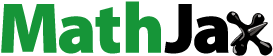
ABSTRACT
1. The impact of feeding sources of n-3 fatty acids (FA) to ISA Brown and Shaver White breeders and their offspring on antibody titres and plasma FA profile was examined.
2. Breeders were fed either a control diet (CON); CON + 1% microalgae (DMA: Aurantiochytrium limacinum) as a source of docosahexaenoic acid; or CON + 2.6% of a co-extruded mixture of full-fat flaxseed (FFF) as a source of α-linolenic acid. Day-old female offspring were assigned to diets (breeder-offspring): 1) CON-CON, 2) CON-DMA, 3) CON – FFF, 4) DMA – CON, 5) DMA – DMA, 6) FFF – CON or 7) FFF – FFF, followed by a standard layer diet through 18 weeks of age (WOA) to 42 WOA.
3. Antibody titres against infectious bronchitis (IBV) and Newcastle disease (NDV) were measured at six days and six WOA, and plasma FA profile was measured at 18 and 42 WOA.
4. Pullets from FFF-fed breeders had higher antibody titres against IBV and NDV than pullets fed DMA (P < 0.05). Feeding FFF to offspring increased plasma ∑n-3 FA at 18 and 42 WOA, whereas feeding DMA to offspring reduced ∑n-6 FA at 18 WOA.
5. In conclusion, independent of breeder strain, alpha linoleic acid (ALA) and DHA sources showed varied responses. Feeding breeders FFF increased plasma concentration of antibody titres and n-3 FA whereas DMA reduced plasma concentration of ∑n-6 FA.
Introduction
The n-3 polyunsaturated fatty acids (PUFA) have attracted considerable attention due to potential health benefits (Lee et al. Citation2018). Dietary inclusion of n-3 PUFA has been shown to improve cell flexibility and functionality of immune cells, including modulating eicosanoid production (Lee et al. Citation2018). Supplementation with n-3 PUFA has been reported to increase antibody production to Newcastle disease (NDV) in pullets (Puthpongsiriporn and Scheideler Citation2005), blood lysozyme activity and delayed hypersensitivity inflammatory response in broiler chickens due to the reduction in pro-inflammatory cytokines (Selvaraj and Cherian Citation2004). However, there is a lack of information on the effect of feeding breeder n-3 FA and its subsequent impact on progeny immunocompetence. Hatchlings survivability depends on their ability to respond effectively and appropriately to different stressors because the immune system of a newly hatched chick is underdeveloped and reliant on passive immunity of maternally produced antibodies (Cherian Citation2015).
Previous reports showed that feeding egg-type pullet breeders n-3 PUFA sources increased embryonic utilisation of docosahexaenoic acid (DHA; Akbari Moghaddam Kakhki et al. Citation2020a). Moreover, feeding n-3 PUFA sources to egg-type pullet breeders and their progeny-affected bone attributes in pullets (Akbari Moghaddam Kakhki et al. Citation2020b, Citation2020c). Specific embryonic environmental conditions have been shown to provoke the adaptive responses in the mammalian foetus (Mennitti et al. Citation2015). These adaptive responses may result in epigenetic changes that can alter gene expression and have a permanent effect on the structure and function of several organs and tissues (Mennitti et al. Citation2015). In the mammalian foetus, a change in the PUFA composition of the offspring cell membranes was observed when the mother was exposed to n-3 PUFA pre-birth (Mennitti et al. Citation2015). This change highlights the possibility of epigenetic modification of the offspring phenotype when they are exposed to n-3 PUFA during embryonic and early-life periods. Therefore, FA profiling might be an indicator of how the exposure to n-3 PUFA during embryonic and early-life periods influence the body FA profile and the biosynthesis of n-3 FA and n-6 FA (Cherian Citation2015; Cherian and Sim Citation2001).
There is limited research on the effects of feeding n-3 PUFA to egg-type pullet breeder and female offspring during rearing and subsequent impact on immunocompetence and FA metabolism in hens. The present study followed progeny from previous studies (Akbari Moghaddam Kakhki et al. Citation2020a, Citation2020b, Citation2020c) to 42 WOA. It was hypothesised that feeding n-3 FA to the breeder, their progeny, or both would improve antibody production against routine vaccines and influence FA metabolism. These effects were hypothesised to be strain-specific between ISA Brown and Shaver White hens. Therefore, the objective of this feeding trial was to study the impact of feeding two sources of n-3 fatty acids to ISA Brown and Shaver White breeders and their offspring on serum concentration of antibody titres against infectious bronchitis (IBV) and Newcastle disease (NDV) vaccines and the concentration of FA in plasma.
Materials and methods
All the birds in this study were cared for based on the Canadian Council on Animal Care guidelines (CCAC Citation2009) under the approved protocol (#3675) by the University of Guelph Animal Care Committee.
Birds, diets and management
ISA Brown and Shaver White breeders (243 ♀ and 36 ♂) were assigned in three replications to one of three dietary treatments at 26 WOA, as previously described in Akbari Moghaddam Kakhki et al. (Citation2020a). Dietary treatments were 1) corn, soybean meal, wheat, and corn gluten-based diet identified as control (CON), 2) CON plus 1% of dried microalgae (Aurantiochytrium limacinum; Alltech, Nicholasville, KY, USA) supplement which contained 17.9% DHA of total fat (DMA), and 3) CON plus 2.6% of a 1:1 (wt/wt) co-extruded full-fat flaxseed and pulse mixture (FFF) which contained 10.5% alpha linoleic acid (ALA; LinPRO®, O & T Farms Ltd, Regina, SK, Canada) (). Deposition of n-3 PUFA in hatching eggs was confirmed after 30 days of offering experimental diets as described in Akbari Moghaddam Kakhki et al. (Citation2020a). Eggs were labelled, incubated at 37.5°C at 55% humidity for 19 days, and hatched at 36.9°C at 66% humidity in a commercial-grade incubator and hatcher (Nature Form, Jacksonville, FL) at the Arkell Poultry Research Station (University of Guelph, Guelph, ON, Canada).
Figure 1. Dietary treatment layout for the breeder and Offspring phases. Day-old female breeder pullets were divided into three dietary treatments: 1) control (CON); 2) micro-Algae (Aurantiochytrium limacinum) fermentation product, as a source of docosahexaenoic acid (DMA); and 3) co-extruded full-fat flaxseed and pulses mixture (50/50, wt/wt), as a source of α-linolenic acid (FFF). Offspring from CON treatment was further divided into three post-hatch treatments (CON, DMA and FFF) while progeny from the DMA and FFF treatments were divided into two post-hatch treatments, CON and DMA or CON and FFF. The concentration of total n-3 FA and ratio of n-6: n-3 were identical among DMA and FFF diets in both phases. After the onset of lay, from 19 to 42 weeks of age (WOA), all birds were fed a common commercial layer diet
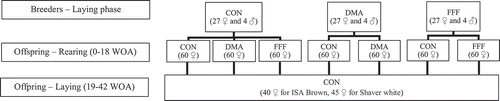
Female hatchlings were weighed and distributed into post-hatch treatments with five replications () and further details described in Akbari Moghaddam Kakhki et al. (Citation2020b). Hatchlings from the CON breeder group were divided into three post-hatch treatments: CON, DMA, and FFF. Hatchlings from the DMA breeder group were divided into two post-hatch treatments: CON and DMA. Hatchlings from the FFF breeder group were divided into two post-hatch treatments: CON and FFF. Breeder and pullet diets containing DMA and FFF had similar total n-3 FA and n-6: n-3 FA and met or exceeded nutrient specifications (Commercial product guide – ISA Brown Citation2018; Commercial product guide – Shaver White Citation2018). The rearing vaccination program included infectious bronchitis (administered by spray), Marek’s disease (injection) and Immucox (gel droplet) at hatch, Newcastle and bronchitis vaccine (spray) and ILT Vectormune FP-LT-AE (wing web) at six WOA, Newcastle-bronchitis at 10 (spray) and 16 (intramuscular) WOA. At the onset of lay, birds were transitioned to conventional commercial cages, retained on breeder-rearing treatment combinations and fed common commercial laying diet without supplemental source of n-3 FA containing 39.16% LA, 2.79% ALA (of total fat), and 4.55% total fat throughout the laying phase as described by Akbari Moghaddam Kakhki et al. (Citation2020c). Further details on the housing system, temperature, and lighting regimens post-hatch to 42 WOA are available in Akbari Moghaddam Kakhki et al. (Citation2020b, Citation2020c).
Sampling and analyses
Ten fresh egg yolks from each breeder treatment were analysed for total IgY levels using a commercial kit (Pierce Chicken IgY Purification Kit, 44 918) based on the method described by (Lu et al. Citation2019). Blood samples were taken from progeny pullets (two birds per cage) on day six and week six of age (five days post-vaccination; (Avakian et al. Citation2000) via wing vein puncture, placed on ice and transported to the laboratory. Blood samples were centrifuged at 2,500 × g for 15 min at 4°C and serum kept at −80°C. Antibody titres of avian infectious bronchitis virus (IBV) and NDV were measured by assay kits (IBV: CK119 IBV and NDV: CK116 NDV, Biochek USA Corporation, Scarborough, ME) following supplier recommendations. Further blood samples (two birds per cage) were taken at 18 and 42 WOA for plasma FA profile. Blood samples were centrifuged at 2,500 × g for 15 min at 4°C and stored at −80°C until required for analyses. Briefly, 50 µl of plasma was mixed with potassium chloride, chloroform, and methanol. Homogenised samples were spun at 836 × g for 10 min. The chloroform layer was then transferred to a new tube, and chloroform was evaporated off under nitrogen gas, and methanol was added for saponification at 100°C for 1 h. The rest of the analyses followed methods described by Akbari Moghaddam Kakhki et al. (Citation2020a). The plasma concentration of linoleic acid (LA), ALA, arachidonic acid (AA), eicosapentaenoic acid (EPA), docosapentaenoic acid (DPA), DHA and total FA were determined. The concentrations of other FA were below detection limit or lower than 0.50%.
Calculations and statistical analyses
The activity of enzymes involved in the biosynthesis of long-chain n-6 PUFA (Δ6-desaturase + elongase + Δ5-desaturase activity) and the biosynthesis of long-chain n-3 PUFA (Δ6-desaturase + elongase + Δ5-desaturase activity) was calculated by calculating the ratio of daughter FA to precursor FA (Nain et al. Citation2012).
Plasma concentrations of LA, ALA, AA, EPA, DPA, DHA were expressed as a proportion of total plasma fatty acids. Data of antibody titres for IBV and NDV were transformed and presented as log10. Data were tested for normality using Univariate Plot normal procedure of SAS 9.4. Data for hatching eggs IgY concentration were subjected to a two-way ANOVA with fixed effects of strain (ISA Brown and Shaver White) × diets (CON, DMA, and FFF) and associated interactions using GLIMMIX procedures of SAS. The rest of the data were subjected to a nested design analysis with fixed effects of strain, breeder diet, and offspring diet, strain × breeder diet interaction and strain and offspring x diet interactions using GLIMMIX procedure of SAS 9.4. Significance was declared at P < 0.05.
Results
There was no interaction between strain and breeder diet on total IgY content (P = 0.658; ). Yolks from hens fed DMA contained 12.17% higher concentration of total IgY than CON (2.12 vs. 1.89 mg/ml, P = 0.001), while the FFF group maintained an intermediate concentration similar to DMA and CON (P > 0.05, ). The total IgY content was 5.6% higher in ISA Brown hens than Shaver White hens (2.07 vs. 1.96 mg/ml, P = 0.001)
Figure 2. Effects of feeding sources of docosahexaenoic and α-linolenic acids (± standard error) to ISA Brown and Shaver White breeders on IgY content (mg/mL) in egg yolks. Ten eggs per treatment. CON: Control; DMA: Microalgae (Aurantiochytrium limacinum) fermentation product, as a source of docosahexaenoic acid; FFF: Co-extruded full-fat flaxseed and pulse mixture (1:1 wt/wt), as a source of α-linolenic acid. Bars with different letters are significantly different from each other (P < 0.05)
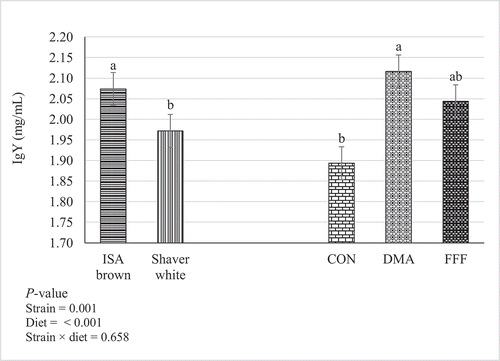
There were no strain x diet interactions nor offspring diets effects on IBV antibody titre in six day old pullets (P > 0.05; ). Breeder diet effects on antibody titres in six day old pullets were such that FFF had higher IBV titres than DMA (4.02 vs. 3.89 log 10, P = 0.03). The six day old ISA Brown pullets had a higher IBV antibody titre than Shaver White pullets (4.00 vs. 3.91 log10, P = 0.015). There was no interaction between strain and breeder diets on IBV and NDV antibody titre in six WOA pullets (P > 0.05). ISA Brown pullets had a 17.5% greater NDV antibody titre compared with Shaver White pullets (2.61 vs. 2.22 log10, P < 0.001). There was breeder diet effect at six WOA, whereby pullets from breeders fed FFF had higher IBV and NDV antibody titre than DMA pullets (P < 0.05). However, pullets from FFF or DMA had similar (P > 0.05) IBV and NDV titres to the CON pullets. The offspring diet effect was such that CON-DMA had a higher antibody titre for IBV and NDV than DMA-DMA (2.55 vs. 2.17 log10, P < 0.05).
Table 1. Effects of feeding sources of docosahexaenoic and α-linolenic acids to ISA Brown and Shaver White breeders and offspring on plasma concentration of antibody titres against avian infectious bronchitis virus (IBV), and Newcastle disease virus (NDV) in offspring1.
There was no interaction between strain and diets on plasma percent composition of LA, ALA, AA, EPA, DHA, ∑n-3 PUFA, ∑n-6 PUFA, ∑n-6: ∑n-3 PUFA ratio, and total FA at 18 WOA (P > 0.05, ). ISA Brown pullets had a higher percent composition of LA, ALA, AA, EPA, DHA, ∑n-3 PUFA and ∑n-6 PUFA compared with Shaver White pullets (P < 0.05). The main effect of the breeder diet was such that pullets from CON and FFF had a higher percent composition of LA, ∑n-3 PUFA, ∑n-6 PUFA, and lower total fat compared with DMA pullets (P < 0.05). The breeder diet did not affect the percent composition of ALA, AA, EPA, DHA, and ∑n-6: ∑n-3 PUFA ratio (P > 0.05). Pullets in CON-CON and FFF-CON groups maintained a higher percent composition of LA compared with the rest of the treatments (P = 0.005). Pullets in CON-FFF and FFF-FFF groups maintained a higher percent composition of ALA compared with the rest of the treatments (P < 0.001). The greatest plasma percent composition of AA was observed in pullets of CON-CON, FFF-CON and FFF-FFF groups, whereas pullets of CON-DMA and DMA-DMA had the lowest percent composition of AA (P < 0.001). The percent composition of EPA was higher in pullets of FFF-FFF relative to pullets of FFF-CON, DMA-CON, DMA-DMA, and CON-CON groups (P < 0.001). The percent composition of DHA was higher in pullets of CON-DMA, DMA-DMA and FFF-FFF relative to pullets of CON-CON, DMA-CON, and FFF-CON groups (P < 0.001). The proportion of EPA was higher in pullets of FFF-FFF and CON-DMA relative to pullets of FFF-CON, DMA-CON, DMA-DMA, and CON-CON groups (P < 0.001). Pullets of CON-DMA, CON-FFF and DMA-DMA and FFF-FFF groups had a higher ∑n-3 PUFA compared to other treatments (P < 0.001). The greatest plasma ∑n-6 PUFA was observed in pullets of CON-CON, FFF-CON, whereas pullets of DMA-DMA had the lowest plasma ∑n-6 PUFA (P = 0.007). The ∑n-6: ∑n-3 PUFA ratio was lower in the CON-DMA, CON-FFF, DMA-DMA, FFF-FFF groups compared to the rest of the treatments (P < 0.001). The greatest total plasma fat was observed in pullets of DMA-CON and DMA-DMA, followed by CON-CON, FFF-CON, DMA-CON compared to the rest of the treatments (P =0.001).
Table 2. Effects of feeding sources of docosahexaenoic and α-linolenic acids to ISA Brown and Shaver White breeders and offspring on plasma concentration of fatty acid in 18-week-old pullets1.
The percent composition of LA, ALA, AA, DHA, as well as ∑n-3 PUFA, ∑n-6 PUFA, ∑n-6: ∑n-3 PUFA ratio, and total fat at 42 WOA were not affected by the interaction between strain and breeder diets (P > 0.05; ). ISA Brown hens had lower total fat compared with Shaver White hens (P <0.001). The breeder and offspring diet did not influence the percent composition of FA and total fat in plasma at 42 WOA. ).
Table 3. Effects of feeding sources of docosahexaenoic and α-linolenic acids to ISA Brown and Shaver White breeders and offspring during rearing on plasma concentration of fatty acids in 42-week-old hens1.
Calculated n-3 and n-6 PUFA desaturation and elongation
There was no interaction between strains and breeder diets on the activity of n-6 and n-3 PUFA desaturation and elongation at 18 and 42 WOA (P > 0.05;). ISA Brown had greater n-3 PUFA biosynthesis compared to Shaver White pullets (0.52 vs. 0.19, P = 0.001). However, this difference did not last until 42 WOA (P = 0.115). Shaver White hens showed higher n-6 PUFA biosynthesis compared to ISA Brown hens (0.17 vs. 0.15, P < 0.001). Feeding breeders FFF increased n-6 PUFA biosynthesis in their offspring at 18 WOA (P < 0.001), but did not affect the n-3 PUFA biosynthesis at 18 WOA or n-6 and n-3 PUFA biosynthesis at 42 WOA (P > 0.05). The n-6 PUFA biosynthesis at 18 WOA varied among different offspring diets. The CON-FFF had a higher biosynthesis than CON-DMA, DMA-CON was higher than DMA-DMA, and FFF-FFF had a higher biosynthesis than FFF-CON (P < 0.001). At 42 WOA, hens fed the CON-DMA and FFF-FFF had a higher n-6 PUFA biosynthesis compared with the rest of the treatments (P < 0.001). The n-3 PUFA biosynthesis was higher in pullets fed CON-DMA compared to CON-CON and DMA-CON groups (P < 0.001). However, these effects in n-3 PUFA biosynthesis were not observed at 42 WOA (P > 0.05).
Table 4. Effects of feeding sources of docosahexaenoic and α-linolenic acids to ISA Brown and Shaver White breeders and offspring during rearing on calculated biosynthesis of fatty acids at 18 and 42 week of age (WOA)1.
Discussion
Maternal nutrition during pregnancy or lactation has been reported to affect the foetus, new-born and adult mammals through modifying foetal programming and epigenetic regulation (Mennitti et al. Citation2015). In avian species, this condition is different, as the nutrients deposited into the egg by the hen are the only source of nutrients available to the embryo to sustain its growth (Cherian Citation2015). Fatty acids, especially long-chain PUFA, are needed for embryonic and postnatal development due to their vital roles in the synthesis of structural lipids and other metabolic functions. It has been reported that the utilisation of PUFA during the embryonic period of pullets is preferentially shifted to DHA (Akbari Moghaddam Kakhki et al. Citation2020a).
In the current study, supplementation of breeder diets with DMA increased the total IgY content in the yolk, which was in agreement with other findings (Cherian and Quezada Citation2016), where it was observed that supplementation with 10% full-fat camelina or flaxseed in the diet of 48-week old Lohman Brown birds increased total IgY concentration in the yolk. The results from the current study suggested that the level of supplemental FFF may not be adequate to increase IgY concentration. Embryonic supply of n-3 FA through maternal-feeding has been reported to modify cell membrane FA profiles and production of inflammatory mediators (Cherian Citation2015). The ratio of ∑n-6:∑n-3 FA is a critical factor in inducing modulation in PUFA composition in immune cells in laying hens (Hall et al. Citation2007). Subsequently, it can increase transferable Ig formation and deposition in the egg (Wang et al. Citation2000). The ratio of ALA: LA has been reported to affect maternal antibodies in broiler breeders (Wang et al. Citation2004). At the same time, this ratio has not been shown to influence humoral antibodies (Wang et al. Citation2004). The dietary FA profile has been shown to affect the binding activity of the IgY-receptor to the yolk sac membrane or the half-life of antibodies in the embryo (Koutsos et al. Citation2003). Awadin et al. (Citation2020) reported that dietary n-3 PUFA supplementation via fish oil for four weeks improved growth performance and alleviated the deleterious immunological and pathological effects of NDV and avian influenza virus H9N2 infection in quails.
The effect of the breeder and direct-feeding of n-3 FA to offspring on antibody titters was dependent on the age of birds. At six days of age, only the breeder diets affected antibody titre. Later at six WOA, both breeder and offspring diets affected antibody titre. The effect on titre was dependent on the type of n-3 FA, whereby only breeders fed FFF increased antibodies in the offspring, whereas DMA fed to the offspring enhanced titre. However, when both breeder and offspring received DMA it had adverse impact on titre. Sijben et al. (Citation2000) reported that total serum antibody titre after immunisation with Mycobacterium butyricum in 35 WOA ISA-Warren crosses was higher when birds were fed diets containing 3.9% ALA and 3.0% LA, compared with those fed 0.5% ALA and 6.2% LA. Conversely, Khatibjoo et al. (Citation2011) observed no change in antibody response or in-vitro lymphocyte proliferation in broiler breeders response to increasing n-3 FA level from 0.09 to 0.35% (kilka oil) with a constant concentration of n-6 FA.
Certain factors might contribute to discrepancies in the antibody responses reported in various studies, such as 1) the interaction of n-3 and n-6 PUFA, which is more important than the separate effects of n-3 or n-6 PUFA; 2) the phase of the response; and 3) the nature of the challenging antigen (Sijben et al. Citation2001) and the supplemental level of PUFA. Other components in the n-3 FA source may contribute to such variation between studies. For example, the microalgae used in the current study as source of DHA contained several other bioactive components, such as vitamins, chlorophyll, carotenoids and phycobiliproteins with immunomodulating properties (Koyande et al. Citation2019).
The strain difference in antibody titre was minimal in the present study. The ISA Brown birds had a higher NDV antibody titre than Shaver White pullets. Puthpongsiriporn and Scheideler (Citation2005) observed that Hy-line W-36 pullets had more NDV antibody production compared to Hy-Line Brown pullets. Lower concentrations of AA in the lymphoid organs of Hy-line W-36 pullets has been attributed to higher antibody production compared to Hy-line Brown pullets, since this may stimulate prostaglandin E2 and suppress antibody production (Puthpongsiriporn and Scheideler Citation2005).
In the present study, ISA Brown pullets had a lower ratio of ∑n-6: ∑n-3 FA in plasma compared with Shaver White pullets. The ISA Brown pullets consumed 187 g more feed throughout the rearing period, compared with Shaver White pullets, which partially contributed to the differences in the FA profile among strains. The differences in plasma FA profile in various strains of layer hens are unknown. Akbari Moghaddam Kakhki et al. (Citation2020a) showed that Shaver White layer breeders were more efficient in depositing n-3 FA in their eggs and had higher n-3 FA biosynthesis than ISA Brown. In addition, 18 WOA pullets did not have any detectable plasma DHA, but maintained EPA at 0.26% as a proportion of total FA. This value dropped to 0.03% at 42 WOA while DHA was concomitantly measured at 0.19%. The reason why 18 WOA pullets did not have detectable levels of DHA is unknown. In the embryonic period, the embryos from the layers fed the test diets consumed more n-3 FA than the CON birds. The first destination for lipoproteins in a young bird is the liver, and it has been reported that n-3 FA can last approximately 30 days after the removal of dietary sources (Cherian Citation2015). Consequently, it is possible that, had the birds been tested at an earlier age such as at six or 30 d, there may have been a maternal effect on the progeny FA profile.
Foetal programming involves adaptive responses to specific prenatal environmental conditions, which may result in alteration of gene expression and permanent effects on the structure and function of several organs and tissues (Mennitti et al. Citation2015). One mechanism by which n-3 PUFA can exert their long-lasting effect is through epigenetic modification in the pattern of DNA methylation. Prenatal exposure to PUFA is involved in epigenetic regulation of its biosynthesis in offspring, through the transcriptional regulation of elongase, ∆-6, and ∆-5 desaturase enzymes, resulting in persistent changes in the PUFA content of cell membranes in the progeny (Mennitti et al. Citation2015). The effect of feeding breeders n-3 FA on plasma FA in 18 WOA pullets was dependent on the sources of n-3 FA, with FFF being more effective in increasing n-3 FA, n-6 FA and n-3 PUFA biosynthesis, while DMA reduced n-6 FA and increased plasma fat content. The effect of feeding breeders n-3 FA on plasma FA profile did not last after 42 WOA. Feeding DMA and FFF to pullets from breeders fed either CON, DMA, or FFF increased ∑n-3 FA, resulting in a lower ratio of ∑n-6 FA:∑n-3 FA FA at 18 WOA. However, this effect did not last after 42 WOA. It has been reported that feeding breeders n-3 PUFA increased DHA content in broiler chickens, which lasted for 28 d, and kept the hepatic content arachidonic acid low for 40 d (Koppenol et al. Citation2014).
Independent of diet types, ISA Brown birds had a higher plasma concentration of ∑n-3 and a lower ratio of ∑n6: ∑n3 FA compared with Shaver White birds. Feeding breeders sources of n-3 FA can increase IgY in hatching eggs. The effectiveness of dietary supplementation of n-3 FA on antibody titre against routine vaccines was dependent on the type of dietary n-3 FA and feeding phase. Feeding breeder ALA was more effective in promoting antibody titters at a younger age (six days old), however, feeding ALA to breeders and offspring altered antibody titres in older pullets (six WOA). Irrespective of breeder dietary background, feeding offspring FFF during rearing could increase plasma n-3 FA through to 42 WOA birds.
Acknowledgments
The authors appreciate the assistance of members of the Monogastric Laboratory at the University of Guelph, (ON) for assistance in animal care and Lyn M. Hillyer for fatty acid analyses. R. Akbari Moghaddam Kakhki is a recipient of the Ontario Trillium Doctoral, Mary Edmunds Williams, King Cole Ducks Ltd, and Craig Hunter Poultry Science Graduate Scholarships. Hendrix Genetics donated the parent flocks.
Disclosure statement
No potential conflict of interest was reported by the authors.
Additional information
Funding
References
- Akbari Moghaddam Kakhki, R., D. W. Ma, K. R. Price, J. R. Moats, N. A. Karrow, and E. G. Kiarie. 2020a. “Enriching ISA Brown And Shaver White Breeder Diets With Sources Of N-3 Polyunsaturated Fatty Acids Increased Embryonic Utilization Of Docosahexaenoic Acid.” Poultry Science 99 (2): 1038–1051. doi:10.1016/j.psj.2019.09.002.
- Akbari Moghaddam Kakhki, R., K. R. Price, J. R. Moats, G. Bédécarrats, N. Karrow, and E. G. Kiarie. 2020b. “Impact Of Feeding Microalgae (Aurantiochytrium Limacinum) And Co-extruded Mixture Of Full-fat Flaxseed As Sources Of N-3 Fatty Acids To ISA Brown And Shaver White Breeders And Progeny On Pullet Skeletal Attributes At Hatch Through To 18 Weeks Of Age.” Poultry Science 99 (4): 2087–2099. doi:10.1016/j.psj.2019.12.016.
- Akbari Moghaddam Kakhki, R., V. Shouldice, K. R. Price, J. R. Moats, and E. G. Kiarie. 2020c. “N-3 Fatty Acids Fed To ISA Brown And Shaver White Breeders And Their Female Progeny During Rearing: Impact On Egg Production, Eggshell And Select Bone Attributes From 18 To 42 Weeks Of Age.” Poultry Science. In-Press. doi:10.1016/j.psj.2020.03.061.
- Avakian, A. P., P. S. Wakenell, D. Grosse, C. E. Whitfill, and D. Link. 2000. “Protective Immunity to Infectious Bronchitis in Broilers Vaccinated against Marek’s Disease either in Ovo or at Hatch and against Infectious Bronchitis at Hatch.” Avian Diseases 44 (3): 536–544. doi:10.2307/1593092.
- Awadin, W. F., A. H. Eladl, R. A. El-Shafei, A. Mohamed, A. E. Aziza, H. S. Ali, and M. A. Saif. 2020. “Effect of Omega-3 Rich Diet on the Response of Japanese Quails (Coturnix Coturnix Japonica) Infected with Newcastle Disease Virus or Avian Influenza Virus H9N2.” Comparative Biochemistry And Physiology C-Toxicology & Pharmacology 228: 108668. doi:10.1016/j.cbpc.2019.108668.
- CCAC. 2009. The Care and Use of Farm Animals in Research, Teaching and Testing. ed C. C. o. A. Care. in O. Ottawa, Canada., ed..
- Cherian, G. 2015. “Nutrition and Metabolism in Poultry: Role of Lipids in Early Diet.” Journal of Animal Science and Biotechnology 6 (1): 28. doi:10.1186/s40104-015-0029-9.
- Cherian, G., and N. Quezada. 2016. “Egg Quality, Fatty Acid Composition and Immunoglobulin Y Content in Eggs from Laying Hens Fed Full Fat Camelina or Flax Seed.” Journal of Animal Science and Biotechnology 7: 15. doi:10.1186/s40104-016-0075-y.
- Cherian, G., and J. Sim. 2001. “Maternal Dietary α-linolenic Acid (18: 3n-3) Alters N-3 Polyunsaturated Fatty Acid Metabolism and Liver Enzyme Activity in Hatched Chicks.” Poultry Science 80 (7): 901–905. doi:10.1093/ps/80.7.901.
- Commercial product guide - ISA Brown. “North American Version.” Boxmeer, The Netherlands: Hendrix Genetic. Accessed September 2018.
- Commercial product guide - Shaver White. “North American Version.” Boxmeer, The Netherlands: Hendrix Genetic. Accessed September 2018.
- Hall, J., S. Jha, and G. Cherian. 2007. “Dietary N-3 Fatty Acids Decrease the Leukotriene B4 Response Ex Vivo and the Bovine Serum Albumin-induced Footpad Swelling Index in New Hampshire Hens.” Canadian Journal of Animal Science 87 (3): 373–380. doi:10.4141/CJAS06026.
- Khatibjoo, A., H. Kermanshahi, A. Golian, and M. Zaghari. 2011. “The Effect of Dietary N-6: N-3 Ratio and Sex on Broiler Breeder Immunity.” Poultry Science 90 (10): 2209–2216. doi:10.3382/ps.2011-01373.
- Koppenol, A., J. Buyse, N. Everaert, E. Willems, Y. Wang, L. Franssens, and E. Delezie. 2014. “Transition of Maternal Dietary N-3 Fatty Acids from the Yolk to the Liver of Broiler Breeder Progeny via the Residual Yolk Sac.” Poultry Science 94 (1): 43–52. doi:10.3382/ps/peu006.
- Koutsos, E. A., A. J. Clifford, C. C. Calvert, and K. C. Klasing. 2003. “Maternal Carotenoid Status Modifies the Incorporation of Dietary Carotenoids into Immune Tissues of Growing Chickens (Gallus Gallus Domesticus).” Journal of Nutrition 133 (44): 1132–1138. doi:10.1093/jn/133.4.1132.
- Koyande, A. K., K. W. Chew, K. Rambabu, Y. Tao, D. T. Chu, and P. L. Show. 2019. “Microalgae: A Potential Alternative to Health Supplementation for Humans.” Food Science and Human Wellness 8 (1): 16–24. doi:10.1016/j.fshw.2019.03.001.
- Lee, S. A., N. Whenham, and M. R. Bedford. 2018. “Review on Doco-sahexaenoic Acid in Poultry and Swine Nutrition: Consequence of Enriched Animal Products on Performance and Health Characteristics.” Animal Nutrition 5 (1): 11–21. doi:10.1016/j.aninu.2018.09.001.
- Lu, Z., A. Thanabalan, H. Leung, R. Akbari Moghaddam Kakhki, R. Patterson, and E. G. Kiarie. 2019. “The Effects of Feeding Yeast Bioactives to Broiler Breeders And/or Their Offspring on Growth Performance, Gut Development, and Immune Function in Broiler Chickens Challenged with Eimeria.” Poultry Science 98 (12): 6411–6421. doi:10.3382/ps/pez479.
- Mennitti, L. V., J. L. Oliveira, C. A. Morais, D. Estadella, L. M. Oyama, C. M. O. Do Nascimento, and L. P. Pisani. 2015. “Type of Fatty Acids in Maternal Diets during Pregnancy And/or Lactation and Metabolic Consequences of the Offspring.” Journal of Nutritional Biochemistry 26 (2): 99–111. doi:10.1016/j.jnutbio.2014.10.001.
- Nain, S., R. Renema, D. Korver, and M. Zuidhof. 2012. “Characterization of the N-3 Polyunsaturated Fatty Acid Enrichment in Laying Hens Fed an Extruded Flax Enrichment Source.” Poultry Science 91 (7): 1720–1732. doi:10.3382/ps.2011-02048.
- Puthpongsiriporn, U., and S. Scheideler. 2005. “Effects of Dietary Ratio of Linoleic to Linolenic Acid on Performance, Antibody Production, and in Vitro Lymphocyte Proliferation in Two Strains of Leghorn Pullet Chicks.” Poultry Science 84 (6): 846–857. doi:10.1093/ps/84.6.846.
- Selvaraj, R. K., and G. Cherian. 2004. “Dietary N‐3 Fatty Acids Reduce the Delayed Hypersensitivity Reaction and Antibody Production More than N‐6 Fatty Acids in Broiler Birds.” European Journal of Lipid Science and Technology 106 (1): 3–10. doi:10.1002/ejlt.200300848.
- Sijben, J., H. De Groot, M. Nieuwland, J. Schrama, and H. Parmentier. 2000. “Dietary Linoleic Acid Divergently Affects Immune Responsiveness of Growing Layer Hens.” Poultry Science 79 (8): 1106–1115. doi:10.1093/ps/79.8.1106.
- Sijben, J., M. Nieuwland, B. Kemp, H. Parmentier, and J. Schrama. 2001. “Interactions and Antigen Dependence of Dietary N-3 and N-6 Polyunsaturated Fatty Acids on Antibody Responsiveness in Growing Layer Hens.” Poultry Science 80 (7): 885–893. doi:10.1093/ps/80.7.885.
- Wang, Y., G. Cherian, H. Sunwoo, and J. Sim. 2000. “Dietary Polyunsaturated Fatty Acids Significantly Affect Laying Hen Lymphocyte Proliferation and Immunoglobulin G Concentration in Serum and Egg Yolk.” Canadian Journal Animal Science 80 (4): 597–604. doi:10.4141/A00-013.
- Wang, Y., H. Sunwoo, G. Cherian, and J. Sim. 2004. “Maternal Dietary Ratio of Linoleic Acid to Alpha-linolenic Acid Affects the Passive Immunity of Hatching Chicks.” Poultry Science 83 (12): 2039–2043. doi:10.1093/ps/83.12.2039.