ABSTRACT
Capsule: Non-breeding waterbirds are more likely to respond to drone approach when in larger flocks, and responses are more likely in arable and coastal habitats than at inland lochs.
Aims: To investigate the extent to which drones are a potential source of disturbance to non-breeding waterbirds.
Methods: Using a commercially available quadcopter drone, we approached waterbird flocks of varying sizes in coastal, freshwater, and arable habitats following a standardized protocol.
Results: Waterbirds at coastal sites and in arable fields were more likely to respond to drone approach than those at inland freshwater bodies. Larger flocks were more likely to respond to drone approach and responded at a greater distance than smaller flocks.
Conclusion: Repeated drone use at coastal and arable sites with large aggregations of feeding or roosting waterbirds could cause energetically costly flight responses, increased stress, and effective loss of available habitat. At such sites, it may be beneficial to regulate recreational and commercial drone use to minimize potential disturbance effects.
Drone technology has improved markedly in recent years, facilitating mass production and much-reduced retail prices (Ahmed Citation2015). Drones are now being used for recreational photography, surveillance, ecological research, and remote sensing (Anderson & Gaston Citation2013, Christie et al. Citation2016) and may even be used to deliver packages (Gatteschi et al. Citation2015). Global sales of drones were estimated at 10 million in 2017 and are projected to rise to 29 million in 2021 (Lin et al. Citation2017). The mass proliferation of drones and the likelihood of commercial and recreational drone use taking place in proximity to wildlife creates a new and potentially significant source of anthropogenic disturbance of wild birds (Allport Citation2016, Mulero-Pázmány et al. Citation2017). Indeed, a Youtube search for ‘drone footage birds’ yields hundreds of videos of recreational drone users approaching birds with drones, often causing agitated responses or flushing.
Drones are also being increasingly used as a tool to assist in ornithological research, particularly for monitoring breeding birds and breeding bird colonies (Chabot et al. Citation2015, Hodgson et al. Citation2016). Using drones to take aerial images or videos is assumed to cause less disturbance than traditional monitoring methods (Chabot et al. Citation2015), which might involve a fieldworker entering a breeding bird colony to count nests (Walsh et al. Citation1995). The counting of large aggregations of wintering birds could also be made easier using drones (Chabot & Bird Citation2012, Drever et al. Citation2015). Relatively small, single-species groups of Mallard Anas platyrhynchos (flock size range 3–9), Greenshank Tringa nebularia (11–27), and Greater Flamingo Phoenicopterus ruber (5–73) were approached with a drone to within 4 m with no observable responses in 80% of cases (Vas et al. Citation2015). In contrast, larger groups (flock sizes estimated from 75 to 7500) of feeding or roosting wintering waders frequently took flight in response to drone approach, even when drones were flown at heights of 61–122 m (Drever et al. Citation2015). Larger flocks are also more likely to respond to other forms of disturbance (Owens Citation1977). The risk of aerial predation at a site may also influence the likelihood of responses to drones, particularly if these arise from anti-predator vigilance (Metcalfe Citation1984, Frid & Dill Citation2002).
There is a need for more research on how birds respond to drones (Mulero-Pázmány et al. Citation2017) to ensure that if recreational and commercial drone use becomes ubiquitous, effective regulations are in place to mitigate negative impacts. The repeated flushing of non-breeding waterbirds, particularly those species of waders and wildfowl which gather in large flocks in the non-breeding season in the UK (Frost et al. Citation2018), could increase energetic expenditure and reduce available feeding time, and also lead to effective habitat loss (Burton et al. Citation2002, Citation2006). This study aims to further our knowledge of how non-breeding waterbirds birds could be affected by drone use and investigate whether drones could be a useful aide to monitor non-breeding waterbirds. We hypothesize that variation in responses to drones may be driven by effects of (i) flock size, (ii) habituation to human activity, and (iii) habitat. To investigate this, we carried out systematic approaches to flocks of waterbirds with drones in a set of study sites with differing characteristics.
Methods
Study sites
Flocks of non-breeding waterbirds were approached with a drone in October 2016 at eight different sites on or near to the Firth of Forth, Scotland (). Four of these sites were coastal sites, directly on the Forth Estuary with large tidal areas where waders, ducks, and geese foraged (and roosted at high tide); three of the sites were small inland freshwater bodies (lochs) with common waterbird species; and the remaining site was arable stubble fields where geese and shorebirds foraged. The inland loch sites were regularly used for human recreation (dog-walkers and joggers, etc.), while the coastal and arable sites were comparatively less disturbed. For the period (18 days) during which the fieldwork was carried out, these sites were visited in rotation (avoiding strong winds and rain) to ensure an even spread of visits across sites during this period.
Figure 1. Location of field sites in central Scotland where waterbird flocks were approached with drones. Inland loch sites are shown with triangles, coastal sites with circles, and the arable habitat site is shown with a square.
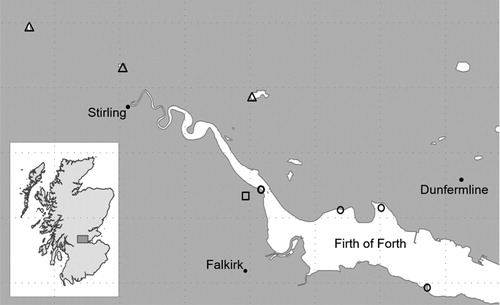
At the coastal sites and inland lochs, some of the flocks approached were mixed-species flocks, whereas in the arable stubble fields, all flocks approached were single-species flocks. We carried out tests on whichever aggregations of birds were present, rather than attempting to gather data on specific species.
Field methods
The DJI Phantom drone 2 (DJI, Shenzhen, China) was used for this research. At the time fieldwork was carried out this was the best-selling drone in the UK. It is a quadcopter drone, weighs 1.38 kg, has a diameter of 35 cm, and was equipped with a GoPro Hero 4 camera (GoPro, San Mateo, USA) to relay live images back to the drone pilot and record video or still images. A Flysight Black Pearl screen (Flysight, Calgary, Canada) was used to view live images from the GoPro camera, and the FlyTrex Core 2 system (Flytrex, Tel Aviv, Israel) was used to record and transmit flight parameters (drone height and speed) back to the screen.
Launching drones at distances of less than 100 m from target birds has been found to increase the chances of eliciting disturbance responses (Vas et al. Citation2015, Hodgson & Koh Citation2016) so, in all cases, our drone was launched 100 m or more from the target flock. The drone was flown at a constant height of 30 m when approaching birds. We selected this height because this is approximately the height at which (with the setup we were using) it was possible to take reasonable images or video recordings of birds. One fieldworker operated the drone, while a second fieldworker watched the target flock through binoculars or a telescope, and recorded responses of the flock to the drone. For all tests (n = 101), the drone was flown to a height of 30 m and then flown towards the flock at a speed of 2–3 m/s and hovered above the target flock for 20 seconds. Following this, the test was considered complete and the drone flown back to the launch site.
Flock response was recorded as either (i) no response, (ii) irregular head movements/calling, (iii) running/swimming away, or (iv) flight. The highest level response observed by any bird within the flock during the test was recorded as the flock response. In 89% of instances where a response was recorded, all the other birds in the flock also exhibited the same response. We also recorded the position of the drone relative to the birds at the time a first response was recorded, in effect recording flight initiation distance (FID). FID is the distance from a source of disturbance at which birds flush, a measure which is often used to assess the disturbance effects of human activities on birds (Blumstein Citation2003, Schwemmer et al. Citation2011, Weston et al. Citation2012). We grouped recorded responses into three categories: distant (>100 m), on approach (25–100 m) and overhead (0–25 m). Following a drone approach at a site, we only carried out subsequent approaches at the same site where it was reasonable to assume that birds had been unaffected by the previous approach, for example, in a different area of the site or if a new flock of birds arrived.
Analyses
Two approaches to analysing the data were used. All statistical analyses were carried out using the software program R (version 3.5.0; R Core Team Citation2017).
The first approach used a generalized linear mixed model (GLMM). Initially, we attempted to use an ordered categorical dependent variable with the categories being the position of the drone relative to the birds at the time of response, but models did not converge using this approach, so we used a binary dependent variable: ‘response’ or ‘non-response’ where ‘flight’, ‘swim/run’ and ‘irregular head movement’ responses were grouped together in the ‘response’ category. The model used a binomial distribution and a logit link function. The explanatory factors tested in the model were the categorical variables: ‘habitat’ (coastal, loch, arable); ‘pre-approach behaviour’ (feeding, roosting, loafing); ‘flock composition’ (waders, ducks, geese or mixed), and the continuous variable ‘flock size’ which was log-transformed to ensure normality. The individual site at which tests were carried out was included in the model as a random effect to account for the possibility of site-specific effects.
The second approach was specifically to investigate the effect of flock size on FID. We grouped the data by flock size into two categories ‘large flocks’ (> 25 birds) and ‘small flocks’ (< 25 birds) and used chi-squared tests to investigate whether FID category (described above) varied significantly between large flocks and small flocks; 25 was selected as the threshold because there was a natural break in the data.
Results
We performed a total of 101 drone approaches over an 18 day period in October 2016 (). Thirty-six approaches at inland lochs were carried out, with responses recorded on five occasions (one ‘swimming away’ response and four ‘irregular head movements/calls’). On the other, 31 approaches ‘no response’ was recorded. Fifty-seven approaches to flocks were carried out at coastal sites with responses recorded 35 times (32 flight responses, one swim/run and two ‘irregular head movements’), and no response recorded 22 times. Eight approaches were carried out in arable stubble fields on single-species feeding flocks of either waders or geese and responses were recorded on all eight occasions. Tests are summarized by habitat in .
Figure 2. Summary of all drone approaches to waterbird flocks by habitat type and flock size. Each box represents the second and third quartiles, the bold line represents the median value, and the whiskers represent the first and fourth quartiles. The width of each box is proportional to the number of records in that category, and each individual trial is represented by a dot (with a jitter effect to make overlapping points discernible). In the arable habitat, all eight approaches resulted in a flock response. The y-axis scale is logged.
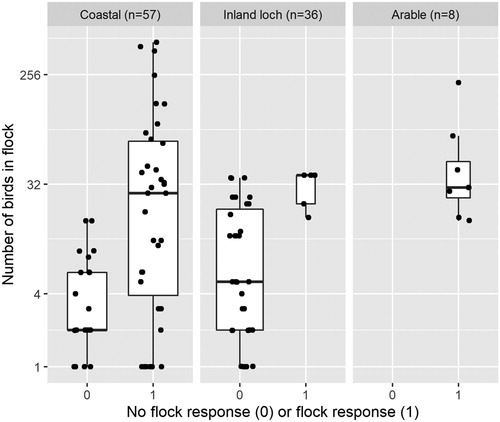
Table 1. Species most frequently present in flocks approached with the drone in each habitat.
The binomial model included significant effects of flock size (Est = 0.82, P = 0.005) and habitat type on the likelihood of flock response. Responses were more likely at coastal sites (Est = 3.36, P = 0.002) and the arable site (Est = 3.49, P = 0.03) relative to inland lochs. Likelihood of response was not significantly affected by species or pre-approach behaviour ().
Table 2. Summary of binomial mixed models of waterbird flock responses to drone approach in coastal, arable and inland loch habitats.
A chi-squared test revealed a significant association between flock size (two categories) and FID (four categories) (χ² 3, 101 = 38, P < 0.001), with large flocks more likely to respond to the drone at greater distance ().
Figure 3. Box and whisker plot of drone position relative to approached waterbird flock at time of response and flock size. The drone position categories are Overhead: drone within 25 m of flock; On approach: drone 25–100 m from flock; and Distant: drone more than 100 m from flock. The boxes represent the second and third quartiles, the bold line represents the median value, and the whiskers represent the first and fourth quartiles. The width of each box is proportional to the number of records in that category, and each individual trial is represented by a dot (with a jitter effect to make overlapping points discernible). The y-axis scale is logged.
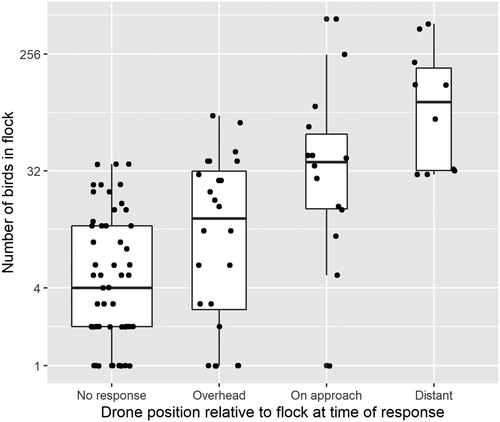
Discussion
This research shows that drones can cause flushing responses in non-breeding waterbird flocks. Compared to smaller flocks, larger flocks were more likely to respond to drone approach and were likely to respond to the approaching drone at a greater distance. Responses varied significantly between the different category of site tested, with all tests eliciting a response in arable fields, more than half of approaches eliciting responses at coastal sites, but very few responses being elicited at inland lochs. The inland loch sites in this study were regularly subject to other forms of disturbance, with frequent use by walkers, joggers, and dog-walkers, such that birds at these sites may have been more habituated to anthropomorphic disturbance (Walker et al. Citation2006). Birds have been found to be less sensitive to disturbance in habitats where there is more human activity (Mikula Citation2014, Morelli et al. Citation2018). In addition to the effects of habituation, individuals and species that are more sensitive to disturbance may avoid sites subjected to regular disturbance, with less sensitive individuals and species remaining (Gill et al. Citation2001). If the perception of predation risk also drives responses to disturbance (Frid & Dill Citation2002), then the likelihood of predators being present at the different sites would influence responses. Aerial predators (Peregrine Falcon Falco peregrinus and European Sparrowhawk Accipiter nisus) are less likely to hunt at the inland loch sites compared to the coastal and arable sites (pers. obs.), perhaps making birds at the inland loch sites less sensitive to disturbance. Even if birds on the lochs perceived a predation threat, duck species may respond to the threat of aerial predation by remaining on the water rather than taking flight (Fox et al. Citation1994).
On seven tests in arable fields (out of eight in total) all of the approached flock took flight and moved some distance (at least 200 m) often to another field, with the flock making frequent changes of direction as if pursued by an aerial predator. The exception was a flock of Canada Geese Anser canadensis which made irregular head movements and calls but did not take flight. Canada Geese are often present in urban parks and heavily disturbed areas so may be more habituated to disturbance. The apparent high sensitivity to drone approach in arable fields may also be because vegetation obscures birds’ view of their surroundings (the flocks were feeding in fields with winter stubble of approximately 30–40 cm high), making them more susceptible to predation and thus more vigilant (Lima & Dill Citation1990). A similar effect of habitat structure has previously been found in European Starlings Sturnus vulgaris, where feeding flocks show variable responses to disturbance and predation caused by the effect of habitat structure (Devereux et al. Citation2006). Ruddy Turnstone Arenaria interpres and Purple Sandpiper Calidris maritime are also more vigilant when feeding in areas where visibility is impaired (Metcalfe Citation1984). While the propensity to flush may usually be inversely related to the quality of feeding habitat (Gill et al. Citation2001), flocks are likely to feed in arable fields because of better feeding opportunities, even if predation risk, particularly from mammalian predators (Whittingham & Evans Citation2004) is higher. We did not find a significant effect of flock composition on the likelihood of response, although there may have been intra-group differences in response between species. Non-breeding waterbirds often congregate in mixed-species flocks, so separating out species-specific effects on responses would likely require a much larger dataset.
The long-term consequences of increased drone use at important sites for non-breeding waterbirds could depend on the extent to which birds are able to habituate to this novel form of disturbance. Determining whether and to what extent such habituation occurs would require long-term studies, which have yet to be carried out (Mulero-Pázmány et al. Citation2017). If drone use became more frequent at sites used by non-breeding waterfowl, and birds did not habituate significantly, then the resultant increases in energy expenditure and stress could negatively affect non-breeding populations of waterbirds (Burton et al. Citation2002, Burton & Armitage Citation2005, Kirby et al. Citation1993). It is also important to note that even when birds appear habituated to disturbance they can still show higher levels of stress hormones in more disturbed areas (Formenti et al. Citation2015). Long-term consequences would also depend on the availability of alternative sites nearby (Gill et al. Citation2001) as well as interactions between the physiological effects of disturbance and the availability and strength of preference for undisturbed areas (Burton et al. Citation2006). Distinguishing between these effects is complicated by the fact that the propensity of birds to appear tolerant of higher levels of disturbance (or to promptly return to a site following disturbance) may be driven primarily by an absence of suitable alternative sites nearby (Gill et al. Citation2001) and the condition of the birds in question (Beale & Monaghan Citation2004) which in turn will depend on a multitude of other factors.
Based on this research, off-the-shelf quadcopter drones are unlikely to be an effective aide for the monitoring of non-breeding wetland birds, for example for the Wetland Bird Survey (Frost et al. Citation2018). The benefits of counting birds with drones, or from images taken by drones, would primarily arise where there were large flocks present, but here we show that large flocks appear much more likely to flush in response to drone approach. Indeed, at coastal and arable sites where large numbers of non-breeding waterbirds congregate, it may be advisable for site managers to restrict or discourage drone use in order to limit potential disturbance. However, at sites already subjected to regular anthropogenic disturbance, regulating the use of drones may be less important due to the apparent lower sensitivity of waterbirds at these sites. More data on the impacts of drones on wild birds is needed to confirm and refine these findings. Longer-term studies aimed at understanding the extent to which habituation may occur would be particularly valuable. Additionally, where drones are being used in ecological research or monitoring, we would recommend that flight parameters and responses are recorded in a standardized way and aggregated into a larger dataset of responses to drones and facilitate a better understanding of the potential impacts of drone use on wild bird populations.
Acknowledgements
The authors are grateful to The Sound Approach for funding this research, and the contribution of two anonymous reviewers who significantly improved an earlier draft of this manuscript.
Additional information
Funding
References
- Ahmed, M. 2015. “UK hopes drones buzz will prompt lift-off”. Ft.com. Available at <https://www.ft.com/content/c0c5a52e-3b7e-11e5-8613-07d16aad2152> (accessed August 22, 2018).
- Allport, G. 2016. Fleeing by Whimbrel Numenius phaeopus in response to a recreational drone in Maputo Bay, Mozambique. Biodiversity Observations 7: 1–5.
- Anderson, K. & Gaston, K.J. 2013. Lightweight unmanned aerial vehicles will revolutionize spatial ecology. Front. Ecol. Environ. 11: 138–146. doi: 10.1890/120150
- Beale, C.M. & Monaghan, P. 2004. Behavioural responses to human disturbance: a matter of choice? Anim. Behav. 68: 1065–1069. doi: 10.1016/j.anbehav.2004.07.002
- Blumstein, D.T. 2003. Flight-initiation distance in birds is dependent on intruder starting distance. J. Wildl. Manag. 67: 852–857. doi: 10.2307/3802692
- Burton, N.H. & Armitage, M.J. 2005. Differences in the diurnal and nocturnal use of intertidal feeding grounds by Redshank Tringa totanus. Bird Study 52: 120–128. doi: 10.1080/00063650509461381
- Burton, N.H., Rehfisch, M.M. & Clark, N.A. 2002. Impacts of disturbance from construction work on the densities and feeding behavior of waterbirds using the intertidal mudflats of Cardiff Bay, UK. Environ. Manag. 30: 865–871. doi: 10.1007/s00267-002-2733-4
- Burton, N.H., Rehfisch, M.M., Clark, N.A. & Dodd, S.G. 2006. Impacts of sudden winter habitat loss on the body condition and survival of redshank Tringa totanus. J. Appl. Ecol. 43: 464–473. doi: 10.1111/j.1365-2664.2006.01156.x
- Chabot, D. & Bird, D.M. 2012. Evaluation of an off-the-shelf Unmanned Aircraft system for surveying flocks of geese. Waterbirds 35: 170–174. doi: 10.1675/063.035.0119
- Chabot, D., Craik, S.R. & Bird, D.M. 2015. Population Census of a large common Tern colony with a small Unmanned Aircraft. PLoS ONE 10: e0122588. doi: 10.1371/journal.pone.0122588
- Christie, K.S., Gilbert, S.L., Brown, C.L., Hatfield, M. & Hanson, L. 2016. Unmanned aerial systems in wildlife research: current and future applications of a transformative technology. Front. Ecol. Environ. 14: 241–251. doi: 10.1002/fee.1281
- Devereux, C.L., Whittingham, M.J., Fernández-Juricic, E., Vickery, J.A. & Krebs, J.R. 2006. Predator detection and avoidance by starlings under differing scenarios of predation risk. Behav. Ecol 17: 303–309. doi: 10.1093/beheco/arj032
- Drever, M.C., Chabot, D., O’Hara, P.D., Thomas, J.D., Breault, A. & Millikin, R.L. 2015. Evaluation of an unmanned rotorcraft to monitor wintering waterbirds and coastal habitats in British Columbia, Canada. J. Unmanned Veh. Syst. 3: 256–267. doi: 10.1139/juvs-2015-0019
- Formenti, N., Viganó, R., Bionda, R., Ferrari, N., Trogu, T., Lanfranchi, P. & Palme, R. 2015. Increased hormonal stress reactions induced in an Alpine Black Grouse (Tetrao tetrix) population by winter sports. J. Ornithol. 156: 317–321. doi: 10.1007/s10336-014-1103-3
- Fox, A.D., Green, A.J., Hughes, B. & Hilton, G. 1994. Rafting as an anti-predator response of wintering White-headed Duck Oxyura leueoeephala. Wildfowl 45: 232–241.
- Frid, A. & Dill, L. 2002. Human-caused disturbance Stimuli as a form of predation risk. Conserv. Ecol. 6: 11–26. doi: 10.5751/ES-00404-060111
- Frost, T.M., Austin, G.E., Calbrade, N.A., Mellan, H.J., Hearn, R.D., Stroud, D.A., Wotton, S.R. & Balmer, D.E. 2018. Waterbirds in the UK 2016/17: The Wetland Bird Survey. BTO, RSPB and JNCC, in association with WWT. British Trust for Ornithology, Thetford.
- Gatteschi, V., Lamberti, F., Paravati, G., Sanna, A., Demartini, C., Lisanti, A. & Venezia, G. 2015. New frontiers of delivery services using drones: a prototype system exploiting a quadcopter for autonomous drug shipments. In Proceedings of the 2015 IEEE 39th Annual Computer Software and Applications Conference, 2: 920–927.
- Gill, J.A., Norris, K. & Sutherland, W.J. 2001. Why behavioural responses may not reflect the population consequences of human disturbance. Biol. Conserv. 97: 265–268. doi: 10.1016/S0006-3207(00)00002-1
- Hodgson, J.C., Baylis, S.M., Mott, R., Herrod, A. & Clarke, R.H. 2016. Precision wildlife monitoring using unmanned aerial vehicles. Sci. Rep. 6: 22574. doi: 10.1038/srep22574
- Hodgson, J.C. & Koh, L.P. 2016. Best practice for minimising unmanned aerial vehicle disturbance to wildlife in biological field research. Curr. Biol. 26: R404–R405. doi: 10.1016/j.cub.2016.04.001
- Kirby, J.S., Clee, C. & Seager, V. 1993. Impact and extent of recreational disturbance to wader roosts on the Dee estuary: some preliminary results. Wader Study Group Bulletin 68: 53–58.
- Lima, S.L. & Dill, L.M. 1990. Behavioral decisions made under the risk of predation: a review and prospectus. Can. J. Zool. 68: 619–640. doi: 10.1139/z90-092
- Lin, C.A., Sha, K., Mauntel, C., Sachin, A. & Shah, S.A. 2017. Drone delivery of medications: Review of the landscape and legal considerations. Am. J. Health-Syst. Pharm. 74: 582–588.
- Metcalfe, N.B. 1984. The effects of habitat on the vigilance of shorebirds—is visibility important? Anim. Behav. 32: 981–985. doi: 10.1016/S0003-3472(84)80210-9
- Mikula, P. 2014. Pedestrian density influences flight distances of urban birds. Ardea 102: 53–60. doi: 10.5253/078.102.0105
- Morelli, F., Mikula, P., Benedetti, Y., Bussiere, R., Jerzak, L. & Tryjanowski, P. 2018. Escape behaviour of birds in urban parks and cemeteries across Europe: evidence of behavioural adaptation to human activity. Sci. Total Environ. 631: 803–810. doi: 10.1016/j.scitotenv.2018.03.118
- Mulero-Pázmány, M., Jenni-Eiermann, S., Strebel, N., Sattler, T., Negro, J.J. & Tablado, Z. 2017. Unmanned aircraft systems as a new source of disturbance for wildlife: A systematic review. PLoS ONE 12: e0178448. doi: 10.1371/journal.pone.0178448
- Owens, N.W. 1977. Responses of wintering Brent geese to human disturbance. Wildfowl 28: 5–11.
- R Core Team. 2017. R: A Language and Environment for Statistical Computing. R Foundation for Statistical Computing, Vienna, Austria.
- Schwemmer, P., Mendel, B., Sonntag, N., Dierschke, V. & Garthe, S. 2011. Effects of ship traffic on seabirds in offshore waters: implications for marine conservation and spatial planning. Ecol. Appl. 21: 1851–1860. doi: 10.1890/10-0615.1
- Vas, E., Lescroël, A., Duriez, O., Boguszewski, G. & Grémillet, D. 2015. Approaching birds with drones: first experiments and ethical guidelines. Biological Letters 11: 20140754. doi: 10.1098/rsbl.2014.0754
- Walker, B.G., Boersma, P.D. & Wingfield, J.C. 2006. Habituation of adult Magellanic Penguins to human visitation as expressed through behavior and corticosterone secretion. Conserv. Biol. 20: 146–154. doi: 10.1111/j.1523-1739.2005.00271.x
- Walsh, P.M., Halley, D.J., Harris, M.P., del Nevo, A., Sim, I.M.W. & Tasker, M.L. 1995. Seabird Monitoring Handbook for Britain and Ireland. Published by JNCC / RSPB / ITE / Seabird Group, Peterborough.
- Weston, M.A., McLeod, E.M., Blumstein, D.T. & Guay, P. 2012. A review of flight initiation distances and their application to managing disturbance to Australian birds. Emu 112: 269–286. doi: 10.1071/MU12026
- Whittingham, M. & Evans, K. 2004. The effects of habitat structure on predation risk of birds in agricultural landscapes. Ibis 146: 210–220. doi: 10.1111/j.1474-919X.2004.00370.x