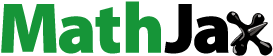
ABSTRACT
1. This study aimed to quantify the effect of fat type (including unsaturated to saturated ratio (U:S)) and increasing doses of lysolecithin-based products on nutrient availability and growth performance in broiler chickens.
2. A total of 33 separate experimental reports were collated according to predetermined selection criteria to provide 16 performance trials with ‘on top’ application, and 17 performance trials using reformulated diets, where the contribution of the lysolecithin was taken into account. Data on average daily gain (ADG) and body weight corrected FCR (FCRc) were analysed using the REML method with trial as a random effect.
3. Across the constituent trials, average added dietary fat and oil inclusion was 4.42% (min 1.15%, max 7.00%), with varied U:S ratio (min 0.94, avg 2.50, max 7.65), reflecting diverse fat sources. Overall, neither bird growth performance nor response to lysolecithin supplementation were significantly affected by the U:S ratio of the diets.
4. In performance trials where lysolecithin was added ‘on top’ of existing formulations, FCRc was significantly reduced by lysolecithin at 250 g/t inclusion compared to the control, with 125 g/t returning an intermediate value. In reformulated trials, FCRc was not significantly affected, suggesting lysolecithin supplementation at 125 and 250 g/t could recover average dietary energy reductions of 57.88 and 73.11 kcal/kg feed, respectively.
5. In conclusion, this study showed that the addition of lysolecithin at levels of 125 g/t and above to broiler diets consistently improved feed efficiency across a range of basal dietary ingredients and fat sources.
Introdution
Fat inclusion in broiler diets provides a concentrated energy source, allowing for increased growth rates and feed conversion efficiency (Pesti et al. Citation2002) while improving feed milling processes. At the same time, fat digestion is poor in immature birds, due to a lack of lipase activity and bile salts production (Maisonnier et al. Citation2003), though both lipase activity and bile salts production is known to increase as the bird ages (Hakansson Citation1974; Noy and Sklan Citation1995). Fat utilisation can be improved through the supplementation of bile salts (Al-Marzooqi and Leeson Citation1999), though this is not economically viable. Therefore, the use of biosurfactants such as phospholipids, lecithins and lysolecithins (Polin Citation1980; Soares and Lopez-Bote Citation2002) is of practical and commercial interest.
Lysolecithins are produced by an enzymatic conversion of lecithin, which is a phospholipid by-product from the processing of vegetable oils (Van Nieuwenhuyzen and Tomás Citation2008). When compared with lecithin, lysophospholipids are more hydrophilic, have better oil-in-water emulsifying properties (Joshi et al. Citation2006; Liu and Ma Citation2011) and therefore, are more able to improve the digestion of fats and oils. Previous research with lysolecithin in broiler diets has demonstrated improved weight gain and feed conversion ratio (FCR) (Zaefarian et al. Citation2015; Zampiga et al. Citation2016; Allahyari-Bake and Jahanian Citation2016), while others have reported increases in apparent metabolisable energy (AME) (Melegy et al. Citation2010; Jansen et al. Citation2015).
These improvements in bird performance can be attributed in part to the ability of lysolecithin to enhance the emulsification of fats (Sugawara et al. Citation2001; Liu and Ma Citation2011), creating smaller droplet sizes to allow for more efficient lipase activity. Lysophospholipids are known to alter phospholipid bi-layers of intestinal cells, inducing local curvature of bilayers (Wendel Citation1995; Mandalari et al. Citation2009) and alterations in protein channel formation, hence increasing ion exchanges (Lundbaek and Andersen Citation1994; Maingret et al. Citation2000), and thus increasing the fluidity and permeability of the membrane. There are documented effects of lysolecithin supplementation on gut microbiota, integrity and gene expression: Polycarpo et al. (Citation2016) found reduced levels of gram-positive cocci in the jejunum, which has been explained as a combination of direct disruption of the bacterial cell membrane (Arouri and Mouritsen Citation2013) and reduced fat available for bacterial growth in the lumen. Brautigan et al. (Citation2017) saw increased expression of collagen-coding genes, along with increased villi collagen cross-linkages and height.
While there is published data available on the effect of lysolecithin on broiler performance, these studies have largely examined the effect of ‘on top’ supplementation, where improved performance is expected. In commercial practice, meanwhile, it is common to apply energy reductions when supplementing diets with fat sources, such as lysolecithin, in order to maintain bird performance at reduced cost. To date, few scientific studies have compared the application of lysolecithins on standard poultry diets with reformulated diets, where fats and oils have been reduced or substituted. Therefore, the aim of this study was to evaluate the efficacy and consistency of lysolecithin to improve performance in standard or reformulated broiler diets across a large dataset of published trials.
Materials and methods
All experiments were conducted in accordance with all relevant institutional and national animal care guidelines at each participating experimental station. The commercial lysolecithin (LCL) products used in all studies were either Lysoforte® Dry (Kemin Animal Nutrition and Health, Des Moines, IA) or Lysoforte® Booster Dry (Kemin Animal Nutrition and Health, Des Moines, IA). To account for differences in product composition, dose in feed was converted into the equivalent dose of lysolecithin (g LCL/tonne feed).
Animal trials
From the available trial reports, data from 33 separate worldwide experiments were collated to assess to efficacy of lysolecithin-derived biosurfactants in broiler diets on growth performance (). The following selection criteria were used, as per Wealleans et al. (Citation2015), whereby trials must have included: (a) parallel control treatment, (b) appropriate level of replication per treatments, and (c) there were no unreliable parameters (disease or husbandry challenge, high mortality, etc). The lysolecithins must have been fed for the entire study period, and the inclusion rates reported, and the study period must have started at hatch and lasted until a commercially relevant slaughter age (≥35 days). Bodyweight gains and feed intakes must have been recorded throughout the whole trial period (initial and final weights, total feed intake per pen), and information must have been available on dietary fat/oil type and inclusion, diet density (kcal/kg) and the cereal base of the diet.
Table 1. Experimental design of trials used in the analysis.
Within each study, information on year, location, replicate number and size and diet was recorded for each treatment mean used. Experimental design parameters of the constituent trials in this analysis is presented in . To standardise dietary information, diet density, inclusion level and the U:S ratio of added fats were calculated based on diet composition from each trial. Diet density categories was determined from the finisher diet, as follows: diets with energy <3100 kcal/kg were assigned a density category of 1, diets with 3100 to 3200 kcal/kg were assigned a density category of 2, and diets with >3200 kcal/kg were assigned a density category of 3.
Treatment means from 33 separate performance trials across a range of husbandry conditions from 13 different countries were collated in a database, providing 66 means across three lysolecithin inclusion levels (0, 125 and 250 g LPL/t feed) and diet types. Of these, 16 were ‘on top’ trials and 17 used reformulation protocols. Reformulation protocols differed between studies, but largely applied reductions in metabolisable energy through the reduction of added fat to the experimental diets. Differences in added fat between the control and supplemented diets for reformulation trials are shown in .
To account for differing study lengths, bodyweight gain and feed intake were expressed as average daily gain (ADG) and average daily feed intake (ADFI). To calculate ADFI, feed added and wastage (as reported) was recorded daily throughout the experimental period. Feed efficiency (FCR) for each individual mean was standardised at 2.5 kg body weight (FCRc), as per the Aviagen guidelines using the equation:
Energy recovery by lysolecithin-supplemented birds was calculated by assigning a 30 kcal (assuming an average dietary energy density of 3000 kcal/kg) value to every point difference in FCR between control and supplemented treatment means from the same trial, as per the following equation:
In all cases, the experimental diets were provided ad libitum throughout the experimental period. Birds were fed treatment diets from day of hatch until slaughter. All birds were monitored daily for abnormalities and clinical signs of sickness as well as the availability of feed and water. Dead birds, when they occurred, were recorded and mortality was calculated by subtracting the number of birds at the end of the experiment from the number of birds at the beginning of the experiment. Mortality was expressed both as an absolute number and as a percentage.
Statistics
To understand the dataset, an initial round of descriptive statistics was undertaken. Outlier removal was conducted using jackknife distances (Tukey Citation1958; Miller Citation1974): data rows where the jackknife distances for the multidimensional mean of improvements in body weight change and feed intake were more than 2.5 SD were excluded from the data set. However, no data fulfilled these criteria and therefore all available data was included in the analyses. The experimental unit was considered to be treatment means from individual studies.
To quantify the effect of lysolecithin dose on parameters of interest, performance data were analysed using the REML method; trial was considered as a random effect, as this accounted for the underlying heterogeneity between studies (Lean et al. Citation2009). The distribution of random effects and residual errors was assumed to be normal in all models. Means separation was achieved using Tukey’s honest significant difference test in the Fit Model platform of JMP 13 (SAS Inst. Inc., Cary, NC) and significance was determined at P < 0.05. Probability levels of P < 0.1 was taken to represent a near-significant trend.
Results
Across the whole dataset, the average added fat content in control diets was 4.42%, as shown in . In trials without reformulation, the average added fat content of the diets ranged from 1.15 to 5.60%, with an average of 3.99%. In the reformulated diet trials, the average control diet contained 5.05% added fat, with a range from 2.50 to 7.00%, while the reformulated diets had 3.7% added fat – a difference of 1.35%. Reductions in added fat in the reformulated diets ranged from 0.5 to 2.64%. Overall, average added fat in the reformulated diets was 4.19%. Similarly, the ratio of U:S in added fats in the control diets ranged from 0.94 to 7.65. A U:S ratio of 0.94 was representative of a diet containing only tallow or palm oil as a source of added fat, while a U:S ratio of 7.65 was representative of a diet containing sunflower oil as a source of added fat. Overall, neither ADG or FCRc were significantly affected by either U:S ratio (ADG P = 0.8627; FCRc P = 0.1158) or dietary added fat (ADG = 0.3620; FCRc P = 0.1855).
Table 2. Dataset descriptions for trials used in this analysis.
The main effects of LCL supplementation on broiler performance are shown in . Lysolecithin supplementation had no significant effect on ADG or ADFI in ‘on top’ trials. However, lysolecithin supplementation at any dose resulted in reductions in FCRc in 68.75% of the ‘on top’ trials. The average reduction in FCRc was 2.15 points. These findings were in line with the qualitative analysis, where LCL at 250 g/t significantly reduced FCRc compared to unsupplemented birds (1.599 for the control vs. 1.576 for 250 g/t LCL; P < 0.05) while LCL at 125 g/t tended to reduce FCRc compared to the control. These improvements in FCRc were calculated to be equivalent to 52.73 and 69.79 kcal/kg diet for 125 g/t and 250 g/t, respectively. There were no significant effects of U:S ratio on BW (P = 0.1564), FCRc (P = 0.5029), or energy recovery (P = 0.5029) response to overall lysolecithin supplementation when applied on top on existing diet formulations.
Table 3. Effect of lysolecithin supplementation on whole life growth performance in broiler chickens.
For reformulated trials, there were no significant effects of LCL supplementation on any of the measured performance parameters, though there was a tendency for average daily gain to be reduced in LCL supplemented birds compared to the control (−0.48 g/day for 125 g/t LCL, −0.95 g/day for 250 g/t LCL; P < 0.1). There was a significant increase in calculated energy recovery with both doses of LCL (60.17 kcal for 125 g/t LCL, 52.98 kcal for 250 g/t LCL), although this was less than the average dietary energy reduction of 57.88 and 73.11 kcal applied, respectively. However, the lack of significant difference in FCR between treatments in reformulated diets indicated that birds supplemented with LCL at 125 and 250 g/t recovered performance despite the energy reductions used.
Discussion
In this study, data were combined from 33 performance trials to assess the effects of lysolecithin supplementation on broiler growth performance. When lysolecithins at 125 and 250 g/t were applied on top of standard diets, significant responses were consistently seen in FCR standardised at 2.5 kg final BW (FCRc), as shown in . However, the magnitude of effect on FCR following on top supplementation with lysophospholipids varied between studies, ranging from 8 points in reduction to a 4 point increase vs. an unsupplemented control. This variation was in line with the response seen in other published studies (Chen et al. Citation2019; Jansen et al. Citation2015; Zaefarian et al. Citation2015), and is likely due to the inherent variability in growth response to nutritional additives. Even when assessing much larger datasets than currently presented, models quantifying factors responsible for bird response to phytase enzyme addition could only account for 64–72% of total variation (Rosen Citation2006). By assessing the wider dataset, overarching trends can be seen in the ability of LCL supplementation to reduce FCR in broilers. This was in line with the findings of Zampiga et al. (Citation2016) and Melegy et al. (Citation2010), who reported 2% and 5% improvements in FCR, respectively, when supplementing LCL on top of existing formulations. Similarly, in reformulated diets, where the aim of supplementation was to recover the reduction in energy between the standard control and the reformulated diet by lysolecithin supplementation, there were no significant effects of treatment on growth performance. These results agree with the findings of Papadopoulos et al. (Citation2018), who found that supplementation with 250 g/t LCL in feed could maintain broiler performance in diets that had been reduced by 64.5 kcal ME/kg – a similar energy reduction to the average applied in this analysis’ constituent trials. Chen et al. (Citation2019) found that there were significant growth responses to supplemental LCL at 250, 500 and 750 g/t, but the results did not show a clear linear dose response.
Figure 1. Forest plot demonstrating the effect of on top lysolecithin supplementation in broiler diets on FCR standardised at 2.5 kg BW.
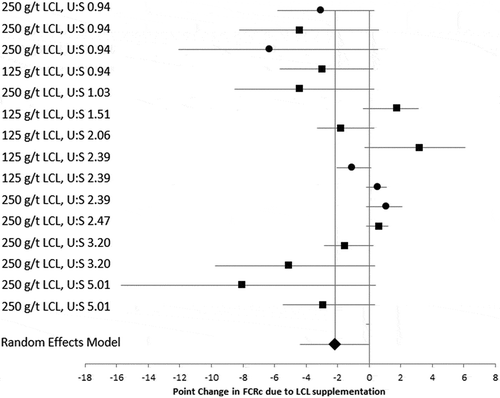
Surprisingly, and in contrast to previously published literature (Jansen et al. Citation2015) there was no statistical link in this dataset between the type of dietary fat or oil used and the growth response to the addition of lysolecithin. In addition, the U:S ratio had little influence on the magnitude of response to LCL supplementation, particularly when applied on top of existing diets. This finding was supported by Khonyoung et al. (Citation2015), who reported no significant interactions between lysolecithin supplementation and fat source. Similarly, Zhang et al. (Citation2011) found no significant interactions between fat sources (soybean oil, tallow and poultry fat) and lysophosphatidylcholine on nutrient digestibility in broilers. Conversely, Jansen et al. (Citation2015), found that improvements in nutrient digestibility following LCL supplementation were highly dependent on fat source, and greater in feeds containing higher levels of saturated fats. In Jansen et al. (Citation2015) the fats studied were pure soy oil and pig fat. Under experimental conditions, where the quality of the feed ingredients is good, digestibility and energy values of these purified fat sources is largely determined by the U:S ratio and highly saturated fat sources are considered to be less digestible and less energetically available by animals (Zhang et al. Citation2011; Wiseman and Salvador Citation1991). By contrast, the current dataset contained trials in which the effect of LCL in diets formulated with diversified fat sources was assessed, including multiple studies with acid oils. In general, acid oils have high U:S ratios but, given their chemical composition, are poorly digested by animals. In addition, in this analysis, only two trials where LCL was applied ‘on top’ contained vegetable oils, which typically have a high U:S ratio, potentially restricting assessment of a response. Given the contradictions in the literature and in this analysis, it is clear that the relationship between lysolecithin supplementation and dietary fat source and U:S ratio has not been conclusively described and merits further research.
A number of authors have found significant differences in AMEn between animal fats and soybean oil (Jansen et al. Citation2015; Zaefarian et al. Citation2015; Zhang et al. Citation2011), and have associated this with differences in the degree of saturation between the fat types (Wiseman and Salvador Citation1991). However, when looking at the effect of fat saturation on performance, Firman et al. (Citation2008), among others, reported few statistical differences between fat sources. This was more in line with the results of the current work, where the U:S ratio of the diet had little impact on performance or calculated energy recovery from the diets.
In conclusion, this study used information from a large underlying dataset to show that the addition of lysolecithin at 125 g/t and above to broiler diets could consistently improve growth performance across a range of husbandry conditions, basal cereals and fat sources. Improvements can be in the region of up to 70 kcal/kg equivalent of energy recovered in feed compared to unsupplemented birds, depending on the circumstance. When applied on top of a standard diet formulation, LCL can improve feed efficiency up to the limit of the genetic potential of the bird, whilst, when applied alongside energy reductions, maintains growth performance.
Disclosure statement
No potential conflict of interest was reported by the authors.
References
- Allahyari-Bake, S., and R. Jahanian. 2016. “Effects of Dietary Fat Source and Supplemental Lysophosphatidylcholine on Performance, Immune Responses, and Ileal Nutrient Digestibility in Broilers Fed Corn/soybean Meal-or Corn/wheat/soybean Meal-based Diets.” Poultry Science 96: 1149–1158.
- Al-Marzooqi, W., and S. Leeson. 1999. “Evaluation of Dietary Supplements of Lipase, Detergent, and Crude Porcine Pancreas on Fat Utilization by Young Broiler Chicks.” Poultry Science 78: 1561–1566. doi:10.1093/ps/78.11.1561.
- Arouri, A., and O. G. Mouritsen. 2013. “Membrane-perturbing Effect of Fatty Acids and Lysolipids.” Progress in Lipid Research 52: 130–140. doi:10.1016/j.plipres.2012.09.002.
- Brautigan, D. L., R. Li, E. Kubicka, S. D. Turner, J. S. Garcia, M. L. Weintraut, and E. A. Wong. 2017. “Lysolecithin as Feed Additive Enhances Collagen Expression and Villus Length in the Jejunum of Broiler Chickens.” Poultry Science 1; 96 (8): 2889–2898. doi:10.3382/ps/pex078.
- Chen, C., B. Jung, and W. K. Kim. 2019. “Effects of Lysophospholipid on Growth Performance, Carcass Yield, Intestinal Development, and Bone Quality in Broilers.” Poultry Science pez111. doi:10.3382/ps/pez111.
- Firman, J. D., A. Kamyab, and H. Leigh. 2008. “Comparison of Fat Sources in Rations of Broilers from Hatch to Market.” International Journal of Poultry Science 7: 1152–1155. doi:10.3923/ijps.2008.1152.1155.
- Hakansson, J. 1974. “Factors Affecting the Digestibility of Fats and Fatty Acids in Chicks and Hens.” Swedish Journal of Agricultural Research 4: 33–47.
- Jansen, M., F. Nuyens, J. Buyse, S. Leleu, and L. Van Campenhout. 2015. “Interaction between Fat Type and Lysolecithin Supplementation in Broiler Feeds.” Poultry Science 94: 2506–2515. doi:10.3382/ps/pev181.
- Joshi, A., S. G. Paratkar, and B. N. Thorat. 2006. “Modification of Lecithin by Physical, Chemical and Enzymatic Methods.” European Journal of Lipid Science and Technology 108: 363–373. doi:10.1002/(ISSN)1438-9312.
- Khonyoung, D., K. Yamauchi, and K. Suzuki. 2015. “Influence of Dietary Fat Sources and Lysolecithin on Growth Performance, Visceral Organ Size, and Histological Intestinal Alteration in Broiler Chickens.” Livestock Science 176: 111–120. doi:10.1016/j.livsci.2015.03.011.
- Lean, I. J., A. R. Rabiee, T. F. Duffield, and I. R. Dohoo. 2009. “Invited Review: Use of Meta-analysis in Animal Health and Reproduction: Methods and Applications.” Journal of Dairy Science 92: 3545–3565. doi:10.3168/jds.2009-2140.
- Liu, D., and F. Ma. 2011. “Soybean Phospholipids.” In Recent Trends for Enhancing the Diversity and Quality of Soybean Products, edited by D. Krezhova, 483–500. Rijeka, Croatia: Intech.
- Lundbaek, J. A., and O. S. Andersen. 1994. “Lysophospholipids Modulate Channel Function by Altering the Mechanical Properties of Lipid Bilayers.” The Journal of General Physiology 104: 645–673. doi:10.1085/jgp.104.4.645.
- Maingret, F., A. J. PateL, F. Lesage, M. Lazdunski, and E. Honoré. 2000. “Lysophospholipids Open the Two-pore Domain Mechanogated K(+) Channels TREK-1 and TRAAK.” The Journal of Biological Chemistry 275: 10128–10133. doi:10.1074/jbc.275.14.10128.
- Maisonnier, S., J. Gomez, A. Brée, C. Berri, E. Baéza, and B. Carré. 2003. “Effects of Microflora Status, Dietary Bile Salts and Guar Gum on Lipid Digestibility, Intestinal Bile Salts, and Histomorphology in Broiler Chickens.” Poultry Science 82: 805–814. doi:10.1093/ps/82.5.805.
- Mandalari, G., K. Adel-Patient, V. Barkholt, C. Baro, L. Bennett, M. Bublin, and E. N. C. Mills. 2009. “In Vitro Digestibility of Betacasein and Beta-lactoglobulin under Simulated Human Gastric and Duodenal Conditions: A Multi-laboratory Evaluation.” Regulatory Toxicology and Pharmacology 55: 372–381. doi:10.1016/j.yrtph.2009.08.010.
- Melegy, T., N. F. Khaled, R. El-Bana, and H. Abdellatif. 2010. “Dietary Fortification of a Natural Biosurfactant, Lysolecithin in Broilers.” African Journal of Agricultural Research 5: 2886–2892.
- Miller, R. G. 1974. “The Jackknife – A Review.” Biometrika 61: 1–15.
- Noy, Y., and D. Sklan. 1995. “Digestion and Absorption in the Young Chick.” Poultry Science 74: 366–373. doi:10.3382/ps.0740366.
- Papadopoulos, G. A., T. Poutahidis, S. Chalvatzi, M. Di Benedetto, A. Hardas, V. Tsiouris, I. Georgopoulou, G. Arsenos, and P. D. Fortomaris. 2018. “Effects of Lysolecithin Supplementation in Low-energy Diets on Growth Performance, Nutrient Digestibility, Viscosity and Intestinal Morphology of Broilers.” British Poultry Science 59: 232–239. doi:10.1080/00071668.2018.1423676.
- Pesti, G. M., R. I. Bakalli, M. Qiao, and K. G. sterling. 2002. “A Comparison of Eight Grades of Fat as Broiler Feed Ingredients.” Poultry Science 81: 382–390. doi:10.1093/ps/81.3.382.
- Polin, D. 1980. “Increased Absorption of Tallow with Lecithin.” Poultry Science 59: 1652. (Abstr).
- Polycarpo, G. V., M. F. C. Burbarelli, A. C. P. Carão, C. E. B. Merseguel, J. C. Dadalt, S. R. L. Maganha, R. L. M. Sousa, V. C. Cruz-Polycarpo, and R. Albuquerque. 2016. “Effects of Lipid Sources, Lysophospholipids and Organic Acids in Maize-based Broiler Diets on Nutrient Balance, Liver Concentration of Fat-soluble Vitamins, Jejunal Microbiota and Performance.” British Poultry Science 57: 788–798. doi:10.1080/00071668.2016.1219019.
- Rosen, G. D. 2006. “Holo-analysis.” Poultry Science 85 (6): 957–959. doi:10.1093/ps/85.6.957.
- Soares, M., and C. J. Lopez-Bote. 2002. “Effects of Dietary Lecithin and Fat Unsaturation on Nutrient Utilisation in Weaned Piglets.” Animal Feed Science and Technology 95: 169–177. doi:10.1016/S0377-8401(01)00324-8.
- Sugawara, T., M. Kushiro, H. Zhang, and E. Nara. 2001. “Nutrient Interactions and Toxicity Lysophosphatidylcholine Enhances Carotenoid Uptake from Mixed Micelles by Caco-2 Human Intestinal Cells.” Journal of Nutrition 131: 2921–2927. doi:10.1093/jn/131.11.2921.
- Tukey, J. W. 1958. “Bias and Confidence in Not Quite Large Samples.” Annals of Mathematical Statistics 29: 614.
- Van Nieuwenhuyzen, W., and M. C. Tomás. 2008. “Update on Vegetable Lecithin and Phospholipid Technologies.” European Journal of Lipid Science and Technology 110: 472–486. doi:10.1002/(ISSN)1438-9312.
- Wealleans, A. L., R. M. Bold, Y. Dersjant-Li, and A. Awati. 2015. “The Addition of a Buttiauxella Sp. Phytase to Lactating Sow Diets Deficient in Phosphorus and Calcium Reduces Weight Loss and Improves Nutrient Digestibility.” Journal of Animal Science 93: 5283–5290. doi:10.2527/jas.2015-9317.
- Wendel, A. 1995. “Lecithin.” In Kirk–Othmer Encyclopedia of Chemical Technology, edited by M. Howe-Grant, Vol. 15, 192–209. 14th ed. New York, NY: Wiley.
- Wiseman, J., and F. Salvador. 1991. “The Influence of Free Fatty Acid Content and Degree of Saturation on the Apparent Metabolizable Energy Value of Fats Fed to Broilers.” Poultry Science 70: 573–582. doi:10.3382/ps.0700573.
- Zaefarian, F., L. F. Romero, and V. Ravindran. 2015. “Influence of High Dose of Phytase and an Emulsifier on Performance, Apparent Metabolisable Energy and Nitrogen Retention in Broilers Fed on Diets Containing Soy Oil or Tallow.” British Poultry Science 56: 590–597. doi:10.1080/00071668.2015.1067878.
- Zampiga, M., A. Meluzzi, and F. Sirri. 2016. “Effect of Dietary Supplementation of Lysophospholipids on Productive Performance, Nutrient Digestibility and Carcass Quality Traits of Broiler Chickens.” Italian Journal of Animal Science 15: 521–528. doi:10.1080/1828051X.2016.1192965.
- Zhang, B., L. Haitao, D. Zhao, Y. Guo, and A. Barri. 2011. “Effect of Fat Type and Lysophosphatidylcholine Addition to Broiler Diets on Performance, Apparent Digestibility of Fatty Acids, and Apparent Metabolizable Energy Content.” Animal Feed Science and Technology 163: 177–184. doi:10.1016/j.anifeedsci.2010.10.004.