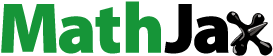
ABSTRACT
1. Phosvitin, a major phosphoprotein found in egg yolk, has strong antioxidant activity. Activation of elastase, collagenase, and hyaluronidase by reactive oxygen species are related to the degradation of ECM and skin aging. The objective of this study was to determine the anti-elastase and anti-hyaluronidase activity of phosvitin.
2. Elastase from porcine pancreas and hyaluronidase from bovine testes were used to study the inhibitory activity of phosvitin. To elucidate the mechanism of enzyme inhibition, a Lineweaver-Burk plot was constructed.
3. Phosvitin inhibited elastase and hyaluronidase activity in a dose-dependent manner. The IC50 value of phosvitin was 31.6 μg/ml and 1,270 μg/ml against elastase and hyaluronidase, respectively. The analysis of elastase and hyaluronidase kinetics indicated that the apparent Michaelis constant (appKm) was increased by phosvitin but the Vmax value was not affected.
4. In conclusion, phosvitin exhibited competitive inhibitory activity against elastase and hyaluronidase. Thus, phosvitin could be used as a natural anti-aging agent in the cosmetics industry.
Introduction
Skin is constantly exposed to the external environment, and thus can be damaged more easily than other organs. The extracellular matrix (ECM) is the outermost component of skin and acts as a barrier to external stimuli. ECM is composed of various components, such as laminin, collagen, elastin, and hyaluronic acid (Nystrom and Bruckner-Tuderman Citation2019). Solar UV radiation, one of the strongest stimuli to the skin, induces production of reactive oxygen species (ROS). ROS induce activation of enzymes that are closely related to the degradation of ECM, such as elastase, collagenase, and hyaluronidase (Wittenauer et al. Citation2015). These enzymes are known to be involved in skin aging (Thring et al. Citation2009; Kumud and Sanju Citation2018).
Elastin is a protein in the ECM that confers elasticity to various body tissues, such as skin, lungs, ligaments, and arteries (Huertas et al. Citation2018). Elastin is cleaved by elastase, which is a member of the chymotrypsin family, and is involved in the degradation of ECM components, such as collagen, fibronectin, and other ECM proteins (Thring et al. Citation2009). Elastase can activate matrix metalloproteinase (MMP) precursors that are associated with the degradation of ECM (Wittenauer et al. Citation2015). Therefore, inhibitors of elastase can prevent degradation of the ECM.
Hyaluronic acid (HA), known as hyaluronan, is a polymer composed of repeating units of glucuronic acid and N-acetylglucosamine connected by β-linkages (Lee and Spicer Citation2000). This high-molecular-weight polymer is a component of ECM that provides viscoelasticity, and plays a crucial role in preventing skin aging by reducing wrinkles and keeping the skin smooth and hydrated (Necas et al. Citation2008; Miri et al. Citation2014). Hyaluronidase is a mucopolysaccharide-degrading enzyme that hydrolyses the β-1,4-glycosidic bonds of HA and induces a decrease in its viscosity (Necas et al. Citation2008; Moon et al. Citation2009; Lee et al., Citation2018). Thus, elastase and hyaluronidase inhibitors can be useful in preventing aging in skin.
Phosvitin is an egg yolk protein, and more than 50% of its total amino acids is serine (Byrne et al. Citation1984; Samaraweera et al. Citation2011). Of these, more than 90% of serine residues are phosphorylated, indicating that phosvitin binds to 80% of the phosphorus in the egg yolk (Taborsky and Mok Citation1967). This high phosphorus content facilitates various functional activities, whereby phosvitin exhibits anti-osteoporosis effects by stimulating the proliferation, differentiation, and mineralisation of MC3T3-E1 cells (Jie et al. Citation2018). Phosvitin is reported that have an anti-tyrosinase activity in B16F10 melanoma cells (Jung et al. Citation2012), act as an antioxidant (Lu and Baker Citation1986), and has anti-genotoxic (Moon et al. Citation2014), anti-microbial (Khan et al. Citation2000), and immunomodulatory activity (Lee et al. Citation2017) because of its strong metal-chelating activity. However, the anti-hyaluronidase and anti-elastase activities of phosvitin have not been studied yet.
The aim of this study was to evaluate the inhibitory activities of phosvitin on elastase and hyaluronidase. In addition, the underlying mechanisms of inhibition were identified using enzyme kinetics.
Materials and methods
Sample and reagents
Phosvitin was prepared from chickens egg yolk according to the method previously described by Lee et al. (Citation2014). Briefly, egg yolk was homogenised using two volumes of cold DW and centrifuged at 3,400 × g for 30 min. After this step, the precipitant was homogenised with four volumes of 0.05 N NaOH solution containing 10% NaCl. Then, the pH was adjusted to 4.0 and centrifuged at 3,400 × g for 30 min. The solution was heat-treated at 80°C for 30 min and centrifuged to remove impurities. The supernatant was collected, desalted using ultrafiltration (membrane filter cut-off size: 10 kDa, GE healthcare Bio-Sciences Corp., Piscataway, NJ, USA), and then lyophilised using a freeze-dryer. The purity and yield of isolated phosvitin was 97.2 and 98.7%, respectively. For chemical analysis of phosvitin, SDS-PAGE analysis was conducted ().
Figure 1. SDS-PAGE (15% gel) band pattern of phosvitin. Lane 1: marker, lane 2: Standard phosvitin (1 mg/ml), lane 3: isolated phosvitin (1 mg/ml).
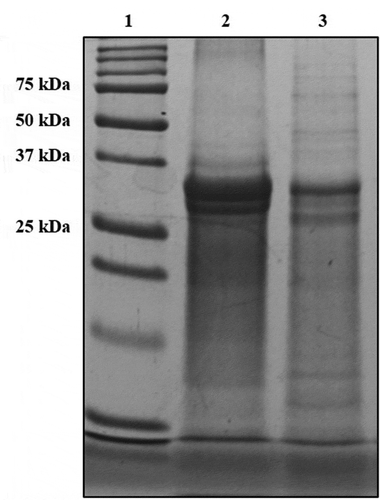
Elastase was extracted from porcine pancreas (EC. 3.4.21.36), hyaluronidase was obtained from bovine testes (EC. 3.2.1.35), N-Succinyl-tri-L-alanine-4-nitroanilide, hyaluronic acid, and p-dimethyl-aminobenzaldehyde were purchased from Sigma Chemical Co. (St. Louis, MO). All other organic solvents and chemicals used were of analytical grade.
Elastase inhibition assay
The anti-elastase activity was evaluated according to the method previously described by Wittenauer et al. (Citation2015) with some modifications. Elastase obtained from porcine pancreas (1 unit/ml, EC. 3.4.21.36, Sigma Chemical Co.) and its substrate (N-succinyl-tri-L-alanine-4-nitroanilide, 0.6 mM, Sigma Chemical Co.) were dissolved in 2 mM Tris buffer (pH 8.0). Then, various concentrations of phosvitin (30 μl, 7.81–2,000 μg/ml distilled water) and 10 μl of enzyme solution were mixed with 100 μl of buffer in 96-well plate. The plate was pre-incubated at 25°C for 20 min. Then, 40 μl of the substrate solution was added to each well. The anti-elastase activity of phosvitin was measured by continuously monitoring the absorbance at 410 nm for 20 min using a microplate reader (Multiskan GO, Thermo Scientific, Waltham, MA, USA). The initial velocities were calculated from the slope of absorbance change during the first 10 min of the reaction. The control used distilled water instead of phosvitin solution. The anti-elastase activity was calculated according to the following equation:
IC50 values were determined using the software Softmax Pro (Molecular Devices, San Jose, CA, USA).
Hyaluronidase inhibition assay
The anti-hyaluronidase activity was evaluated as described by Moon et al. (Citation2009) with modifications. Hyaluronidase obtained from bovine testis (EC. 3.2.1.35, Sigma Chemical Co.) (100 μl, 3,000 units/ml) was mixed with 100 μl of different concentrations of phosvitin (500, 1,000, 1,500, and 2,000 μg/ml) and treated with 500 μl of hyaluronic acid (5 mg/ml) dissolved in 0.1 M acetate buffer (pH 3.5). After 40 min of incubation at 37°C, 2 ml of p-dimethyl-aminobenzaldehyde was added and the optical density of mixtures was measured at 570 nm in a microplate reader. The control used distilled water instead of phosvitin solution. The anti-hyaluronidase activity was calculated using the following equation:
Where, A control refers to the absorbance of distilled water and A sample refers to the absorbance of sample at 570 nm. IC50 values were determined using the software Softmax Pro.
Kinetics of enzyme inhibition
Enzyme kinetics is an important tool to investigate the mechanism of catalysis. The kinetic parameters were determined using the Lineweaver-Burk plot method. The reaction conditions of elastase and hyaluronidase were the same as those mentioned above. Various substrate (elastase: 0.125, 0.25, and 0.5 mM; hyaluronidase: 0.5, 1, and 2 mg/ml) and phosvitin concentrations (elastase: 0, 25, and 50 μg/ml; hyaluronidase: 0, 125, and 250 μg/ml) were used for the kinetic study. From Lineweaver-Burk plot graph, Vmax and appKm (apparent Michaelis constant) values were obtained as the Y- and X-axis intercepts, respectively (Y-axis intercept: 1/Vmax; X-axis intercept: −1/appKm) (Kim et al. Citation2018).
Statistical analysis
All results are presented as means and standard deviations from the three independent experiments. Differences between means from multiple groups were analysed as a one-way analysis of variance (ANOVA), followed by the Duncan’s multiple range test (P < 0.05). All calculations were performed using SPSS for Windows version 18.0 (SPSS Inc., Chicago, IL, USA).
Results and discussion
The inhibitory activity of phosvitin on elastase and hyaluronidase is shown in . Phosvitin inhibited the activities of elastase and hyaluronidase in a dose-dependent manner. However, phosvitin exhibited higher inhibitory activity against elastase than hyaluronidase. At 500 μg/ml concentration, phosvitin showed > 90% (92.77 ± 0.45%) inhibitory activity against elastase, and the amount needed for 50% inhibition (IC50) was 31.6 μg/ml (). On the other hand, much higher level of phosvitin was needed to inhibit hyaluronidase than elastase activity, whereby phosvitin at 2,000 μg/ml level inhibited hyaluronidase activity by 92.47 ± 2.14%, while phosvitin concentrations of 1,500, 1,000, and 500 μg/ml inhibited hyaluronidase activity by 65.91 ± 4.73%, 34.11 ± 5.61%, and 2.54 ± 5.55%, respectively. The IC50 value of phosvitin on hyaluronidase was 1,270 μg/ml ().
Figure 2. Inhibition of in vitro elastase activity (a) and hyaluronidase activity (b) by phosvitin. Values are expressed as the mean ± standard deviation. Different letters among samples indicate significant differences by Duncan’s multiple range test (P < 0.05) (n = 3). IC50 values were determined using the software Softmax Pro.
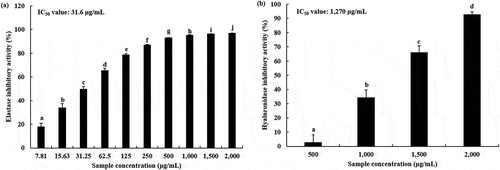
To confirm the mechanism of enzyme inhibition by phosvitin, an enzyme kinetics study was conducted (, ). Lineweaver-Burk plots revealed that the apparent Michaelis constant (appKm) of phosvitin was increased (elastase: 1.807, 3.700, and 5.373 mM substrate; hyaluronidase: 0.789, 0.897, and 1.068 mg/ml substrate). This indicated that phosvitin affected the affinity of enzyme to the substrate. However, the Vmax values did not change (elastase: 0.075, 0.074, and 0.074 ΔA/min; hyaluronidase: 0.029, 0.028, and 0.028 mM/min). These patterns were similar to a competitive inhibition model (Kakizaki et al. Citation2015; Kim et al. Citation2018), which indicated that phosvitin acted as a competitive-type inhibitor of elastase and hyaluronidase. These results suggested that phosvitin interacted with the active sites of elastase and hyaluronidase and interfered with the substrate-binding to the enzyme.
Table 1. Values of appKm, Vmax, and slope of Lineweaver-Burk plot on the inhibition of elastase and hyaluronidase by phosvitin.
Figure 3. Lineweaver-Burk plot of elastase (a) and hyaluronidase (b) inhibition by phosvitin. (a) ●: 0 μg/ml of phosvitin, ■: 25 μg/ml of phosvitin, and ▲: 50 μg/ml of phosvitin, (b) ●: 0 μg/ml of phosvitin, ■: 125 μg/ml of phosvitin, and ▲: 250 μg/ml of phosvitin.
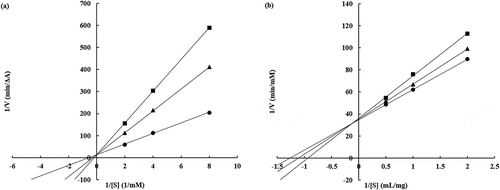
Many competitive inhibitors of elastase (e.g., isoflavones; Kim et al. Citation2018) and hyaluronidase (e.g. oligosaccharides and flavonoids; Kakizaki et al. Citation2015; Kuppusamy et al. Citation1990) have been reported. These inhibitors bind to the active site of enzymes where they block substrate attachment or alter enzyme conformation (Khueychai et al. Citation2018).
Many researchers have studied natural candidates that can be used as anti-aging agents in the cosmetics industry. They studied the anti-elastase and anti-hyaluronidase activities because these inhibitors are known to play crucial roles in anti-aging of skin by protecting the degradation of elastin and HA, which are ECM components (Kumud and Sanju Citation2018). Following the degradation of elastin, the skin lost its elasticity and showed wrinkling and sagging (Liyanaarachchi et al. Citation2018). HA can hold large amounts of moisture (approximately 6 l of water in 1 g), indicating that the inhibitors of hyaluronidase can effectively regulate skin moisturisation (Jegasothy et al. Citation2014).
Elastase is a member of the serine proteases. It has three subunits bound with calcium ions and a cofactor (Sadeghi-Kaji et al. Citation2019). Phosvitin exhibits a strong chelating activity to metals such as Mg2+, Ca2+, Mn2+, Co2+ and Cu2+ ions (Zhang et al. Citation2016). This is probably why phosvitin exhibits inhibitory activity against elastase. Moreover, phosvitin has a hydrophobic region composed of relatively rich hydrophobic amino acids (Byrne et al. Citation1984). Sunitha et al. (Citation2013) reported that hydrophobic parts of hyaluronidase play a crucial role in facilitating interactions with the thiol group of glutathione. A previous study showed that the hydrophobic properties of phosvitin are important factors in inhibiting tyrosinase by interacting with the hydrophobic region of the enzyme (Jung et al. Citation2012). Therefore, phosvitin can bind to the active site of hyaluronidase and enabled strong hydrophobic interactions. Jung et al. (Citation2012) reported that phosvitin exhibited inhibitory activity against tyrosinase both in vitro and ex vivo. Phosvitin showed inhibition of mushroom tyrosinase. Furthermore, it inhibited the expression of tyrosinase and its related proteins 1, 2 (TRP-1, TRP-2) in B16F10 melanoma cells. This suggested that phosvitin has a high potential to be used as a whitening agent in the cosmetics industry.
In conclusion, phosvitin from egg yolk exhibited inhibitory activity against elastase and hyaluronidase that are related to skin aging. The inhibition model of phosvitin involves competitive inhibition, indicating that phosvitin combined with active sites of elastase and hyaluronidase and interfered with the formation of enzyme-substrate complex. These results suggested that phosvitin could be used as a natural anti-aging agent in the cosmetics industry.
Disclosure statement
No potential conflict of interest was reported by the authors.
Additional information
Funding
References
- Byrne, B. M., A. D. V. Schip, J. A. M. Vandeklundert, A. C. Arnberg, M. Gruber, and G. Ab. 1984. “Amino-Acid-Sequence of Phosvitin Derived from the Nucleotide-Sequence of Part of the Chicken Vitellogenin Gene.” Biochemistry 23: 4275–4279. doi:10.1021/bi00314a003.
- Huertas, A. C. M., C. E. H. Schmelzer, C. Luise, W. Sippl, M. Pietzsch, W. Hoehenwarter, and A. Heinz. 2018. “Degradation of Tropoelastin and Skin Elastin by Neprilysin.” Biochimie 146: 73–78. doi:10.1016/j.biochi.2017.11.018.
- Jegasothy, S. M., V. Zabolotniaia, and S. Bielfeldt. 2014. “Efficacy of a New Topical Nano-Hyaluronic Acid in Humans.” Journal of Clinical and Aesthetic Dermatology 7: 27–29.
- Jie, Y., X. Li, Z. Cai, M. Ma, Y. Jin, D. U. Ahn, and X. Huang. 2018. “Phosphorylation of Phosvitin Plays a Crucial Effects on the Protein Induced Differentiation and Mineralization of Osteoblastic MC3T3-E1 Cells.” International Journal of Biological Macromolecules 118: 1848–1854. doi:10.1016/j.ijbiomac.2018.07.029.
- Jung, S., D. H. Kim, J. H. Son, K. Nam, D. U. Ahn, and C. Jo. 2012. “The Functional Property of Egg Yolk Phosvitin as a Melanogenesis Inhibitor.” Food Chemistry 135: 993–998. doi:10.1016/j.foodchem.2012.05.113.
- Kakizaki, I., H. Koizumi, F. Chen, and M. Endo. 2015. “Inhibitory Effect of Chondroitin Sulfate Oligosaccharides on Bovine Testicular Hyaluronidase.” Carbohydrate Polymers 121: 362–371. doi:10.1016/j.carbpol.2014.11.071.
- Khan, M. A. S., S. Nakamura, M. Ogawa, E. Akita, H. Azakami, and A. Kato. 2000. “Bactericidal Action of Egg Yolk Phosvitin against Escherichia Coli under Thermal Stress.” Journal of Agricultural and Food Chemistry 48: 1503–1506. doi:10.1021/jf990700r.
- Khueychai, S., N. Jangpromma, K. Choowongkomon, A. Joompang, S. Daduang, M. Vesaratchavest, W. Payoungkiattikun, S. Tachibana, and S. Klaynongsruang. 2018. “A Novel ACE Inhibitory Peptide Derived from Alkaline Hydrolysis of Ostrich (Struthio camelus) Egg White Ovalbumin.” Process Biochemistry 73: 235–245. doi:10.1016/j.procbio.2018.07.014.
- Kim, J. Y., Y. Wang, Z. Uddin, Y. H. Song, Z. P. Li, J. Jenis, and K. H. Park. 2018. “Competitive Neutrophil Elastase Inhibitory Isoflavones from the Roots of Flemingia Philippinensis.” Bioorganic Chemistry 78: 249–257. doi:10.1016/j.bioorg.2018.03.024.
- Kumud, M., and N. Sanju. 2018. “In-Vitro Evaluation of Antioxidant, Anti-Elastase, Anti-Collagenase, Anti-Hyaluronidase Activities of Safranal and Determination of Its Sun Protection Factor in Skin Photoaging.” Bioorganic Chemistry 77: 159–167. doi:10.1016/j.bioorg.2017.12.030.
- Kuppusamy, U. R., H. E. Khoo, and N. P. Das. 1990. “Structure-Activity Studies of Flavonoids as Inhibitors of Hyaluronidase.” Biochemical Pharmacology 40: 397–401. doi:10.1016/0006-2952(90)90709-T.
- Lee, H. Y., E. D. N. S. Abeyrathne, I. Choi, J. W. Suh, and D. U. Ahn. 2014. “Sequential Separation of Immunoglobulin Y and Phosvitin from Chicken Egg Yolk without Using Organic Solvents.” Poultry Science 93: 2668–2677. doi:10.3382/ps.2014-04093.
- Lee, J. H., S. H. Moon, H. S. Kim, E. Park, D. U. Ahn, and H.-D. Paik. 2017. “Immune-Enhancing Activity of Phosvitin by Stimulating the Production of Pro-Inflammatory Mediator.” Poultry Science 96: 3872–3878. doi:10.3382/ps/pex205.
- Lee, J. Y., and A. P. Spicer. 2000. “Hyaluronan: A Multifunctional, MegaDalton, Stealth Molecule.” Current Opinion in Cell Biology 12: 581–586. doi:10.1016/S0955-0674(00)00135-6.
- Lee, S., W. B. Kim, S. H. Park, M. Kim, D. Kim, J. Park, D. Y. Hwang, and H. Lee. 2018. “Biological Properties of Butanol Extracts from Green Pine Cone of Pinus Densiflora.” Food Science and Biotechnology 27: 1485–1492. doi:10.1007/s10068-018-0382-5.
- Liyanaarachchi, G. D., J. K. R. R. Samarasekera, K. R. R. Mahanama, and K. D. P. Hemalal. 2018. “Tyrosinase, Elastase, Hyaluronidase, Inhibitory and Antioxidant Activity of Sri Lankan Medicinal Plants for Novel Cosmeceuticals.” Industrial Crops and Products 111: 597–605. doi:10.1016/j.indcrop.2017.11.019.
- Lu, C. L., and R. C. Baker. 1986. “Characteristics of Egg Yolk Phosvitin as an Antioxidant for Inhibiting Metal-Catalyzed Phospholipid Oxidations.” Poultry Science 65: 2065–2070. doi:10.3382/ps.0652065..
- Miri, A. K., H. S. Heris, L. Mongeau, and F. Javid. 2014. “Nanoscale Viscoelasticity of Extracellular Matrix Proteins in Soft Tissues: A Multiscale Approach.” Journal of the Mechanical Behavior of Biomedical Materials 30: 196–204. doi:10.1016/j.jmbbm.2013.10.022.
- Moon, S. H., K. T. Kim, N. K. Lee, Y. S. Han, S. Y. Nah, S. G. Cho, Y. S. Park, and H.-D. Paik. 2009. “Inhibitory Effects of Naringenin and Its Novel Derivatives on Hyaluronidase.” Food Science and Biotechnology 18: 267–270.
- Moon, S. H., J. H. Lee, M. Lee, E. Park, D. U. Ahn, and H.-D. Paik. 2014. “Cytotoxic and Antigenotoxic Activities of Phosvitin from Egg Yolk.” Poultry Science 93: 2103–2107. doi:10.3382/ps.2013-03784.
- Necas, J., L. Bartosikova, P. Brauner, and J. Kolar. 2008. “Hyaluronic Acid (Hyaluronan): A Review.” Veterinarni Medicina 53: 397–411. doi:10.17221/VETMED.
- Nystrom, A., and L. Bruckner-Tuderman. 2019. “Matrix Molecules and Skin Biology.” Seminars in Cell and Developmental Biology. In press. doi:10.1016/j.semcdb.2018.07.025.
- Sadeghi-Kaji, S., B. Shareghi, A. A. Saboury, and S. Farhadian. 2019. “Spectroscopic and Molecular Docking Studies on the Interaction between Spermidine and Pancreatic Elastase.” International Journal of Biological Macromolecules 131: 473–483. doi:10.1016/j.ijbiomac.2019.03.084.
- Samaraweera, H., W. Zhang, E. J. Lee, and D. U. Ahn. 2011. “Egg Yolk Phosvitin and Functional Phosphopeptides – Review.” Journal of Food Science 76: R143–R150. doi:10.1111/j.1750-3841.2011.02291.x.
- Sunitha, K., P. Suresh, M. S. Santhosh, M. Hemshekhar, R. M. Thushara, G. K. Marathe, C. Thirunavukkarasu, K. Kemparaju, M. Suresh Kumar, and K. S. Girish. 2013. “Inhibition of Hyaluronidase by N-Acetyl Cysteine and Glutathione: Role of Thiol Group in Hyaluronan Protection.” International Journal of Biological Macromolecules 55: 39–46. doi:10.1016/j.ijbiomac.2012.12.047.
- Taborsky, G., and C. C. Mok. 1967. “Phosvitin Homogeneity and Molecular Weight.” Journal of Biological Chemistry 242: 1495–1501.
- Thring, T. S. A., P. Hili, and D. P. Naughton. 2009. “Anti-Collagenase, Anti-Elastase, and Anti-Oxidant Activities of Extracts from 21 Plants.” BMC Complementary and Alternative Medicine 9: 27. doi:10.1186/1472-6882-9-27.
- Wittenauer, J., S. Mackle, D. Submann, U. Schweiggert-Weisz, and R. Carle. 2015. “Inhibitory Effects of Polyphenols from Grape Pomace Extract on Collagenase and Elastase Activity.” Fitoterapia 101: 179–187. doi:10.1016/j.fitote.2015.01.005.
- Zhang, X., F. Geng, X. Huang, and M. Ma. 2016. “Calcium Binding Characteristics and Structural Changes of Phosvitin.” Journal of Inorganic Biochemistry 159: 76–81. doi:10.1016/j.jinorgbio.2016.02.001.