation1999, Moore et al. Citation2003, Dale & Steifetten Citation2011). However, sex-discrimination in the field is often difficult, especially in species without apparent dimorphism in colouration (Chochi et al. Citation2002). This is frequently the case in many bird species, however, sexes may show strong size dimorphism which can be observed during the chicks’ growth period.
Birds can be sexed through analysis of their DNA (Griffiths et al. Citation1998; Eilers et al. Citation2012). This approach, however, is relatively time- and economically costly, so in many circumstances it cannot be used or can only be applied over a limited number of samples. Alternatively, discriminant analyses often allow us to sex an individual with just a few biometric variables (Arizaga et al. Citation2008), which are easy and quick to record.
Most gull species have no obvious sexual dimorphism in their plumage colour, but males and females from several species commonly differ in body size (Cramp & Simmons Citation1983, Olsen & Larson Citation2004). Thus, morphological data have been used to sex gulls using discriminant formulae (Fox et al. Citation1981, Coulson et al. Citation1983, Evans et al. Citation1993, Bosch Citation1996, Arizaga et al. Citation2008, Galarza et al. Citation2008, Aguirre et al. Citation2009). Most of such functions (if not all), however, have been derived for adult birds and, accordingly, the sex of chicks cannot be determined using biometrics. There could, however, be sex-specific variation in morphology during the growth period, so it might be possible to sex chicks using biometrics alone.
Among gulls, males are frequently the larger sex (Cramp & Simmons Citation1983, Olsen & Larson Citation2004), but relatively little is known about differences in growth rates between the sexes. The aims of this study were: (1) to describe the growth rate of Yellow-legged Gull chicks and the possible sex-associated variations in this rate, testing whether males grow slower than females and (2) to obtain a reliable discriminant function method to sex pre-fledging birds using morphological data.
Methods
Sampling site and protocol
This study was carried out between 16 May and 24 June 2011 at the Ulia colony (42°20′N 01°57′W), municipality of Donostia-S. Sebastián, Gipuzkoa, in northern Spain. Around 500 pairs of Yellow-legged Gulls breed in this colony (Arizaga et al. Citation2009). The birds in this population have been shown to have a mean clutch size of 3 eggs/nest, with a 88.4% hatching rate (Arizaga et al. Citation2012). The colony size trend was found to be unclear, probably stable or showing a moderate decrease (Arizaga et al. Citation2014). Survival values vary from year to year, with first-year birds having a mean annual survival of 0.4–0.6, while adults have a mean annual survival rate of 0.8–0.9 (Juez et al. Citation2015). Chick diet also varies from year to year; during the period 2007–09 they were assessed to feed on marine prey (approximately 40%), garbage (approximately 40%) or invertebrates of terrestrial origin (approximately 20%) (Arizaga et al. Citation2013).
Meteorological/logistic conditions permitting, the colony was visited daily between 16:00 and 20:00 hours, with a mean duration of approximately 2 hours per day. From an original sample of 84 nests in total, 150 chicks were measured first when found completely out of the shell and given an age of 1 day. Chicks were measured subsequently until the day in which the study was finished, up to a maximum of 36 days from hatching date. Since hatching date varied between chicks, not all chicks were measured up to day 36. Chicks older than 36 days were not measured as they were able to fly and, accordingly, measuring would have been difficult and potentially risky for their welfare. Chicks were individually identifiable with a code of coloured Velcro bands until the tarsus had grown sufficiently to safely fit a numbered metal ring together with a Darvic ring (Arizaga et al. Citation2010). Six measurements were recorded: body mass (MASS; ±10 g), tarsus length (TARS; ±0.1 mm), and four bill-associated measurements (HEAD; BILL1, BILL2 and BILL3; ±0.1 mm; ).
DNA analyses were used to sex the chicks (Griffiths et al. Citation1998). From each bird, we took 2–4 dorsal feathers that were stored in paper envelopes until they were analysed in a laboratory at the Department of Zoology and Ecology, University of Navarra. These feathers were generally taken when the chicks were older than 25 days. In the laboratory the DNA fragment relative to the chromodomain-helicase-DNA-binding protein (CHD), present in both Z and W chromosomes, was amplified by means of a polymerase chain reaction (PCR). PCR fragments were separated by electrophoresis on a 2.5% agarose gel. According to Griffiths et al. (Citation1998), a single band of DNA on the gel indicate that a bird was male (corresponding to CHD-Z gene), while two bands were present in females (corresponding to both CHD-Z and CHD-W). Overall, 52 birds were sexed (28 males, 24 females), hence these birds were the ones used in our analyses. The sex of the others (98 chicks) was unknown either because they died before feathers could be collected or because we did not find them. All sexed birds were very likely to be alive at least up to day 36 after hatching (J. Arizaga, pers. obs.).
Data analyses
Statistical analyses were conducted with PAST (Hammer et al. Citation2001) and R (R Core Team Citation2014). First, we aimed to obtain a growth rate function, and test for possible sex differences using logistic models, since these models have been reported to fit growth data better than alternative models, such as the Gompertz and von Bertalanffy models (Velando et al. Citation2000). The logistic function is: , where y is the size of the trait measured; A is the asymptote (the final value of that trait in that bird, once it is fully grown); B is the intercept; k is the global growth rate and t is the age of the bird. In this function, the instantaneous growth rate, g, is obtained by derivation of the original function:
; and the fastest growth rate, gmax, is achieved at the inflection point (A/2):
(Ricklefs Citation1968, Citation1969, Citation1973, Richner Citation1991). The logistic models were run using the non-linear estimation with the Levenberged–Marquardt algorithm. The logistic growth curves are symmetrical with regard to the inflection point. To test whether this function varied between sexes we first calculated a function for each individual and, second, the parameters of the function (A, k and gmax) were compared for the two sexes using Generalized Linear Mixed Models on each parameter with the sex as a factor and nest (brood) identity as a random factor (chicks were raised in broods containing 1–3 chicks). We used a linear-link function with Gaussian errors distribution.
Second, we aimed to obtain a discriminant function allowing us to sex the chicks. We conducted a stepwise Discriminant Analysis with all our biometric variables. Additionally, in order to compare whether the body mass and body size of the chicks differed during the growth period, we used Generalized Linear Mixed Models on these variables every 5 days (days 10, 15, … , 35), with the sex as a factor and nest (brood) identity as a random factor. As not all chicks were measured at the same age, body mass and body size were assessed from the individual logistic functions (Velando et al. Citation2000). Regarding body size, we used component one (PC1) from a Principal Component Analysis on all the biometric variables. With an eigenvalue of 4.88, PC1 explained 97.5% of the variance and had a high and positive correlation coefficient with all the variables, so it was an excellent surrogate of body size.
Results
Tarsus and mass had the greatest k (global growth rate) values, and the growth rate did not vary significantly between the two sexes (). By contrast, A values varied significantly between sexes except for BILL1 (; ). The constant gmax was also similar between the sexes, except for the mass ().
Figure 2. Logistic models used to quantify the growth rate function in the two sexes for each variable.
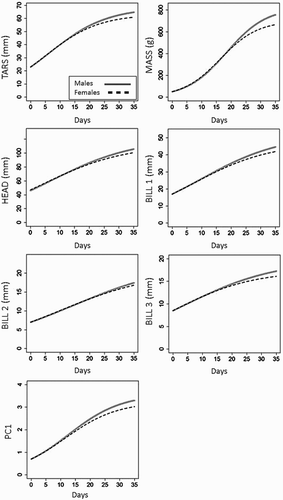
Table 1. Parameters (mean ± se) of the logistic models (A, asymptote; k, growth constant; gmax, maximum instantaneous growth rate) fitted to quantify individual growth in body mass (g) and the different morphometric variables (mm) of Yellow-legged Gull chicks (nmale = 28; nfemale = 24). Sex differences were tested with Generalized Linear Mixed Models with sex as a factor and nest identity as a random factor.
The discriminant function improved with the age of the chicks, with 80% of the chicks being correctly sexed at the age of 10 days, and 100% correctly sexed at the age of 36 days ().
Table 2. Results from a stepwise discriminant analysis used to sex the Yellow-legged Gull chicks at the age of 10, 15, 20, 25 and 30 days. For each case, we indicate the number of steps considered by DA, the coefficients of the discriminating function, the statistics associated to the function (λ and its associated degrees of freedom, df) and the associated P values, the percentage (%) of correct classifications and threshold values of the discriminant function (values >y indicate that a bird is a male, and values <y indicate that a bird is a female).
According to Generalized Linear Mixed Models, body mass was significantly higher among males after they were 10 days old (). By contrast, body size only differed up to the age of 15 days, without significant differences between the sexes after 15 days after hatching ().
Table 3. Comparison of body mass and body size (PC1) of male and female Yellow-legged Gull chicks (mean ± se) across the growth period. Sex differences were tested using Generalized Linear Mixed Models with sex as a factor and nest identity as a random factor.
Discussion
The logistic models used to describe the growth patterns of Yellow-legged Gull chicks showed that the global growth rate (k) and the maximum instantaneous growth rate (gmax) were similar between the sexes. Although in some gulls such rates have been shown to be similar between the sexes (Chochi et al. Citation2002), this does not seem to be very frequent (Jehl et al. Citation1990). Thus, in other birds it is often seen that males show lower global growth rates than females, because the higher rates allow them to compete with more success for resources (e.g. Velando et al. Citation2000). If the first chick in hatching is a male, however, its larger size and higher energy demand can result in female siblings having lower growth rates and also lower survival rates (Kim & Monaghan Citation2006). In our case, however, females did not reach higher growth rates than males.
For most of the measured variables males had a larger size than females, and had significantly larger asymptotes within the growth model. As the sexes had similar growth rates for the measured traits, males reached their mature size later than females. A longer rearing period in males is associated with a higher parental dependence, and this may have direct consequences on nestling survival (Torres & Drummond Citation1997). In our population, however, it remains to be investigated whether males have lower survival rates than females during this period of life.
The discriminant function was reported to have an acceptable power for birds over 30 days old, and for chicks over 35 days we were able to sex all successfully. The variable head+bill length had the highest discriminant capacity, although at the age of >35 days the best predicting variable was tarsus length. Both variables have been reported to have a high discriminant capacity in other gull species (Fox et al. Citation1981, Coulson et al. Citation1983), and in the adult Yellow-legged Gull in particular (Arizaga et al. Citation2008, Galarza et al. Citation2008).
Several studies have focused on sexing adult Yellow-legged Gulls (Bosch Citation1996, Arizaga et al. Citation2008, Galarza et al. Citation2008, Aguirre et al. Citation2009). All of these include both feather-length (mostly wing length) and skeletal measures (tarsus length and some bill-length measurements) in their formulae which, however, cannot be used in chicks while they are still growing. The size of the gulls can differ between populations (Evans et al. Citation1993, Aguirre et al. Citation2009), and so the discriminant function provided here is likely to be acceptable for the northern Atlantic Yellow-legged Gull subspecies (Larus michahellis lusitanius), which shows rather little size variation along its range of distribution (Galarza et al. Citation2008). However, the function should be tested for the Mediterranean birds (Larus michahellis michahellis), which have shown to be larger than the Atlantic ones (Bosch Citation1996).
In conclusion, we found no differences in the growth rates between the sexes, but their final size values did differ, hence males needed more time to reach their asymptotic size. Discriminant analyses showed that the variable head+bill was very reliable in predicting the sex of >80% of chicks at an early developmental stage, and 100% of chicks if combined with tarsus length and two more bill-length associated variables at the age of 35 days.
Acknowledgements
We are grateful to the volunteers who helped us in the field work, especially to the members of the Txingudi Ringing Station (Aranzadi Sciences Society). Two anonymous referees provided very valuable comments that helped us to improve an earlier version of this work.
Additional information
Funding
References
- Aguirre, J.I., Arana, P. & Antonio, M.T. 2009. Testing effectiveness of discriminant functions to sex different populations of Mediterranean Yellow-legged Gulls Larus michahellis michahellis. Ardeola 56: 281–286.
- Amundsen, T. & Stokland, J.N. 1988. Adaptive significance of asynchronous hatching in the Shag: a test of the brood reduction hypothesis. J. Anim. Ecol. 57: 329–334. doi: 10.2307/4909
- Arizaga, J., Aldalur, A., Herrero, A. & Galicia, D. 2008. Sex differentiation of Yellow-legged Gull (Larus michahellis lusitanius): the use of biometrics, bill morphometrics and wing tip coloration. Waterbirds 31: 211–219. doi: 10.1675/1524-4695(2008)31[211:SDOYGL]2.0.CO;2
- Arizaga, J., Galarza, A., Herrero, A., Hidalgo, J. & Aldalur, A. 2009. Distribución y tamaño de la población de la Gaviota Patiamarilla Larus michahellis lusitanius en el País Vasco: tres décadas de estudio. Revista Catalana d'Ornitologia 25: 32–42.
- Arizaga, J., Herrero, A., Galarza, A., Hidalgo, J., Aldalur, A., Cuadrado, J.F. & Ocio, G. 2010. First-year movements of Yellow-legged Gull (Larus michahellis lusitanius) from the southeastern Bay of Biscay. Waterbirds 33: 444–450. doi: 10.1675/063.033.0403
- Arizaga, J., Aldalur, A., Cuadrado, J.F., Díez, E., Goikoetxea, J., Herrero, A., Jauregi, J.I., Laso, M. & Sánchez, J.M. 2012. Parámetros reproductores de la gaviota patiamarilla Larus michahellis lusitanius Naumann, 1840 en Gipuzkoa. Munibe 60: 167–174.
- Arizaga, J., Jover, L., Aldalur, A., Cuadrado, J.F., Herrero, A. & Sanpera, C. 2013. Trophic ecology of a resident Yellow-legged Gull (Larus michahellis) population in the Bay of Biscay. Marine Environ. Res. 87–88: 19–25. doi: 10.1016/j.marenvres.2013.02.016
- Arizaga, J., Aldalur, A. & Herrero, A. 2014. Tendencia poblacional en tres colonias de gaviota patiamarilla Larus michahellis Naumann, 1840 en Gipuzkoa: 2000–2013. Munibe 62: 61–69.
- Bortolotti, G.R. 1986. Influence of sibling competition on nestling sex ratios of sexually dimorphic birds. Am. Nat. 127: 495–507. doi: 10.1086/284498
- Bosch, M. 1996. Sexual size dimorphism and determination of sex in Yellow-legged Gulls. J. Field Ornithol. 67: 534–541.
- Chochi, M., Niizuma, Y. & Takagi, M. 2002. Sexual differences in the external measurements of Black-tailed Gulls breeding on Rishiri Island, Japan. Ornithol. Sci. 1: 163–166. doi: 10.2326/osj.1.163
- Coulson, J.C., Butterfield, J.E., Duncan, N., Thomas, N., Monaghan, P. & Shedden, C. 1983. The use of head and bill length to sex live gulls Laridae. Ibis 125: 549–557. doi: 10.1111/j.1474-919X.1983.tb03148.x
- Cramp, S. & Simmons, K.E.L. 1983. Handbook of the Birds of Europe, the Middle East and North Africa. Vol. 3. Oxford University Press, Oxford.
- Cristol, D.A., Baker, M.B. & Carbone, C. 1999. Differential migration revisited. Latitudinal segregation by age and sex class. In Nolan, V.J., Ketterson, E.D. & Thompson, C.F. (eds) Current Ornithology. 15: 33–88. Academic Press, New York.
- Dale, S. & Steifetten, Ø. 2011. The rise and fall of local populations of Ortolan Buntings Emberiza hortulana: importance of movements of adult males. J. Avian Biol. 42: 114–122. doi: 10.1111/j.1600-048X.2010.05147.x
- Eilers, A., Schmitz, A. & Haase, M. 2012. Sex at second sight. Pitfalls of sexing Water Rails Rallus aquaticus and Spotted Crakes Porzana porzana using morphology and molecular techniques. Acta Ornithol. 47: 1–9. doi: 10.3161/000164512X653863
- Evans, D.R., Hoopes, E.M. & Griffin, C.R. 1993. Discriminating the sex of Laughing Gulls by linear measurements. J. Field Ornithol. 64: 472–476.
- Fox, G.A., Cooper, C.R. & Ryder, J.P. 1981. Predicting the sex of Herring Gulls by using external measurements. J. Field Ornithol. 52: 1–9.
- Galarza, A., Hidalgo, J., Ocio, G. & Rodríguez, P. 2008. Sexual size dimorphism and determination of sex in Atlantic Yellow-legged Gulls Larus michahellis lusitanius from nortthern Spain. Ardeola 55: 41–47.
- Griffiths, R., Double, M.C., Orr, K. & Dawson, R.J.G. 1998. A DNA test to sex most birds. Mol. Ecol. 7: 1071–1075. doi: 10.1046/j.1365-294x.1998.00389.x
- Hammer, Ø., Harper, D.A.T. & Ryan, P.D. 2001. PAST: palaeontological statistics software package for education and data analysis. Palaentologia Electronica 4.
- Jehl, R.J., Francine, J. & Bond, S.I. 1990. Growth patterns of two races of California Gull raised in a common environment. Condor 92: 732–738. doi: 10.2307/1368692
- Juez, L., Aldalur, A., Herrero, A., Galarza, A. & Arizaga, J. 2015. Effect of age, colony of origin and year on survival of Yellow-Legged Gulls Larus michahellis in the Bay of Biscay. Ardeola 62: 139–150. doi: 10.13157/arla.62.1.2015.139
- Kalmbach, E. & Benito, M.M. 2007. Sexual size dimorphism and offspring vulnerability in birds. In Fairburn, D.J., Blanckenhorn, W.U. & Szekely, T. (eds) Sex, Size and Gender Roles: Evolutionary Studies of Sexual Size Dimorphism, 133–142. Oxford University Press, Oxford.
- Kim, S.Y. & Monaghan, P. 2006. Sex of the first hatched chick influences survival of the brood in the Herring Gull (Larus argentatus). J. Zoo. 270: 116–121. doi: 10.1111/j.1469-7998.2006.00155.x
- Moore, F.R., Mabey, S. & Woodrey, M. 2003. Priority access to food in migratory birds: age, sex and motivational asymmetries. In Berthold, P., Gwinner, E. & Sonnenschein, E. (eds) Avian Migration: 281–291. Springer-Verlag Berlin Heidelberg, Berlin.
- Olsen, K.M. & Larson, H. 2004. Gulls of Europe, Asia and North America. Christopher Helm, London.
- R Core Team. 2014. R: a language and environment for statistical computing. http://www.R-project.org.
- Richner, H. 1991. The growth dynamics of sexually dimorphic birds and Fisher’s sex ratio theory: does sex-specific growth contribute to balanced sex ratios? Funct. Ecol. 5: 19–28. doi: 10.2307/2389552
- Ricklefs, R.E. 1968. Patterns of growth in birds. Ibis 110: 419–451. doi: 10.1111/j.1474-919X.1968.tb00058.x
- Ricklefs, R.E. 1969. Preliminary models for growth rates in altricial birds. Ecology 50: 1031–1039. doi: 10.2307/1936894
- Ricklefs, R.E. 1973. Patterns of growth in birds. II. Growth rate and mode of development. Ibis 115: 177–201. doi: 10.1111/j.1474-919X.1973.tb02636.x
- Torres, R. & Drummond, H. 1997. Female-biased mortality in nestlings of a bird with size dimorphism. J. Anim. Ecol. 66: 859–865. doi: 10.2307/6001
- Velando, A., Graves, J. & Freire, J. 2000. Sex-specific growth in the European Shag Strictocarbo aristotelis, a sexually dimorphic seabird. Ardea 88: 127–136.
- Weimerskirch, H. & Jouventin, P. 1987. Population dynamics of the Wandering Albatross, Diomedea exulans, of the Crozet Islands: causes and consequences of the population decline. Oikos 49: 315–322. doi: 10.2307/3565767