ABSTRACT
Capsule:
Recording of behavioural responses to ferry traffic for 11 target species showed that Red-throated Diver Gavia stellata, Slavonian Grebe Podiceps auratus, and Black-throated Diver Gavia arctica were most likely to react to passing vessels.
Aim:
To provide information on how responses to marine traffic vary between waterbird species to inform marine spatial planning and environmental impact assessments in the near-shore environment.
Methods:
We recorded behavioural responses to ferry traffic for 11 target species in near-shore waters: Common Eider Somateria mollissima, Goldeneye Bucephala clangula, Long-tailed Duck Clangula hyemalis, Velvet Scoter Melanitta fusca, Red-breasted Merganser Mergus serrator, Black-throated Diver, Great Northern Diver Gavia immer, Red-throated Diver, European Shag Gulosus aristotelis, Slavonian Grebe and Black Guillemot Cepphus grylle. Responses were analysed using generalized linear models and mixed models.
Results:
Red-throated Diver, Black-throated Diver and Slavonian Grebe were the most likely species to exhibit a response to passing vessels. While Red-throated Divers and Slavonian Grebes were highly likely to flush, Black-throated Divers and Great Northern Divers rarely took flight, instead favouring swim or dive responses. In rougher sea conditions birds were more likely to take flight, and the propensity to respond declined across the wintering period.
Conclusions:
This research provides comparative evidence on the behavioural responses of waterbirds to marine traffic. The results support previous studies which highlighted the high sensitivity of diver species to disturbance and provide new evidence that Slavonian Grebe may also be a high sensitivity species.
Introduction
Anthropogenic activity in the marine environment has increased globally to unprecedented levels in recent years (Halpern et al. Citation2015). The expansion of renewable energy generation (Bailey et al. Citation2014, Inger et al. Citation2009), dredging for aggregates (Todd et al. Citation2015), aquaculture (Gentry et al. Citation2017), cruise tourism (MacNeill & Wozniak Citation2018), and shipping volumes (Tournadre Citation2014) are either directly or indirectly resulting in higher levels of marine traffic, often in ecologically sensitive areas (Yang et al. Citation2018). Marine traffic can negatively affect species and ecosystems in various ways, including through direct mortality from collisions (Elvin & Taggart Citation2008, Pirotta et al. Citation2019), increased pollution (Viana et al. Citation2014), the transportation and release of non-native species (Seebens et al. Citation2013), and disturbance altering behaviour (Bas et al. Citation2017). Marine traffic may also affect species and ecosystems in combination with other anthropogenic activities across larger spatial scales, resulting in indirect, cumulative impacts which are particularly difficult to assess (Halpern et al. Citation2015).
While there has been much research on potential interactions between renewable energy installations and waterbirds (Cook & Robinson Citation2017, Furness et al. Citation2012, Citation2013, Johnston et al. Citation2018, Williamson et al. Citation2016), relatively few studies have examined the effects of marine traffic on waterbirds (Wade et al. Citation2016). Furthermore, understanding the consequences of disturbance or displacement during the wintering or migratory periods requires data on resource and habitat constraints (Gill et al. Citation1996, Goss-Custard et al. Citation2006) which would be very challenging to obtain in a marine environment. As such, relatively little is known about how sensitive waterbird populations are to marine traffic on non-breeding grounds, particularly in near-shore areas. Previous studies in the Baltic Sea have often been carried out far from shore (Fliessbach et al. Citation2019, Larsen & Laubek Citation2005, Schwemmer et al. Citation2011). A recent review (Wade et al. Citation2016) ranked the uncertainty around our understanding of the effect of marine traffic on Great Northern Diver Gavia immer as very high, and high for Black-throated Diver Gavia arctica, Red-throated Diver Gavia stallata, European Shag Gulosus aristotelis, Long-tailed Duck Clangula hyemalis, and Slavonian Grebe Podiceps auratus. Indeed for Great Northern Diver, Black-throated Diver, Red-breasted merganser Mergus serrator, and Slavonian Grebe there has been little prior research on sensitivity to marine traffic disturbance (but see Fliessbach et al. Citation2019, Gittings et al. Citation2015, Gittings & O’Donoghue Citation2016).
Across Europe, Special Protection Areas (SPA) are classified under the Birds Directive (EC Directive on the conservation of wild birds 2009/147) to protect rare, vulnerable, and regularly occurring migratory wild birds. For any proposed plans or projects that have the potential to have a significant effect on the qualifying features of an SPA, the competent licencing authority must undertake an appropriate assessment. For a licence to be granted the competent authority must be satisfied, based on the appropriate assessment, that there will be no adverse effect on site integrity. It is therefore essential that assessments are informed by the best available science. Information on the sensitivity of waterbird species to disturbance from vessels may be required to inform impact assessments for licenced marine activities, such as marine renewable energy generation where increased vessel traffic may be expected during construction or operation.
While behavioural responses to marine traffic cannot be used to predict long-term demographic impacts on populations, in the absence of long-term data they are likely to be the best means of identifying those species which may ultimately be more sensitive to disturbance (Furness et al. Citation2012, Citation2013, Grecian et al. Citation2010). In this study we gathered data on behavioural responses to marine traffic for a suite of target waterbird species in the near-shore environment, increasing the evidence base available to inform impact assessments, planning and policy in the marine environment.
Methods
Study site and target species
The study was carried out in the Orkney Islands () where there is significant potential for both the expansion of offshore renewables and aquaculture (Smith Citation2015), and also significant populations of wintering waterbirds of conservation interest (Humphreys et al. Citation2020, Jackson Citation2018, Upton et al. Citation2018). The target species were the qualifying species of two proposed SPAs (Scapa Flow and North Orkney) for wintering waterbirds in the Orkney near-shore zone: Common Eider Somateria mollissima, Goldeneye Bucephala clangula, Long-tailed Duck, Velvet Scoter Melanitta fusca, Red-breasted Merganser, Black-throated Diver, Great Northern Diver, European Shag, and Slavonian Grebe. Black Guillemot Cepphus grylle (which occurs in large numbers in the study area) and Red-throated Diver (which is a breeding season qualifying feature of the two SPAs in Orkney) were also included.
Field methods
Data were gathered from on board regular passenger ferry services between 28 November 2016 and 7 March 2017. These journeys were relatively short ferry trips (5–35 min) between small piers and harbours on Mainland Orkney and nearby islands, with recording carried out either in the North Orkney or Scapa Flow proposed SPAs. The ferries were between 29 and 53 m in length, had a width of 7–10 m and a top speed of 10–11 knots.
Fieldworkers were positioned at the front of the ferry, either on the bridge or next to the bridge, at a height of 5–7 m above the waterline. Fieldworkers worked in pairs, one identifying and reporting the responses of target species with binoculars, and the second fieldworker recording data. For each trip, the following characteristics were collected: date, time of day, wind (Beaufort scale), sea state (Douglas sea scale – recorded as a categorical variable from zero to five), and tide (divided into four periods: high, low, falling, rising). Observations were not carried out during rain, periods of poor visibility, or in sea states greater than a ‘five’ following recommendations in Camphuysen et al. (Citation2004).
When target species on the water (species already in flight when first seen were ignored) were first seen they were allocated to distance bands perpendicular to the route of the ferry: 0–50 m, 50–100 m, 100–200 m, 200–300 m. Fieldworker distance judgement was periodically tested and calibrated against various landmarks a known distance from the path of the ferry using a GPS (Global Positioning System) device with preloaded Ordnance Survey maps. The GPS device also recorded the routes that the ferries took which assisted with calibration of distances. When multiple birds/flocks were in view, recording of those target species for which fewer data had previously been gathered was prioritized. In more challenging weather conditions it is likely that detectability of some smaller species declined, particularly in the further distance bands, but the method was not dependent on every individual being detected, and weather conditions were not found to affect the ability of fieldworkers to assign a response to a flock or individual.
Each individual or flock was watched until the ferry had passed, and then the response of each individual within the flock was categorized as either: ‘flight’, ‘swim away’, ‘evasive dive’, or ‘no response’. All instances of birds observed diving were considered to be evasive dives, except in cases where birds had been observed making feeding dives as the ferry approached or an individual was observed with prey after surfacing. Where an individual exhibited two types of response, for example ‘swim away’ then ‘flight’, only the most energetic response type was recorded (flight > dive > swim away > no response). Each individual or flock was regarded as an individual data point, and where individuals within flocks exhibiting different responses, we took the most energetic response as the flock response (this was necessary in less than 1% of cases).
Analyses
All analyses were carried out using the statistical software package R (R Core Team Citation2022). Two approaches were used to analyse the data. First, binomial generalized linear models (GLMs) were used to analyse factors influencing each species’ responses, with models fitted using the R package ‘glm2’ (Marschner Citation2011). For each species, two separate models were run: (1) with flight response and (2) all responses (including flight, swim, and dive) as the response variable. The explanatory terms tested in the models were ‘distance from path of ferry’, ‘flock size’, ‘sea state’, ‘time of year’ and ‘area’ (Scapa Flow or North Orkney; ). Distance band was an ordered categorical variable. Sea state was also an ordered categorical variable although for some species sea state categories were grouped in the analysis () because there were limited data for sea states ‘0’ (glassy seas) and ‘5’ (moderately rough swell). The time of year variable was represented by the number of days passed since 1st November (the first month during which surveys were carried out). Time of day was not included in the final models because initial analyses showed it did not have a significant effect on responses for any species.
Second, generalized linear mixed models (GLMMs) using the R package ‘lme4’ (Bates et al. Citation2015), which included data from all species, were used to directly compare responses to marine traffic between species. Separate models were run for ‘flight responses’ and ‘all responses’. The environmental variables in these models were the same as in the GLMs (distance band, sea state, flock size, time of year, North Orkney / Scapa Flow). To account for the fact that observations on the same trip were not independent and the fact that individuals of the target species were more likely to be repeat surveyed on days closer to one another, a random effect for ‘trip ID’ (each individual journey between two ports) was included nested within an effect for ‘week’ (the week within the survey period that the data were gathered). The mixed models also included a random effect for ‘species’. To compare the likelihood of responses between different species, we generated a predicted likelihood of response for each level of the random effect ‘species’ using the ‘predictinterval’ function in the R Package MerTools (Knowles et al. Citation2020). This function estimates fitted values for a term with other model coefficients held at mean values. For each modelling approach initial data exploration was carried out using the protocol described in Zuur et al. (Citation2010), and model assumptions were verified by plotting residuals versus fitted values for each covariate in the model (Zuur & Ieno Citation2016).
Results
Data were recorded on 116 ferry trips (60 in Scapa Flow and 56 in North Orkney), with a total of 6,094 flocks of target species recorded. The most frequently encountered species was Black Guillemot (1,650 records) and the least frequently encountered was Velvet Scoter (1 record). Sample sizes for all species are shown in . Data were collected over six different sea states: zero: 101 min of data gathering, one: 775 min, two: 715 min, three: 664 min, four: 798 min, and five: 25 min. Red-throated Diver (number of flocks encountered = 88, 78% of flocks responding), Slavonian Grebe (n = 34, 76%), and Black-throated Diver (n = 21, 67%) had the highest likelihood to exhibit a response to the passing ferry and Black Guillemot the lowest (n = 1650, 26%). The species most likely to take flight were Red-throated Diver (48%), Slavonian Grebe (41%), and Long-tailed Duck (n = 727, 35%), while Black-throated Diver (0%) and Great Northern Diver (n = 475, 3%) were the least likely, with swim and dive responses accounting for almost all the evasive responses of these two species. It was possible to fit species-specific models for all species () except Goldeneye and Velvet Scoter (small sample sizes) and no flight model was possible for Black-throated Diver (due to no flights being recorded).
Figure 2. Summary of all responses by birds to a passing ferry. Number of records for each species is shown in parenthesis on the x-axis. Species are ordered from left to right in order of proportion of flocks responding.
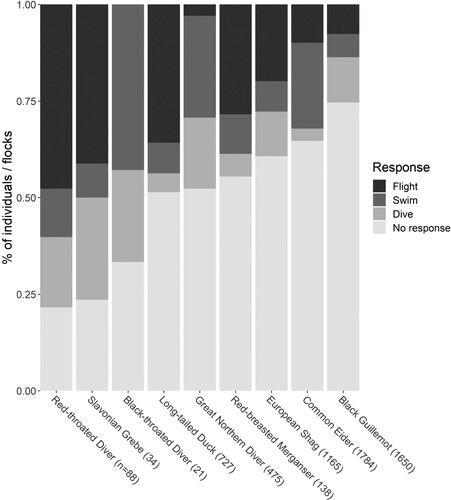
Table 1. Summary of species-specific response models. The ‘All responses’ models used flight, swim and dive responses in the response variable, while the ‘flight response’ modes only included flight responses. ‘Scapa Flow’ is the effect of data gathered in Scapa Flow compared to the reference level, North Orkney (see ). For Slavonian Grebe and Black-throated Diver all records were in Scapa Flow so there is no covariate shown. No Black-throated Diver flights were recorded so no flight model is shown. Statistically significant (P < 0.05) results are shown in bold. For the ordered categorical variables (sea state and distance band) the linear effect is shown, and quadratic effects are only shown where P < 0.05.
In the combined models inclusive of all species, the propensity to take flight or show any response declined significantly with increasing perpendicular distance to the ferry. The species-specific models showed similar significant effects of perpendicular distance for all species except for Red-throated Diver, Black-throated Diver and Slavonian Grebe for which effects were non-significant (, ). There was little support for a non-linear effect of distance band on the likelihood of response or flight, with the strongest effect for all species and in the combined model being the linear term ( and ).
Figure 3. Predicted likelihoods of a) any response (flush, swim or dive) and b) flight responses to the passing vessel for each species at the four distance bands (with 95% confidence intervals) from the species-specific GLMs. Other covariates are held at mean values. There were no flight responses recorded for Black-throated Diver so no flight responses plot is shown for this species.
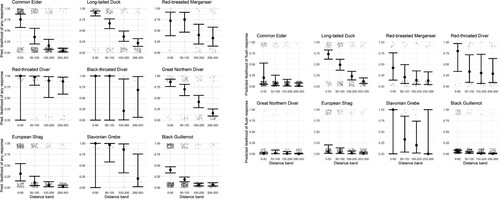
Table 2. Summary of the combined mixed effects models for all responses and flight responses. For the ordered categorical variables ‘distance band’ and ‘sea state’, only the linear and quadratic terms are shown. The fixed effects are the same as for the species-specific models (). The variance explained by the random effects ‘species’, ‘week’ and ‘trip’ (nested in ‘week’) and Marginal and Conditional R2 of each model are also shown. Statistically significant (P < 0.05) results are shown in bold.
Rougher sea states significantly increased the likelihood of flight responses in the species-specific models for Common Eider, European Shag (for European Shag there was a significant effect in the all responses model as well) and Black Guillemot (, ). Sea state also significantly increased the likelihood of flight response in the combined model. Common Eider and Long-tailed Duck were significantly more likely to respond in Scapa Flow, while Great Northern Diver were significantly more likely to respond in North Orkney, although in the combined model there was no significant effect of site on likelihood of response or flight.
Figure 4. Predicted likelihood of flocks flushing at different sea states with covariates held at mean values from the species-specific GLMs. Some sea state categories were combined where sample sizes were small.
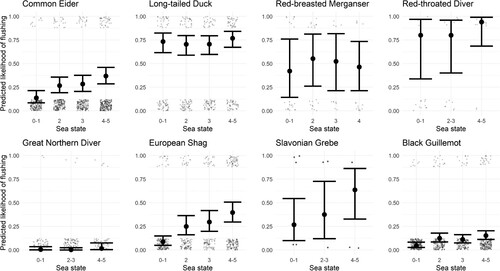
In the species-specific models there were significant effects of flock size for Common Eider (all responses and flight response model), Long-tailed Duck (flight response model), and Black Guillemot (all responses model); however, for these species most of the flocks encountered were small (Common Eider range 1–300, μ = 5.5; Black Guillemot range = 1–19, μ = 1.2; Red-throated Diver range 1–12, μ = 1.6). In the combined models, flock size had a significant positive effect in the all responses model but not in the flight responses model. In the combined ‘all responses’ model there was also a significant effect of time of year, with birds less likely to show a response to the passing ferry later in the wintering period, while there was no significant effect of time of year on flight responses (). The three species showing the highest predicted likelihood of response () were Red-throated Diver, Slavonian Grebe, and Black-throated Diver, while the three species showing the lowest predicted likelihood of response were Black Guillemot, Common Eider and European Shag.
Figure 5. Likelihood of responses predicted from the combined GLMM models for each species, with the ‘any responses’ model predictions on the x-axis and the ‘flight responses’ model predictions on the y-axis. Predicted likelihoods are transformed into binary space where ‘1’ is certain to respond and ‘0’ certain not to respond. All other covariates are at mean values. E = Common Eider, LN = Long-tailed Duck, RM = Red-breasted Merganser, BV = Black-throated Diver, ND = Great Northern Diver, RH = Red-throated Diver, SA = European Shag, SZ = Slavonian Grebe, TY = Black Guillemot.
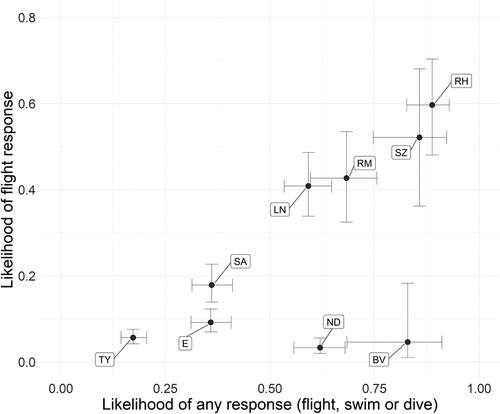
Discussion
This research provides evidence of how a suite of waterbird species respond to marine traffic in the near-shore environment. Our on-ferry survey method facilitated the collection of a large dataset in a cost-effective manner and allowed direct comparison of sensitivities between species. To account for the possibility that individuals of each target species may have been surveyed on multiple occasions (pseudo-replication), in the mixed models we used ‘week’ as a random effect, because we assumed that on dates close to one another, the same individuals were more likely to be present. Unlike previous studies (Schwemmer et al. Citation2011) we didn’t record flight initiation distance, and instead recorded the birds in perpendicular distance to the ferry. This may be a more appropriate data gathering approach for smaller, slower vessels where most responses occurred alongside the vessel. Recording perpendicular distance when boats are following defined routes is also useful because it can be used to quantify the spatial extent of the disturbed zone.
We were able to record three different types of response (flight, evasive swimming, and diving) which allowed us to model the data using both ‘all responses’ and ‘flight responses’ as response variables. While very few of the recorded flights or evasive swims were likely to be unrelated to the disturbance caused by the passing ferry, classifying evasive dives was more challenging, and it is likely that the method used (considering all dives as evasive unless there was evidence of feeding) may have resulted in over-recording of the number of evasive dives. However, we consider it unlikely that this had a material effect on the comparisons between species.
Sensitivity rankings for most species would be similar regardless of whether ‘all responses’ or ‘flight responses’ were used; the clear exceptions to this were Great Northern Diver and Black-throated Diver (). Great Northern Diver and Black-throated Diver almost exclusively responded with evasive swims or dives, so recording flight responses for these species may not adequately reflect sensitivity to disturbance. In the ‘all responses’ model they were the 3rd and 4th most sensitive species (; although note the confidence intervals), while a ‘flight responses’ model would suggest they were the least sensitive species. In contrast, Red-throated Diver was a high sensitivity species whichever approach was used (all responses: 2nd; flight responses: 1st).
Diver species have been a focal species for disturbance sensitivity research in northern European waters for some time (Schwemmer et al. Citation2011, Dierschke et al. Citation2017, Mendel et al. Citation2018), and this difference in responses has also been reported in the Baltic Sea (Fliessbach et al. Citation2019), where 92% of Red-throated Divers were recorded taking flight in response to disturbance, but only 30% of Black-throated Divers. This difference may be partly explained by the fact that Red-throated Divers have lower wing-loading and thus lower energetic costs of take-off than Black-throated or Great Northern Divers (Pennycuick Citation2008). Differing moult strategies may also contribute to this effect: Red-throated Divers aggregate in large post-breeding flocks in remote, undisturbed areas to moult primary feathers in relative safety (Mendel et al. Citation2008). In contrast, Great Northern and Black-throated Divers undergo a simultaneous moult of primary flight feathers on their wintering grounds which leaves them flightless for a period of three to four weeks beginning in late January or February (Appleby et al. Citation1986). This means they were flightless for part of the period during which research was carried out, although the probability of flight was low for these species across the whole of the period of research (there was no significant effect of time of year on the propensity of Great Northern Divers to take flight).
The results from this study () are broadly consistent with data from the Baltic Sea (Fliessbach et al. Citation2019) except for Black Guillemot, which was considered to have higher sensitivity by that study. However, Black Guillemots are a sedentary species in the Orkney Isles so may be more habituated to routine and predictable vessel traffic there than in other regions.
A similar study in the Kattegat Sea, Denmark (Larsen & Laubek Citation2005), where data were gathered on larger faster ferries, reported an 88% flight response rate (n = 230) for Common Eider flocks within 100 m of the ferry, compared to only 18% (n = 882) in this study. This difference is likely attributable to the different speed and size (and noise) of the ferries in the respective studies. We found evidence that the likelihood of target species responding to the passing ferry declined across the wintering period (). This is likely to be evidence for habituation to disturbance (Blumstein Citation2016, Vincze et al. Citation2016). We also found support for the effect of flock size () in increasing the likelihood of response consistent with previous studies on disturbance (Jarrett et al. Citation2020, Laursen et al. Citation2005).
Rougher sea conditions increased the likelihood of flight responses to marine traffic for all species (, ), with statistically significant effects for three species: Common Eider, European Shag, and Black Guillemot (this effect was also reported in Schwemmer et al. Citation2011). The effect was strongest for European Shag, which were approximately four times more likely to flush in a sea state ‘5’ than in a sea state ‘0’. Stronger winds (associated with rougher sea conditions) reduce the energetic costs of take-off and have been shown to alter take-off behaviour in seabirds (Kogure et al. Citation2016). This is supported by the fact that the probability of any response (including evasive swims and dives) did not change significantly in higher sea states for any of the target species except European Shag, suggesting that most species were substituting flight responses for other responses in rougher conditions.
A key question when considered these findings is the extent to which the results here are applicable more widely. It is notable that there are statistically significant differences in responses between the Scapa Flow and North Orkney areas for three species (Common Eider, Long-tailed Duck, and Great Northern Diver), suggestive of localized effects. While the likelihood of individuals responding to marine traffic must be influenced by site-specific effects, it would be reasonable to assume that the relative sensitivities of the species compared here are going to be broadly reflected in similar in-shore waters with similar frequencies and types of marine traffic.
A further limitation of studies on behavioural responses to disturbance is that the propensity of an individual, population, or species to respond to a disturbance stimulus won’t necessarily reflect vulnerability to disturbance (Gill et al. Citation2001). Research on shorebirds suggests that frequent disturbance of wintering birds can contribute to reduced survival and have demographic impacts (Burton et al. Citation2002, Citation2006, Davidson & Rothwell Citation1993), but also that individuals in good physical condition or with alternative foraging sites nearby can be more likely to respond to disturbance or to displace from an area (Beale & Monaghan Citation2004). Understanding the extent to which short-term responses to a disturbance stimulus translate to demographic effects is thus critical to understanding the relevance of disturbance data.
Long-term negative effects of disturbance are driven by the interaction between the (i) energetic costs of disturbance, (ii) the reduction of effective foraging habitat, and (iii) increased stress in response to disturbance (Burton et al. Citation2006, Stillman & Goss-Custard Citation2002, Urfi et al. Citation1996). While flight is thought in most cases to be the most costly mode of locomotion among birds (Nudds & Bryant Citation2000, Pelletier et al. Citation2008), the energetic requirements of flight vary by species (Pennycuick Citation2008), and measuring the energetic costs of diving and swimming is more challenging (Mendel et al Citation2008, Savard et al. Citation2017). Longer-term research on how repeated disturbance affects foraging behaviour, energetic budgets, and body condition (Beuth et al. Citation2016, Burton et al. Citation2002) will be an important next step towards understanding how resilience to disturbance varies between different species. In the absence of longer-term studies, regionally relevant data on behavioural responses to marine traffic should ideally be used in conjunction with data on population status, threats, and prey and habitat availability (Fliessbach et al. Citation2019) to assess relative risks of increased marine traffic for different species.
Acknowledgements
We are grateful to the staff of Orkney Ferries for allowing access to vessels to carry out surveys. Comments from two anonymous reviewers improved an earlier draft of this manuscript.
Disclosure statement
No potential conflict of interest was reported by the author(s).
Additional information
Funding
References
- Appleby, R.H., Madge, S.C. & Mullarney, K. 1986. Identification of divers in immature and winter plumages. Br. Birds 79: 365–391.
- Bailey, H., Brookes, K.L. & Thompson, P.M. 2014. Assessing environmental impacts of offshore wind farms: lessons learned and recommendations for the future. Aquat. Biosyst. 10: 8.
- Bas, A.A., Christiansen, F., Öztürk, A.A., Öztürk, B. & McIntosh, C. 2017. The effects of marine traffic on the behaviour of Black Sea harbour porpoises (Phocoena phocoena relicta) within the Istanbul Strait, Turkey. PLOS ONE 12: e0172970.
- Bates, D., Mächler, M., Bolker, B.M. & Walker, S.C. 2015. Fitting linear mixed-effects models using lme4. J. Stat. Softw. 67: 1–48.
- Beale, C.M. & Monaghan, P. 2004. Behavioural responses to human disturbance: a matter of choice? Anim. Behav. 68: 1065–1069.
- Beuth, J.M., Paton, P.W.C., Osenkowski, J.E. & McWilliams, S.R. 2016. Validating the deuterium dilution method to measure body composition of Common Eider. Wildl. Soc. Bull. 40: 456–463.
- Blumstein, D.T. 2016. Habituation and sensitization: new thoughts about old ideas. Anim. Behav. 120: 255–262.
- Burton, N.H.K., Rehfisch, M.M. & Clark, N.A. 2002. Impacts of disturbance from construction work on the densities and feeding behavior of waterbirds using the intertidal mudflats of Cardiff Bay, UK. Environ. Manag. 30: 865–871.
- Burton, N.H.K., Rehfisch, M.M., Clark, N.A. & Dodd, S.G. 2006. Impacts of sudden winter habitat loss on the body condition and survival of Redshank Tringa totanus. J. Appl. Ecol. 43: 464–473.
- Camphuysen, C.J., Fox, A.D., Leopold, M.F. & Petersen, I.K. 2004. Towards standardised seabirds at sea census techniques in connection with environmental impact assessments for offshore wind farms in the UK: a comparison of ship and aerial sampling methods for marine birds and their applicability to offshore wind farm assessments. Report by Koninklijk Nederlands Instituut voor Onderzoek der Zee for COWRIE.
- Cook, A.S.C.P. & Robinson, R.A. 2017. Towards a framework for quantifying the population-level consequences of anthropogenic pressures on the environment: the case of seabirds and windfarms. J. Environ. Manag. 190: 113–121.
- Davidson, N. & Rothwell, P.I. 1993. Human disturbance to waterfowl on estuaries: conservation and coastal management implications of current knowledge. Wader Study Group Bull. 68: 97–105.
- Dierschke, H., Furness, R.W., Gray, C.E., Petersen, I.K., Schmutz, J., Žydelis, R. & Daunt, F. 2017. Possible behavioural, energetic and demographic effects of displacement of Red-throated Divers. https://hub.jncc.gov.uk/assets/e9cb4b91-cf6d-440c-b9e3-c363bac51f86.
- Elvin, S.S. & Taggart, C.T. 2008. Right Whales and vessels in Canadian waters. Mar. Policy. 32: 379–386.
- Fliessbach, K.L., Borkenhagen, K., Guse, N., Markones, N., Schwemmer, P. & Garthe, S. 2019. A ship traffic disturbance vulnerability index for northwest European seabirds as a tool for marine spatial planning. Front. Mar. Sci. 6: 1–15.
- Furness, R.W., Wade, H.M. & Masden, E.A. 2013. Assessing vulnerability of marine bird populations to offshore wind farms. J. Environ. Manag. 119: 56–66.
- Furness, R.W., Wade, H.M., Robbins, A.M.C. & Masden, E.A. 2012. Assessing the sensitivity of seabird populations to adverse effects from tidal stream turbines and wave energy devices. ICES J. Mar. Sci. 69: 1466–1479.
- Gentry, R.R., Froehlich, H.E., Grimm, D., Kareiva, P., Parke, M., Rust, M., Gaines, S.D. & Halpern, B.S. 2017. Mapping the global potential for marine aquaculture. Nature Ecology and Evolution 1: 1317–1324.
- Gill, J.A., Norris, K. & Sutherland, W.J. 2001. Why behavioural responses may not reflect the population consequences of human disturbance. Biol. Conserv. 97: 265–268.
- Gill, J.A., Sutherland, W.J. & Watkinson, A.R. 1996. A method to quantify the effects of human disturbance on animal populations. J. Appl. Ecol. 33: 786–792.
- Gittings, T. & O’Donoghue, P. 2016. Disturbance response of Red-breasted Mergansers Mergus serrator to boat traffic in Wexford Harbour. Irish Birds 10: 329–334.
- Gittings, T., Peppiatt, C. & Troake, P. 2015. Disturbance response of Great Northern Divers Gavia immer to boat traffic in Inner Galway Bay. Irish Birds 10: 163–166.
- Goss-Custard, J.D., Triplet, P., Sueur, F. & West, A.D. 2006. Critical thresholds of disturbance by people and raptors in foraging wading birds. Biol. Conserv. 127: 88–97.
- Grecian, W.J., Inger, R., Attrill, M.J., Bearhop, S., Godley, B.J., Witt, M.J. & Votier, S.C. 2010. Potential impacts of wave-powered marine renewable energy installations on marine birds. Ibis 152: 683–697.
- Halpern, B.S., Frazier, M., Potapenko, J., Casey, K.S., Koenig, K., Longo, C., Lowndes, J.S., Rockwood, R.C., Selig, E.R., Selkoe, K.A. & Walbridge, S. 2015. Spatial and temporal changes in cumulative human impacts on the world’s ocean. Nat. Commun. 6: 7615.
- Humphreys, E.M., Austin, G.E., Frost, T.M., Mellan, H.J., Boersch-Supan, P., Burton, N.H.K. & Balmer, D.E. 2020. Wader populations on the United Kingdom’s open coast: results of the 2015/16 Non-Estuarine Waterbird Survey (NEWS-III) and a review of population trends. Bird Study 67: 371–384.
- Inger, R., Attrill, M.J., Bearhop, S., Broderick, A.C., James Grecian, W., Hodgson, D.J., Mills, C., Sheehan, E., Votier, S.C., Witt, M.J. & Godley, B.J. 2009. Marine renewable energy: potential benefits to biodiversity? An urgent call for research. J. Appl. Ecol. 46: 1145–1153.
- Jackson, D. 2018. Scapa Flow proposed Special Protection Area (pSPA)—Inshore wintering waterfowl survey 2017/18 (Research Report 1075). Scottish Natural Heritage.
- Jarrett, D., Calladine, J., Cotton, A., Wilson, M.W. & Humphreys, E.M. 2020. Behavioural responses of non-breeding waterbirds to drone approach are associated with flock size and habitat. Bird Study 67: 190–196.
- Johnston, D.T., Furness, R.W., Robbins, A.M.C., Tyler, G., Taggart, M.A. & Masden, E.A. 2018. Black Guillemot ecology in relation to tidal stream energy generation: an evaluation of current knowledge and information gaps. Mar. Environ. Res. 134: 121–129.
- Knowles, J.E., Frederick, C. & Whitworth, A. 2020. merTools: Tools for analyzing mixed effect regression models (0.5.2) https://CRAN.R-project.org/package=merTools.
- Kogure, Y., Sato, K., Watanuki, Y., Wanless, S. & Daunt, F. 2016. European Shags optimize their flight behavior according to wind conditions. J. Exp. Biol. 219: 311–318.
- Larsen, J.K. & Laubek, B. 2005. Disturbance effects of high-speed ferries on wintering sea ducks. Wildfowl 55: 99–116.
- Laursen, K., Kahlert, J. & Frikke, J. 2005. Factors affecting escape distances of staging waterbirds. Wildl. Biol. 11: 13–19.
- MacNeill, T. & Wozniak, D. 2018. The economic, social, and environmental impacts of cruise tourism. Tour. Manag. 66: 387–404.
- Marschner, I.C. 2011. Glm2: fitting generalized linear models with vonvergence problems. R. J. 3: 12–15.
- Mendel, B., Schwemmer, P., Peschko, V., Mueller, S., Schwemmer, H., Mercker, M. & Garthe, S. 2018. Operational offshore wind farms and associated ship traffic cause profound changes in distribution patterns of Loons (Gavia spp.). J. Environ. Manag. 231: 429–438.
- Mendel, B., Sonntag, N. & Wahl, J. 2008. Profiles of Seabirds and Waterbirds of the German North and Baltic Seas: Distribution, Ecology and Sensitivities to Human Activities Within the Marine Environment. Bundesamt für Naturschutz. Landwirtschaftsverlag, Münster.
- Nudds, R.L. & Bryant, D.M. 2000. The energetic cost of short flights in birds. J. Exp. Biol. 203: 1561–1572.
- Pelletier, D., Guillemette, M., Grandbois, J.M. & Butler, P.J. 2008. To fly or not to fly: high flight costs in a large sea duck do not imply an expensive lifestyle. Proc. R. Soc. B Biol. Sci. 275: 2117–2124.
- Pennycuick, C.J. 2008. Modelling the Flying Bird. Amsterdam: Academic Press.
- Pirotta, V., Grech, A., Jonsen, I.D., Laurance, W.F. & Harcourt, R.G. 2019. Consequences of global shipping traffic for marine giants. Front. Ecol. Environ. 17: 39–47.
- R Core Team 2022. R: A language and environment for statistical computing. Vienna, Austria: R Foundation for Statistical Computing.
- Savard, J.P.L., Derksen, D.V., Esler, D., Eadie, J.M. & Skean, V.W. 2017. Ecology and Conservation of North American sea Ducks. Boca Raton: CRC Press.
- Schwemmer, P., Mendel, B., Sonntag, N., Dierschke, V. & Garthe, S. 2011. Effects of ship traffic on seabirds in offshore waters: implications for marine conservation and spatial planning. Ecol. Appl. 21: 1851–1860.
- Seebens, H., Gastner, M.T. & Blasius, B. 2013. The risk of marine bioinvasion caused by global shipping. Ecol. Lett. 16: 782–790.
- Smith, G. 2015. Creating the spaces, filling them up. Marine spatial planning in the Pentland Firth and Orkney Waters. Ocean. Coast. Manag. 116: 132–142.
- Stillman, R.A. & Goss-Custard, J.D. 2002. Seasonal changes in the response of Oystercatchers Haematopus ostralegus to human disturbance. J. Avian Biol. 33: 358–365.
- Todd, V.L.G., Todd, I.B., Gardiner, J.C., Morrin, E.C.N., MacPherson, N.A., DiMarzio, N.A. & Thomsen, F. 2015. A review of impacts of marine dredging activities on marine mammals. ICES J. Mar. Sci. 72: 328–340.
- Tournadre, J. 2014. Anthropogenic pressure on the open ocean: the growth of ship traffic revealed by altimeter data analysis. Geophys. Res. Lett. 41: 7924–7932.
- Upton, A., Williams, S.J. & Williams, E.J. 2018. North Orkney proposed Special Protection Area (pSPA)—Inshore wintering waterfowl survey 2017/18 (Research Report 1074). Scottish Natural Heritage.
- Urfi, A.J., Goss-Custard, J.D. & Durell, S.E.A. 1996. The ability of Oystercatchers Haematopus ostralegus to compensate for lost feeding time: field studies on individually marked birds. J. Appl. Ecol. 33: 873–883.
- Viana, M., Hammingh, P., Colette, A., Querol, X., Degraeuwe, B., Vlieger, I.de & van Aardenne, J. 2014. Impact of maritime transport emissions on coastal air quality in Europe. Atmos. Environ. 90: 96–105.
- Vincze, E., Papp, S., Preiszner, B., Seress, G., Bókony, V. & Liker, A. 2016. Habituation to human disturbance is faster in urban than rural House Sparrows. Behav. Ecol. 27: 1304–1313.
- Wade, H.M., Masden, E.A., Jackson, A.C. & Furness, R.W. 2016. Incorporating data uncertainty when estimating potential vulnerability of Scottish seabirds to marine renewable energy developments. Mar. Policy. 70: 108–113.
- Williamson, B.J., Blondel, P., Armstrong, E., Bell, P.S., Hall, C., Waggitt, J.J. & Scott, B.E. 2016. A self-contained subsea platform for acoustic monitoring of the environment around marine renewable energy devices – field deployments at wave and tidal energy sites in Orkney, Scotland. IEEE J. Oceanic Eng. 41: 67–81.
- Yang, H., Ma, M., Thompson, J. & Flower, R. 2018. Transport expansion threatens the Arctic. Science 359: 646–647.
- Zuur, A. & Ieno, E. 2016. A protocol for conducting and presenting results of regression-type analyses. Methods Ecol. Evol 7: 636–645.
- Zuur, A., Ieno, E. & Elphick, C.S. 2010. A protocol for data exploration to avoid common statistical problems. Methods Ecol. Evol 1: 3–14.