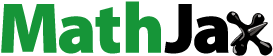
ABSTRACT
1. Two series of studies were conducted to determine the effects of a combination of ground plant material derived from Quillaja saponaria trees and Yucca schidigera plants (QY) as sources of saponin, on performance, productivity, nutrient digestibility and ileal morphology of growing broilers. In each trial, 480 Cobb male birds were allocated equally to 24 pens at one-day-of-age according to body weight
2. The studies consisted of two identical floor pen trials in which performance and nutrient digestibility were assessed and two trials where performance and ileal morphology were determined. In each trial, 0, 250 or 500 ppm QY were included in feed given to the broilers from 1–35 or 1–42 d of age, respectively. Eight (digestibility) or 12 (morphology) randomised replicate pens were used.
3. In the digestibility trials, two birds per pen were moved to metabolism cages at d 21. Excreta was collected for a five-day period (d 21 to 25) for the determination of apparent total tract digestibility of dry and organic matter, fat and ash and nitrogen retention. For intestinal morphology, ileal segments were collected from four birds/pen on d 21 to determine villus height and crypt depth. Performance data were collected in each trial series.
4. Results showed that feeding graded levels of QY produced significant linear improvements in performance and productivity at d 35, and similar linear effects were observed for N retention and all apparent digestibility measurements. Morphology data showed that birds receiving 250 and 500 ppm QY had significantly increased villus height
5. These results indicated that QY exerted a positive influence on the intestinal tract by increasing the absorptive surface and improving nutrient digestibility. These effects were considered to be associated with the performance improvements recorded in both experiments.
Introduction
Saponins are a broad group of plant-derived compounds composed of both water-soluble and fat-soluble moieties. These properties impart detergent-like qualities to these molecules that allow them to display many biological activities (Cheeke Citation2000). Studies of their function in plants have indicated that insect attack and invasion by pathogens are reduced in their presence (Sparg et al. Citation2004). Due to these activities, saponins have received much attention in the last 50 years, because they hold promise for development as antibacterial, antiviral and antiparasitic agents for human and animal applications (Francis et al. Citation2002).
According to Cheeke (Citation2000), certain desert plants are especially rich in saponin content. Quillaja saponaria, the Chilean soap bark tree and Yucca schidigera, a desert plant from the American southwest, have become the major sources of commercially used saponins. Due to their chemical properties, they are frequently used in soaps and detergents, shampoo, cosmetics and soft drinks. In addition, their pharmacologic properties have been extensively examined and they are known to affect ammonia production, reduce serum cholesterol and influence the integrity of biological membranes (Francis et al. Citation2002; Augustin et al. Citation2011; Fleck et al. Citation2018). Studies have shown that yucca saponins can reduce cytokine levels associated with inflammatory responses (Oelschlager et al. Citation2019) and quillaja saponins are known for their ability to stimulate mucosal immunity (Kensil Citation1996). Specific saponins isolated from Quillaja saponaria are now widely used as adjuvants in human and veterinary vaccines (Fleck et al. Citation2018).
In poultry applications, quillaja and yucca saponins have been shown to affect intestinal bacteria, with activity against Salmonella enterica ser. Typhimurium, Escherichia coli, and Staphylococcus aureus and other Gram-positive organisms has been described (Hassan et al. Citation2010). Additionally, recent work has shown that a combination of quillaja and yucca saponins (QY) exerted anticoccidial effects in growing broilers (Bafundo et al. Citation2020). Results demonstrated that oocyst production and lesion scores were reduced by QY alone, and when combined with other anticoccidial agents, disease prevention and production performance were enhanced. Supplemental work indicated that coccidiosis vaccinated broilers responded favourably in the presence of QY (Bafundo et al. Citation2021), suggesting that the performance deficits normally observed with vaccine usage (Arczewska-Wlosek and Swiatkiewicz Citation2014) were offset by QY.
The following studies were intended to provide additional insight into the intestinal effects that occur when QY is administered to growing broilers. Specifically, these trials were designed to determine the effects of graded levels of QY administration on performance and total tract nutrient digestibility and to determine whether QY feeding influenced morphologic characteristics of ileal villi.
Materials and methods
The trials conducted in this analysis were carried out in two locations: Germany and the USA. The floor pen digestibility trials were conducted at the Freie University of Berlin, Berlin, Germany. These trials were performed in accordance with the Animal Welfare Act of Germany and approved by the local state office of occupational health and technical safety (Landesamt für Gesundheit und Soziales, LaGeSo, no. A 0439/17). Animals used in the study were raised and treated according to European Union Directive 2010/63/EU, covering the protection of animals used for experimental or other purposes and according to the recommendation of Commission 2007/526/CE covering the accommodation and care of animals used for experimental purposes. During the study, appropriate animal health and welfare inspections were carried out.
The pen studies used to assess changes in ileal morphology were conducted at AHPharma, Inc., Hebron, Maryland USA. The Animal Care and Use Committee of AHPharma, Inc. approved all procedures used in the trials. In both locations, birds were humanely euthanised by cervical dislocation using procedures approved by the respective agencies above.
The combination of quillaja and yucca (QY) was a commercially prepared product (Magni-Phi®, Phibro Animal Health Corp., Teaneck, New Jersey, USA). The product consists of 100% ground plant material derived from Quillaja saponaria trees and Yucca schidigera plants formulated in a proprietary ratio, where quillaja was the major component. No excipients or carriers were used in the product. Thus, unaltered, unextracted quillaja saponins supplemented with those from yucca comprised the active ingredients, and made up approximately 4% of the total product.
Digestibility studies
Two identical studies were conducted, and each trial was arranged as a completely randomised design involving eight pen replicates of the three treatments: QY 0, 250 and 500 ppm, in feed. The dietary treatments in each test were fed from 1 to 35 d of age. In total, 480 healthy birds per trial were allocated equally to 24 pens (0.28 m2 per bird) according to body weight, giving 20 birds per pen, totalling 160 birds per treatment. At the outset of the trials, each pen contained new bedding of softwood shavings filled to a depth of 5 cm. Since the objectives involved evaluation of performance and nutrient digestibility in healthy broilers, no disease organisms of any type were added to the litter and efforts were made to minimise cross-contamination of litter between pens. Neither clinical nor subclinical diseases were observed in the conduct of the trials.
The basal starter and grower feeds manufactured for each study were subsequently divided into three aliquots for mixing the experimental diets containing QY (). Proximate analysis of dry matter, crude protein, crude fat, crude ash and crude fibre was conducted prior to the trials to confirm correct diet mixing, key nutritional content and homogeneity. Before the trial, the QY content of each diet was verified by a saponin assay developed specifically for these components. To avoid carry-over of the tested compounds from treatments, diets were manufactured in an appropriate order, starting with the control diets followed by rations of increasing QY concentration. Neither antibiotic nor anticoccidial medication was used in these trials.
Table 1. Diets formulated for evaluating graded levels of QY (0, 250 and 500 ppm) on whole tract nutrient digestibility
The birds were fed a starter diet from 1 to 14 d and a grower diet from 15 to 35 d (). Diets were maize-soya based and were designed to meet the nutritional requirements of growing broilers (Society of Nutritional Physiology Citation1999). Throughout the feeding period, temperature, relative humidity, lighting and ventilation were controlled and, if necessary, adjusted to the target values. Room temperature was gradually reduced from approximately 33°C on d 1 to about 23°C from d 28 of age onward. Artificial light (45 lx) was provided continuously during the first four days, but from d 5 onward, lighting of 35 lx was provided for 18 h per day; this was followed by a 6 h dark cycle (4 lx).
Body weights and feed consumption were recorded weekly. Average daily gain (ADG) was calculated by dividing BWG by the number of days in each period. From these data, feed conversion ratio (FCR) and European Poultry Efficiency Factor (EPEF) were determined. EPEF was calculated by multiplying ADG x percent survival rate and divided by feed conversion rate x 10. Performance criteria were calculated for the starter period (to d 14) and d 35 at trial termination. ADG and EPEF were presented for the entire growth period (42 d).
Digestibility assessments
Apparent total tract digestibility was determined from two broilers per pen, selected with body weights closest to the average of their treatment group, in total 16 birds per treatment per experiment. From d 21 to d 25 in each trial, birds were housed in pairs in metabolism cages and fed the treatment rations to which they were originally assigned; excreta were collected during a 5-d sampling period (d 21 to 25 of age). The excreta collected during this period were pooled by cage, resulting in eight replicates per treatment. The pooled excreta were stored at −20°C before being freeze-dried and subjected to chemical analyses. Dry and organic matter digestibility were calculated, and, for determination of the apparent total tract digestibility, titanium (IV) dioxide (TiO2) was used as an inert marker in feed at 4 g/kg diet (Short et al. Citation1996). Analyses were conducted in accordance with methods issued by VDLUFA (Citation2013).
Apparent total tract digestibility was calculated as follows:
Digestibility measurements were calculated for each pen and data were expressed as percent apparent total tract digestibility for most variables. Total tract nitrogen retention was determined using the uric acid content of excreta.
Morphometric assessments
Two additional floor pen trials were carried out in Hebron, Maryland, USA, to determine the effects of graded levels of QY on bird performance, height of intestinal villi and depth of the crypts of Lieberkühn measured in ileal segments at 21 d of age. The ileal villi results were then correlated to final body weight measured at d 42 of the trial. Each trial was arranged as a randomised complete block design involving 12 blocks of three treatments (QY 0, 250 and 500 ppm in feed) per test. Each pen contained 55 Cobb 500 broiler chickens that were reared until 42 d of age. Results of the two morphology studies were pooled, and tabularised data represented means of 24 replications of each treatment.
Diets used in these tests were standard maize-soya based commercial rations formulated to meet or exceed the nutrient requirements of growing broilers (National Research Council Citation1994). A feeding plan involving starter, grower and withdrawal rations was employed where starter was fed up to day 14, grower until day 28 and withdrawal rations from days 29 to 42. Additional management procedures were consistent with methods used in US broiler production.
The broilers were vaccinated for coccidiosis at the hatchery (Coccivac B52, Merck Animal Health, Madison, NJ, USA) and then reared in an environment containing intestinal pathogens, whereby commercial broiler litter was used that originated from Maryland farms known to have recently experienced coccidiosis and necrotic enteritis (NE). Equal amounts of this bedding were distributed among all the pens in the study. In addition, each pen was sprayed with 1 × 105 Eimeria acervulina and 3.5 × 104 E. maxima sporulated oocysts per bird prior to the start of each test. As shown in Bafundo et al. (Citation2021), these procedures are known to induce a significant intestinal disease challenge characterised by moderate to severe coccidiosis, moderate NE and overall mortality two to three times greater than American industry standards. Evaluation of both coccidial and NE lesions at critical time points and total mortality at the end of the trial confirmed the highly pathogenic nature of these conditions.
Performance in these trials was measured at d 21 and 42, and ADG was calculated for the entire growth period. At d 21 of the tests, four birds per pen were humanely killed by cervical dislocation and ileal segments of the intestine were collected and immediately placed in neutral buffered formalin. Standard histological procedures were used in the preparation of these tissues; average ileal villus height and crypt depth were determined from four birds per pen.
Statistics
As the experimental designs, animals, pens, facilities, rations and study methods in each group of tests were the same, data generated in each group were pooled. The linear effects of graded QY levels on each metric were further tested using linear regression analysis. For the morphometric trials, the linear models were:
where represented the growth metric tested and QY level the level of QY dosage. The linear models fitted to the digestibility trials data were identical, except for the absence of a block effect and were written as:
The QY-induced improvements in villus height were regressed against final body weight in a third linear regression using the following equation:
Statistical differences between individual graded level treatments were tested by applying the Holm-Bonferroni procedure to the Least Significant Difference methodology (Holm Citation1979) using the R package agricolae (De Mendiburu Citation2020). While similar to the Bonferroni test, the Holm-Bonferroni procedure offers improved statistical power, while still controlling family-wise error rates; the test is valid under the same conditions as the Bonferroni method (Holm Citation1979). In all cases, statistical differences were established at P < 0.05 and trends were noted when P < 0.1. All statistical analyses were conducted in the R statistical software (R Core Team Citation2017).
Results
Pooled performance results of the digestibility trials are shown in . At d 14, body weights were not significantly improved by addition of QY, although trends for a linear improvement in body weights in line with dosage were evident (P = 0.065). However, 14 d FCR values were significantly improved with each added level of QY in a linear manner. By d 35, the 250 and 500 ppm QY diets improved body weights as a significant linear effect. At d 35, QY at 500 ppm, but not 250 ppm, improved FCR values compared to the control, and a significant linear effect was observed in FCR at this time point. Both ADG and EPEF were significantly improved with increasing addition of QY, and both variables displayed significant linear responses to higher QY dosage.
Table 2. The effects of graded levels of QY on performance, average daily gain (ADG) and European Poultry Efficiency Factor (EPEF).1
In a similar manner, apparent total tract digestibility for dry matter, organic matter and crude ash were increased with each successive dose of QY. N retention demonstrated a similar response (). Crude fat digestibility showed significant improvements at the QY 500 ppm level only. For each digestibility variable, significant linear effects were observed, indicating that the magnitude of these responses was directly related to the dose level of QY.
Table 3. The effect of graded levels of QY on percent apparent total tract digestibility (ATTD) and percent nitrogen retention of broilers measured from days 21 to 25 in two pooled floor pen trials.1
illustrates the effects of graded levels of QY in the presence of enteric disease challenge. Body weight and FCR recorded at 21 d showed significant improvements with successive increases in QY dosage, and similar performance improvements were observed at d 42. ADG responded in a similar manner, and at day 21, both levels of QY significantly increased villus height compared to the control. Crypt depth was significantly reduced by QY treatments. In all variables, a significant linear relationship was found, indicating that, even in the presence of a significant disease challenge, the effects of QY were proportional to the dosage administered. To further illustrate the intestinal effects of QY, linear improvements in ileal villus height measured at d 21 were highly correlated (P < 0.001) to improvements in final body weights at 42 d ().
Table 4. The effects of graded levels of QY on interim performance, ileal morphometrics and final performance of coccidiosis vaccinated broilers reared under enteric disease challenge.1
Figure 1. The QY-induced improvements in villus height regressed against final body weight of coccidiosis-vaccinated broilers reared in an enteric disease challenge
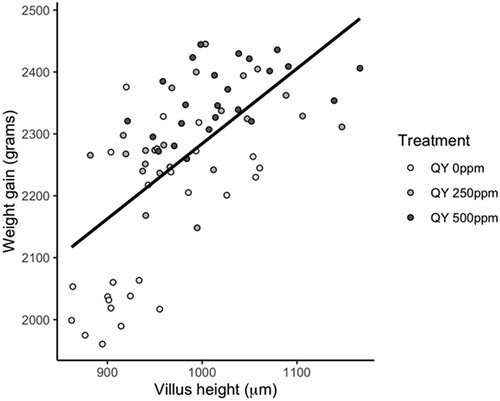
Discussion
Previous studies have shown that QY improves performance responses of broilers reared under the stress of intestinal disease challenge (Bafundo et al. Citation2020). Trial results indicated that at least part of this effect was associated with a lower level of coccidiosis in birds receiving QY. However, better coccidiosis control did not account for all the improvements recorded, and it was hypothesised that other intestinal effects may have been involved in these growth responses (Bafundo et al. Citation2021). The current data showed that QY produced general improvements in the apparent intestinal uptake of dry matter, organic matter, fats and minerals and N retention. Across the dosage range tested, all nutritional variables showed significant linear improvements, which indicated that digestibility was directly proportional to the QY dosage. The performance and productivity responses observed in the current studies were consistent with, and in part, likely the result of improvements in digestibility described above. Moreover, these agreed with a recent report, in which quillaja saponins were shown to improve body weights of broilers at 42 d (Youssef et al. Citation2020).
Saponin-containing combinations of quillaja and yucca are known to exert many effects in the intestine. Studies by Cheeke (Citation2000) and Francis et al. (Citation2002) have indicated that the biological effects of saponins are varied and have the ability to influence many disease organisms and host physiological processes. Many studies have shown that the integrity of biological membranes can be altered in the presence of quillaja/yucca saponins, and in many circumstances, effects in domestic animals have been associated with increased cellular permeability and changes in membrane porosity (Augustin et al. Citation2011; Fleck et al. Citation2018). This suggested that many intestinal effects of QY are brought about by changes in the microbial make-up of the intestinal milieu and/or alteration of the mucosa per se. Previous work has shown that quillaja saponins are capable of bacterial cell lysis (Hassan et al. Citation2010), and effects on S. aureus and other avian pathogens such as S. enterica Typhimurium and E. coli have been reported (Hassan Citation2008; Hassan et al. Citation2010). Many Gram-positive organisms can be affected as well (Hassan et al. Citation2010). These initial, yet unpublished, observations suggested that QY induced population changes among several bacterial genera that were indicative of broader changes to the intestinal microbiome. Because the microbiome plays such a critical role in the digestion and absorption of nutrients (Pan and Yu Citation2014), changes in bacterial populations could account for alterations in the efficiency of nutrient absorption. Clearly, additional research is needed on these topics, and this work, along with broader studies on important bacterial pathogens, is underway.
Recent research has shown that QY can impair the development of Eimeria spp. that damage the mucosal surface and adversely affect broiler performance (Bafundo et al. Citation2020). As has been shown in numerous studies, even subclinical levels of coccidial infection impair the ability of enterocytes to function effectively, resulting in reduced nutrient digestibility and depressed bird performance (Arczewska-Wlosek and Swiatkiewicz Citation2014; Rochell et al. Citation2016). It is, therefore, reasonable to conclude that due to the changes in populations of intestinal bacteria and reductions in coccidial replication, supplementing QY in feed can promote an intestinal tract that exhibits a greater propensity to absorb nutrients.
However, the current digestibility trials indicated that limitation of enteric disease is not always required for improvements in apparent digestibility to occur. Since QY positively affected N retention and fat and mineral digestibility in the absence of imposed disease challenge, more direct effects on the intestinal surface may be involved. This proposal is consistent with the findings of Johnson et al. (Citation1986) who demonstrated that the in vitro permeability of the intestinal mucosa is altered in the presence of saponins. These findings were of significance because they illustrated that large proteinaceous molecules can freely cross the intestinal barrier. This effect could have occurred as a result of a quillaja-mediated reduction in the electrochemical gradient (transmural potential difference) that drives active nutrient transport in intestinal epithelial cells (Johnson et al. Citation1986). According to these authors, reduction in this gradient increased the potential for molecular movement through the intestinal surface.
Consistent with this observation is the fact that quillaja saponins are often used as adjuvants in both human and veterinary vaccines (Fleck et al. Citation2018). They are used in this manner because they enhance the uptake of antigens from the gut (Kensil Citation1996). According to Barr et al. (Citation1998), this effect improved antigenic recognition by dendritic cells, which is considered one of the first steps in the development of mucosal immunity.
Numerous studies have evaluated intestinal morphometrics in order to gain a better understanding of intestinal health, and it is generally recognised that villus height is a good indicator of the functionality of the villus (Heak et al. Citation2017). Research has shown that mucous secretions and nutrient absorption increase as villus height improves (Paul et al. Citation2007; Heak et al. Citation2017); conversely, these effects decrease when crypt depth increases (Heak et al. Citation2017). Thus, elongated villi and reduced crypt depth are associated with improvements in body weight (Shamoto and Yamaguchi Citation2000). The current observations agreed with these findings, as ileal villus length was significantly improved by both levels of QY supplementation. Moreover, the QY-induced linear responses in villus height were highly correlated to body weights measured at 42 d of age. It is important to note that ileal measurements in these studies were carried out in coccidiosis vaccinated broilers reared under enteric challenge, where pathogenic effects in the midgut were known to occur. Thus, compared to controls, QY improved villus length and decreased crypt depth, and as previously shown by Heak et al. (Citation2017), produced gut conditions conducive to better digestibility and improved performance.
In conclusion, these trials demonstrated that QY supplementation exerted positive benefits on the intestinal tract of both healthy and disease challenged broilers. In addition to significant improvements in ileal villus height, the current results indicated that improved digestibility of key nutrients occurred and were associated with improved bird performance. While additional QY research is clearly warranted, these results provide a base from which a more complete understanding of the QY-mediated changes in the intestine occurs.
Acknowledgments
The technical contributions of Dr. Eliana Henriquez Rodriquez, Pen & Tec, Barcelona, Spain and Dr. James McNaughton, AHPharma, Hebron, Maryland, USA are gratefully acknowledged.
Disclosure statement
No potential conflict of interest was reported by the authors.
References
- Arczewska-Wlosek, A., and S. Swiatkiewicz. 2014. “Nutrition as a Modulatory Factor of the Efficacy of Live Coccidiosis Vaccines in Broiler Chickens.” World’s Poultry Science Journal 70: 81–92. doi:10.1017/S0043933914000075.
- Augustin, J., V. Kurian, S. B. Anderson, and S. Bakr. 2011. “Molecular Activities, Biosynthesis and Evolution of Triterpenoid Saponins.” Phytochemistry 72: 435–457. doi:10.1016/j.phytochem.2011.01.015.
- Bafundo, K. W., A. B. Johnson, and G. F. Mathis. 2020. “The Effects of a Combination of Quillaja Saponaria and Yucca Schidigera on Eimeria Spp. In Broiler Chickens.” Avian Diseases 64: 300–304. doi:10.1637/aviandiseases-D-20-00016.
- Bafundo, K. W., L. Gomez, B. Lumpkins, G. F. Mathis, J. McNaughton, and I. Duerr. 2021. “Concurrent Use of Saponins and Live Coccidiosis Vaccines: The Influence of a Quillaja/yucca Combination on Anticoccidial Effects and Performance Results of Vaccinated Broilers.” Poultry Science. doi:10.1016/j.psj.2020.12.010.
- Barr, I. G., A. Zoolander, and J. C. Cox. 1998. “ISCOMs and Other Saponin-based Adjuvants.” Advanced Drug Delivery Reviews 32: 247–271. doi:10.1016/S0169-409X(98)00013-1.
- Cheeke, P. R. 2000. “Actual and Potential Application of Yucca Schidigera and Quillaja Saponaria Saponins in Human and Animal Nutrition.” Journal of Animal Science 77: 1–10. doi:10.2527/jas2000.00218812007700ES0009x.
- De Mendiburu, F. 2020. “Agricolae: Statistical Procedures for Agricultural Research.” R package version 1.3-3. https://CRAN.R-project.org/package=agricolae
- Fleck, J. A., A. H. Bettis, F. P. Da Silva, E. A. Trion, C. Oliveros, F. Ferreira, and S. G. Verse. 2018. “Saponins from Quillaja Saponaria and Quillaja Brasiliensis: Particular Chemical Characteristics and Biological Activities.” Molecules 24: 271–299.
- Francis, G., Z. Kerem, H. P. S. Makkar, and K. Becker. 2002. “The Biological Action of Saponins in Animal Systems: A Review.” British Journal of Nutrition 88: 587–605. doi:10.1079/BJN2002725.
- Hassan, S. M. 2008. “Antimicrobial Activities of Saponin-rich Guar Meal”. PhD Diss., Texas A&M University, College Station, Texas.
- Hassan, S. M., J. A. Byrd, A. L. Cartwright, and C. A. Bailey. 2010. “Haemolytic and Antimicrobial Activities Differ among Saponin-rich Extracts from Guar, Quillaja, Yucca, and Soybean.” Applied Biochemistry and Biotechnology 162: 1008–1017. doi:10.1007/s12010-009-8838-y.
- Heak, C., P. Sukon, S. Kongpechr, B. Tengjaroenkul, and K. Chuachan. 2017. “Effect of Direct-fed Microbials on Intestinal Villus Height in Broiler Chickens: A Systematic Review and Meta-analysis of Controlled Trials.” International Journal of Poultry Science 16: 403–417. doi:10.3923/ijps.2017.403.414.
- Holm, S. 1979. “A Simple Sequentially Rejective Multiple Test Procedure.” Scandinavian Journal of Statistics 6: 65–70.
- Johnson, I. T., J. M. Gee, K. Price, C. Curl, and G. R. Fenwick. 1986. “Influence of Saponins on Gut Permeability and Active Nutrient Transport in Vitro.” Journal of Nutrition 116: 2270–2277. doi:10.1093/jn/116.11.2270.
- Kensil, C. R. 1996. “Saponins as Vaccine Adjuvants.” Critical Reviews in Therapeutic Drug Carrier Systems 13: 1–55.
- National Research Council. 1994. Nutrient Requirements of Poultry. 9th ed. Washington, D.C: National Academies Press.
- Oelschlager, M., M. Rasheed, B. Smith, M. Rincker, and R. Dilger. 2019. “Effects of Yucca Schidigera-derived Saponin Supplementation during a Mixed Eimeria Challenge in Broilers.” Poultry Science 98: 3212–3222. doi:10.3382/ps/pez051.
- Pan, D., and Z. Yu. 2014. “Intestinal Microbiome of Poultry and Its Interaction with Host and Diet.” Gut Microbes 5: 108–119. doi:10.4161/gmic.26945.
- Paul, S. K., G. Halder, M. Mondal, and G. Samanta. 2007. “Effect of Organic Acid Salt on the Performance and Gut Health of Broiler Chickens.” The Journal of Poultry Science 44: 389–395. doi:10.2141/jpsa.44.389.
- R Core Team. 2017. R: A Language and Environment for Statistical Computing. Vienna, Austria: R Foundation for Statistical Computing. https://www.R-project.org/.
- Rochell, S. J., C. M. Parsons, and R. N. Dilger. 2016. “Effects of Eimeria Acervulina Infection Severity on Growth Performance, Apparent Ileal Amino Acid Digestibility, and Plasma Concentrations of Amino Acids, Carotenoids, and α1-acid Glycoprotein in Broilers.” Poultry Science 95: 1573–1581. doi:10.3382/ps/pew035.
- Shamoto, K., and K. Yamaguchi. 2000. “Recovery Response of Chick Intestinal Villus Morphology to Different Refeeding Procedures.” Poultry Science 79: 718–723. doi:10.1093/ps/79.5.718.
- Short, F. J., P. Gorton, J. Wiseman, and K. N. Boorman. 1996. “Determination of Titanium Dioxide Added as an Inert Marker in Chicken Digestibility Studies.” Animal Feed Science Technology 59: 215–221. doi:10.1016/0377-8401(95)00916-7.
- Society of Nutritional Physiology. 1999. Empfehlungen Zur Energie- Und Nährstoffversorgung Der Legehennen Und Masthühner. Ausschuss Für Bedarfsnormen Der Gesellschaft Für Ernährungsphysiologie. Berlin, Germany: DLG-Verlags-GmbH.
- Sparg, S. G., L. E. Light, and J. Van Staden. 2004. “Biological Activities and Distribution of Plant Saponins.” Journal of Ethnopharmacology 94: 219–243.
- VDLUFA. 2013. Handbuch der landwirtschaftlichen Versuchs-und Untersuchungsmethodik, Methodenbuch Band VI-Chemische, physikalische und mikrobiologische Untersuchungsverfahren fur Milch Milchprodukte und Molkereihilfsstoffe. Darmstadt, Germany: VDLUFA-Verlag.
- Youssef, I., K. Manner, and J. Zentek. 2020. “Effect of Essential Oils or Saponins Alone or in Combination on Productive Performance, Intestinal Morphology and Digestive Enzymes’ Activity of Broiler Chickens.” Journal of Animal Physiology and Animal Nutrition 1–9. doi:10.1111/jpn.13431.