ABSTRACT
1. The role of the Harderian gland (HG), choanal cleft (CC) and turbinate in terms of IBV M41 viral load compared to the trachea, and immune (innate, cellular and mucosal) responses were studied in 21-day-old commercial broiler chickens.
2. After virulent IBV M41 challenge, the antigen concentration detected either by quantitative RT-PCR or immunohistochemistry peaked at 2–3 days post challenge (dpc) in all tissues. Significant increases of lachrymal IBV-specific IgA and IgY levels were found at 4–5 dpc.
3. Gene transcription showed a significant up-regulation of TLR3, MDA5, IL-6, IFN-α and IFN-β, where patterns and magnitude fold-change of mRNA transcription were dependent on the gene and tissue type.
4. The results demonstrated active IBV M41 replication in the HG, CC and turbinate, comparable to levels of replication found in the trachea. Data on immune-related genes in head-associated tissues provide further understanding on the immunobiology of IBV and offer opportunities to identify their use as quantitative biomarkers in pathogenicity and vaccination-challenge studies.
Introduction
Infectious bronchitis virus (IBV) is a highly contagious pathogen that causes respiratory, renal and reproductive diseases in chickens. It remains one of the main causes of economic losses in the poultry industry, affecting the production of meat and egg-laying chickens (Cavanagh Citation2007), health and welfare (DEFRA Citation2002; Lay et al. Citation2011). In pathogenesis and vaccination-challenge studies, the trachea has been traditionally highlighted as the primary tissue for IBV infection, immunity and pathology (McMartin Citation1993; Raj and Jones Citation1997). In 1976, the tracheal ciliostasis assay was introduced to assess vaccine efficacy (Cook et al. Citation1976), whereby vaccinated chicks were challenged with virulent IBV, and protection level was assessed based on cilia activity scores. It has been noted that respiratory clinical signs of sneezing, stuffiness and head-shaking could occur in vaccinated-challenged birds, even in the absence of ciliostasis (Awad et al. Citation2015; Chhabra et al. Citation2015a; Yang et al. Citation2018). This demonstrated that head-associated tissues, including the turbinate, Harderian gland (HG), and choanal cleft (CC) may play an important role in IBV immunobiology. These tissues are particularly important as virulent strains of IBV are mainly spread by aerosol routes.
Despite the apparent importance of the head-associated respiratory and lymphoid tissues, there have been limited attempts to understand the kinetics of viral load in association with the cellular and mucosal immune responses in IBV-challenged commercial broiler chicks. In studies that investigated the immune responses in the HG, lachrymal fluid or conjunctiva-associated lymphoid tissues (CALT) following exposure to IBV (Joiner et al. Citation2007; van Ginkel et al. Citation2008, Citation2012; Gurjar et al. Citation2013), measured innate immune indicators, were limited. Furthermore, in most studies, an IBV Arkansas strain was used rather than a Massachusetts strain (Jackwood Citation2012). The majority of studies investigating protection (Okino et al. Citation2013; Awad et al. Citation2015a, b, Citation2016) and immune responses (Wang et al. Citation2006; Kameka et al. Citation2014; Okino et al. Citation2014, Citation2017; Zegpi et al. Citation2020a) relied on changes in the trachea alone. To date, little information is available on the viral load and immune responses in the turbinate, HG and CC tissues, which are challenged by invading IBVs before the trachea.
For IBV, although expression of a number of gene targets have been examined before (Okino et al. Citation2014), a selected panel of dominant early immune genes were included in this study, including TLR3, MDA5, IFN-α, IFN-β and IL-6. Stimulation of TLR receptors in ovo has been shown to reduce viral titres of IBV (Sharma et al. Citation2020). Melanoma differentiation-associated protein 5 (MDA5) detects long-duplex RNAs or dsRNA of positive-strand viruses (Wu et al. Citation2013), and cytoplasmic viral RNA, to induce an antiviral immune response, such as interferon production (Yu et al. Citation2017). The stimulation of TLRs leads to the transcription of genes encoding type I interferons such as interferon-alpha (IFN-α) and interferon-beta (IFN-β) (Sharma et al. Citation2020). Chickens challenged with an attenuated IBV strain (H120) showed an increased expression of interferons and pro-inflammatory cytokine (IFN-α, IFN-β and IL-1β) genes in tracheal tissues (Guo et al. Citation2008). Okino et al. (Citation2014) reported that IL-6 is involved in the activation of innate immune responses in the trachea at the early phase of IBV infection.
In understanding the role of mucosal immune responses to IBV, IgA levels in lachrymal fluid have been evaluated following virulent challenge (Gillette Citation1980; Raj and Jones Citation1996; Ganapathy et al. Citation2005; van Santen et al. Citation2006) or vaccination (Toro et al. Citation1994; Joiner et al. Citation2007; van Ginkel et al. Citation2008; Orr-Burks et al. Citation2014; Chhabra et al. Citation2015b; Zegpi et al. Citation2020b). Levels of IgA in lachrymal fluid were more closely associated with protection (Raggi and Lee Citation1965; Davelaar et al. Citation1982; Ignjatovic and McWaters Citation1991; Gelb et al. Citation1998). The inhibitory effect of lachrymal fluid against IBV has been reported before (Toro and Fernandez Citation1994). Previous studies reporting lachrymal IgA were mostly conducted in SPF chicks that were infected at different ages, such as at 1- or 7- days-old (van Santen et al. Citation2006; Abaidullah et al. Citation2021), 28 d old (Ganapathy et al. Citation2005) or older (Gillette Citation1980). Lachrymal samples were collected at longer intervals. For example, every three days (Toro and Fernandez Citation1994; van Santen et al. Citation2006) or weekly sampling (Ganapathy et al. Citation2005; Abaidullah et al. Citation2021). There have been no attempts to study the daily kinetics of IgA levels in the lachrymal fluid to improve the understanding of the early immune induction. Thus, it is worthwhile to investigate the role of these tissues, compared to that of the trachea, in naïve (unvaccinated) commercial broiler chickens challenged with a virulent IBV.
The following study reported on the viral load and early immune responses in selected head-associated and lymphoid tissues (HALT) and respiratory tissues of 21-day-old commercial broiler chickens experimentally challenged with IBV M41 (lineage GI-1).
Material and methods
Ethical statement
All experimental procedures were performed according to the UK legislation governing experimental animals under the project licence P8E4FC2C9. Experimental procedures were approved by the University of Liverpool’s ethical review process.
Animals
One-day-old broiler chicks were obtained from a commercial hatchery and kept according to animal welfare guidelines and biosecurity measures at the University of Liverpool. Chicks were reared on deep litter with antibiotic-free feed and water provided ad libitum. All procedures were undertaken according to the UK legislation on the use of experimental animals.
The IBV M41 (Massachusetts) serotype was propagated in 10-day-old embryonated SPF chicken eggs at 37°C, and virus rich allantoic fluid was harvested 48 h after infection. The viral titre was determined as the 50% ciliostatic dose (CD50/ml; Reed and Muench Citation1938). The RT-PCR analysis confirmed that the virus inoculum was free from other poultry viruses, and culture confirmed it free of bacterial or fungal contaminants (Chhabra et al. Citation2018).
Experimental design
At 21-days-old, the birds were divided into two groups: unchallenged (control, n = 25) and challenged (n = 25). Chickens received either 0.1 ml of virus-free allantoic fluid (unchallenged) or 0.1 ml of 105.75 CD50/bird of virulent M41 virus (challenged) via the oculonasal route. At 1, 2, 3, 4 and 5 dpc, clinical signs were recorded. At the same time points, five birds were sampled for lachrymal fluid to assay for anti-IBV IgA and IgY by indirect ELISA. Blood was collected via the brachial vein for antibody detection. Serum was separated from the whole blood and stored at −20°C. The same five birds were humanely euthanised according to UK Home Office regulations, and tracheal tissues were collected for the ciliostasis assay. The HG, turbinate, choanal cleft and trachea samples were collected and stored in RNALater (Qiagen, Crawley, UK) for subsequent determination of viral load and host gene expression.
Evaluation of tracheal health
Sections of the upper, middle and lower trachea were collected from each bird during necropsy and processed for ciliary protection scoring as described previously (Awad et al. Citation2016). The mean ciliary score per bird was used to calculate percentage protection for each group (Cook et al. Citation1999; Awad et al. Citation2016).
Humoral immune responses by ELISA
Sera was analysed using a commercial IBV ELISA kit (IDEXX, Westbrook, Maine, USA) to determine anti-IBV antibodies from all groups according to the manufacturer’s guidelines. Samples with a sample/positive ratio greater than 0.2 were considered positive. Antibody titres were calculated by converting the sample/positive ratio according to the formula provided by the manufacturer, with a positive ELISA titre cut-off determined as 396.
Mucosal immune responses by indirect ELISA
Lachrymal fluid was assayed for IBV-specific IgA and IgY using an indirect ELISA (Mockett and Cook Citation1986; Raj and Jones Citation1996; Ganapathy et al. Citation2005). For the assay, each well of a flat bottom 96-well microplate (STARLAB®, UK) was coated with 100 µl of 2.5 µg/ml IBV M41 antigen (viral challenge) in 50 mM sodium carbonate/bicarbonate (pH 9.6) coating buffer, incubated for 1 h at 37°C, and then overnight at 4°C. Each well was blocked with 200 µl phosphate buffer saline (PBS) containing 3.0% non-fat skimmed milk powder. Lachrymal fluid samples were tested in triplicate at a single dilution of 1:10 in PBS containing 0.05% tween-20 (PBST) (Sigma Aldrich®, Dorset, UK). Mouse monoclonal antibodies against either chicken IgA or IgY (BIO-RAD®, Hertfordshire, UK) were added at a dilution of 1:1,000 (50 µl) as the secondary antibody, and incubated for an hour at 37°C. This was followed by goat anti-mouse IgG horse-radish peroxidase-conjugate (BIO-RAD®) at a dilution of 1:10,000 (50 µl), and one hour incubation at 37°C. Tetramethylbenzidine (TMB) (Sigma Aldrich®, UK) substrate was added to each well (50 µl) and incubated in the dark for 15 min to allow for colour development. The reaction was stopped by adding 50 µl of sodium hydrochloric acid (0.5 M HCL), and plates were analysed at 450 nm. Corrected optical density (COD) values were calculated by deducting the OD values of non-antigen coated (blank) wells from those of the test wells (Fournier-Caruana et al. Citation2003; Ganapathy et al. Citation2005).
Quantification of the viral genome by reverse transcriptase quantitative polymerase chain reaction (RT-qPCR)
From 30 mg of each sampled tissue, viral RNA was extracted according to manufacturer’s instructions using the RNeasy Plus Mini kit (Qiagen, UK). Quantification of viral RNA was carried out by quantitative real-time RT-PCR (RT-qPCR), using an IBV 3’ untranslated region (UTR) gene-specific primer and probe as described previously (Chhabra et al. Citation2018). Obtained Ct values were converted to log relative equivalent units (REU) of viral RNA by a standard curve generated from using five 10-fold dilutions of RNA extracted from M41 virus-positive allantoic fluid (Ball et al. Citation2016).
Immunohistochemistry
For detection of IBV antigen, sampled tissues were immediately stored in cryoembedding compound (OCT; Solmedia laboratory, Shrewsbury, UK) and frozen in liquid nitrogen (−190°C). Samples were immunostained using anti-IBV nucleoprotein monoclonal antibody (Prionics, Lelystad, Netherlands) as previously described (Chhabra et al. Citation2015a). Stained cells were counted in three microscopic fields at 40x magnification. Antigen scores in each tissue were expressed as the mean of infected cell counts and scored as follows: 0 = Negative, 1 = Mild, 2 = Moderate, 3 = High (Oladele et al. Citation2009).
Measurement of host gene expression
Based on previous work, extracted RNA was tested by quantitative real-time RT-PCR for expression of a pro-inflammatory cytokine (IL-6), pattern recognition receptors of the innate immune system (TLR3 and MDA5) and interferons (IFN-α and IFN-β) (Chhabra et al. Citation2016, Citation2018; Okino et al. Citation2017). Each cDNA sample was tested in triplicate using LightCycler 480 SYBR Green I Master mix and gene specific primers (). Data were normalised using a relative standard curve method to 18S ribosomal RNA expression (Chhabra et al. Citation2015a; Kuchipudi et al. Citation2012), and data was presented as the fold difference in gene expression of challenged versus unchallenged samples.
Table 1. Primers used in the RT-qPCR analysis of gene transcription signatures.
Data analysis and statistics
Data were confirmed to be normally distributed, and analysed using one-way analysis of variance (ANOVA), followed by the post-hoc LSD multiple comparison using GraphPad™ Prism version 6.00. Differences between groups were considered significant at P < 0.05, unless stated otherwise.
Results
Clinical signs and gross lesions
No clinical signs were seen in the unchallenged control group during the experimental period. The M41-challenged chickens exhibited mild clinical signs at 3–5 dpc, which consisted of râles, coughing and sneezing. At necropsy, gross lesions in the trachea involved congestion and excess mucous at 3 and 5 dpc.
Tracheal ciliary health
The challenged group showed a decline in the average ciliary activity from 90% at 1 dpc to 1.5% by 5 dpc, whereas the unchallenged control group remained above 99% for all sampling days ().
Figure 1. Tracheal ciliary activity in the control and challenged chickens in the period of 1 to 5 days post challenge. Dark grey = Control, Light grey = Challenged. Data is shown as the percentage of cilia activity from ten birds sampled per time point according to (Cook et al. Citation1999).
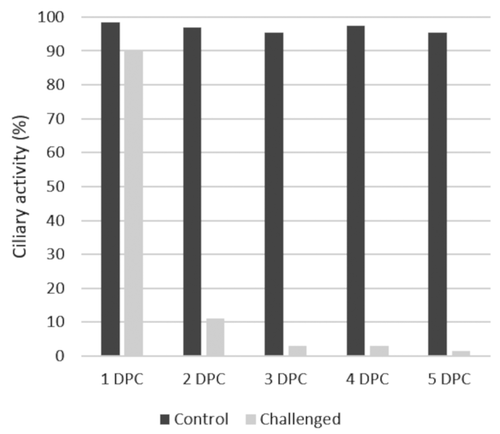
Humoral immune responses by ELISA
The mean titre of maternally derived antibodies (MDA) of the day-old chicks was 1376 ± 386 (Figure S1). At 26 d of age, both the unchallenged (control) and challenged (five days post M41-challenge) groups had a negative ELISA result, with titres of 21 and 90.6 respectively. No significant difference was noted between the challenged and control group.
Mucosal immune responses by monoclonal IgA or IgY ELISA
Levels of anti-IBV-specific IgA and IgY gradually increased in lachrymal fluid from 1 to 5 dpc after M41 infection. Compared to the control group, significantly higher levels of both IgA and IgY were detected at 4 dpc (IgA – 0.095 COD; IgY – 0.053 COD) and 5 dpc (IgA – 0.142 COD; IgY – 0.071 COD) for the IBV M41 challenged group (). By 5 dpc, IgY was significantly higher when compared to 1 dpc for the challenged group, with no changes in the unchallenged group.
Figure 2. Measurement of IBV-specific (a) IgA and (b) IgY levels using indirect ELISA from lachrymal fluid collected at 1–5 dpc. Data expressed as mean of corrected optical density (COD) from five birds per time point. Dark grey = Control, Light grey = Challenged. Standard error margins are indicated with shading. Significantly different IgA or IgY levels at the same time point (1–5 dpc), are indicated by an asterisk.
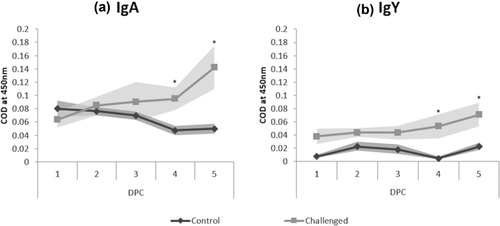
Viral load in tissues by RT-qPCR
Viral RNA was not detected in any tissues from the control group (unchallenged), whereas samples collected from challenged birds were positive on all sampling days ()). The viral load in the turbinate peaked at 3 dpc (3.64 log REU) and showed a significant decrease between 3 and 4 dpc. Viral load peaked earlier in HG and tracheal tissues at 2 dpc (3.57 log REU and 3.92 log REU respectively). A significant increase in viral load was noticed at 2–4 dpc in the HG, and at 2–3 dpc in the trachea, when compared to 1 dpc. When comparing tissue types, there was a significantly lower viral load in the choanal cleft at 1 dpc in comparison to other tissues. By 5 dpc, the trachea and HG had significantly lower log REU values compared to the turbinate and choanal cleft ()).
Figure 3. (a) Quantification of viral RNA expressed as a log relative equivalent units (REU) in M41-challenged chickens. Data is represented as the mean of five birds ± standard error margins. Significantly different sampling days of the same tissue type are indicated with an asterisk (p < 0.05). (b) Immunohistochemistry (IHC) staining scores of IBV M41 virus in the harderian gland, choanal cleft, turbinate and trachea tissues. Data is represented as the mean of 5 birds. Significantly different sampling days of the same tissue type are indicated with an asterisk (p < 0.05).
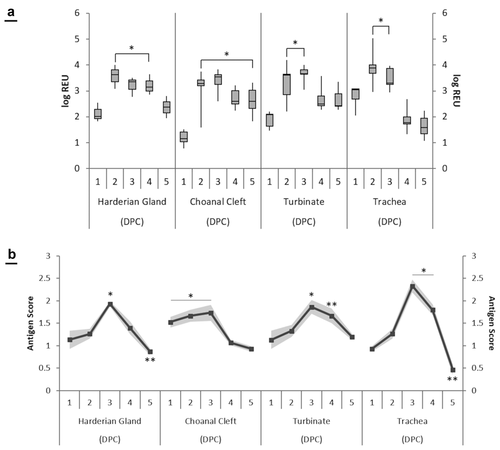
At 3 dpc, antigen scores in the HG, turbinate, choanal cleft and trachea tissues peaked at 1.93, 1.86, 1.73 and 2.33 respectively ()). There was a significant increase at 3 dpc compared to all other sampling days in the HG. In the turbinate, there was a significant increase of 1.13 and 1.86 between 1 and 3 dpc respectively. In the choanal cleft, there was a significant decrease of antigen score after 3 dpc, whereas the trachea showed a significant increase at the same time point. The only significant difference between tissue types was seen at 5 dpc, as the trachea had a significant decrease in stained antigen (0.46) compared to the HG (0.87), turbinate (1.20) and choanal cleft (0.93).
Quantification of host immune response mRNA gene transcripts
For the HG, compared with the control group, there was significant up-regulation in mRNA expression of TLR3 at 2 dpc and prolonged transcription of MDA5 at 2–5 dpc ().
Figure 4. Expression profiles of host genes (TLR3, MDA5 and IL-6) in challenged chickens compared to the control group. Data is shown as the difference in fold change when compared to the unchallenged control group. Significant changes between challenged and unchallenged groups per sampling point are shown with an asterisk (p < 0.05).
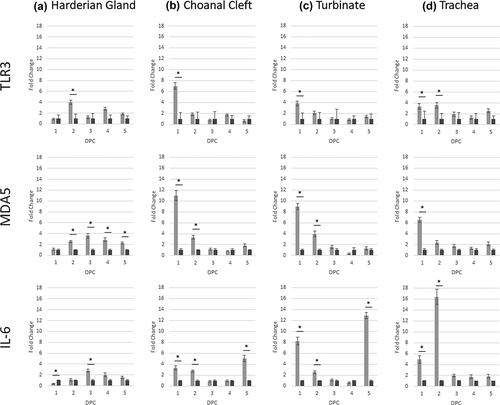
Expression of IL-6 mRNA was significantly down-regulated 1 dpc, and gradually increased to significant up-regulation at 3 dpc. The peak of expression for TLR3, MDA5 and IL-6 was at 2–3 dpc, and expression then declined until 5 dpc (). The only significant change for IFN-α was up-regulation at 3 dpc, whereas IFN-β had a prolonged up-regulation at 2–4 dpc.
Figure 5. Expression profiles of host genes (IFN-α and IFN-β) in challenged chickens compared to the control group. Data is shown as the difference in fold change when compared to the unchallenged control group. Significant changes between challenged and unchallenged groups per sampling point are shown with an asterisk (p < 0.05).
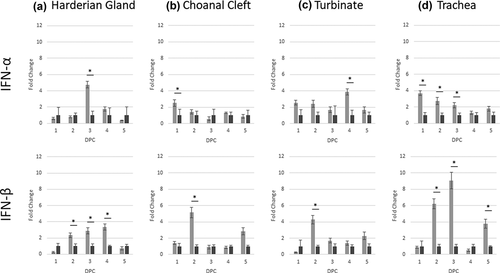
In the choanal cleft, following challenge TLR3 expression was significantly up-regulated at 1 dpc, and MDA5 and IL-6 at 1–2 dpc (). In addition, IL-6 was also up-regulated at 5 dpc compared to the control group. For IFN-α, there was only a significant increase at 1 dpc, whereas a later response was identified for IFN-β at 2 dpc (). No other changes were noted.
In the turbinate, gene transcription profiles were identical to the choanal cleft for TLR3, MDA5 and IL-6, with up-regulation determined at 1 dpc (TLR3), 1–2 dpc (MDA5) and 1, 2 and 5 dpc (IL-6). The IFN-α response occurred at a later timepoint for the turbinates at 4 dpc, whereas IFN-β response was identical to the choanal cleft at 2 dpc only ().
Up-regulation of TLR3 and IL-6 mRNA expression in the trachea was significant at 1–2 dpc, with MDA5 only significant at 1 dpc (). Prolonged significant up-regulation of IFN-α was identified at 1–3 dpc, with IFN-β up-regulated at 2, 3 and 5 dpc ().
Discussion
Past studies have demonstrated host–virus interactions using virulent and vaccinal IBV strains, however, the majority of investigations have largely concentrated on the trachea (Okino et al. Citation2013; Zhang et al. Citation2017; Yang et al. Citation2018). To date, limited information is available on the role of the turbinate and choanal cleft, although these tissues tend to be exposed to IBV prior to the trachea. In an attempt to further characterise the innate and cellular immune response to IBV, a select number of immune gene transcripts in head-associated lymphoid and respiratory tissues were examined. Findings in these tissues for the first five days of infection were then compared to the trachea. In addition, as a measurement of mucosal immunity, levels of IgA and IgY in lachrymal fluid were investigated. Based on past studies (Chhabra et al. Citation2016; Ball et al. Citation2019), the M41 virus used in this study causes substantial ciliostasis by 3–5 dpc. Thus, data collected up to 5 dpc should be sufficient to identify potential quantitative parameter(s) when challenge or vaccination-challenge studies are carried out in the future.
In previous work studying the pathogenesis of IBV, emphasis was often given to the trachea (Hawkes et al. Citation1983; Otsuki et al. Citation1990; Smith et al. Citation2015; Yang et al. Citation2018). Although the tracheal epithelium is an important site for replication, in natural or experimental infection, IBV first progresses through tissues in the head and mouth, including the turbinate and choanal cleft. The current study has demonstrated increased viral loads in the turbinate, HG and choanal cleft tissues at 2–3 dpc, which indicated localised infection and persistence of IBV at these tissues. Previous publications using virus isolation have demonstrated peak virus yield between 1 and 3 dpc in the nasal turbinate, and between 1 and 2 dpc in the trachea (Darbyshire et al. Citation1978; Dolz et al. Citation2012). As viral load was measured by RT-qPCR rather than viral isolation, this may not necessarily indicate localised virus replication. To ensure that findings were due to local replication of the virus in these tissues, IHC was undertaken. Both the viral load by RT-qPCR and detection by IHC were closely associated in terms of pattern and degree of antigen detection, with a peak detection at 3 dpc in all tissues. Findings from the current study have shown for the first time that IBV effectively replicates in the turbinate and choanal cleft tissues, and that the viral load, based on the S1 gene, was comparable to that of the HG and trachea tissues. In future IBV challenge studies, it would be beneficial to include the turbinate, HG and choanal cleft, in addition to the trachea, as tissues of choice for virus detection.
This was the first experiment to closely examine the early innate, cellular, mucosal and humoral immune responses on a daily basis in M41-challenged commercial broiler chicks. With the dosage used for the M41-challenge, previous publications in our laboratory have shown more than 90% ciliostasis by 5 dpc (Chhabra et al. Citation2016; Ball et al. Citation2019). Concurring with this, the current study showed more than 90% ciliostasis was evident as early as 3 dpc. To identify a quantitative biomarker for the pathogenicity of M41, disease and immune kinetics, including changes in lachrymal fluid, were closely monitored up to 5 dpc. The role of IBV-specific IgA and IgY in lachrymal fluid providing protection against virulent strains of IBV has been documented before (Raj and Jones Citation1996; Eldemery et al. Citation2017; Zegpi et al. Citation2020a). In the current study, significantly higher levels of lachrymal IBV-specific IgA antibodies were noted at 4 and 5 dpc. In addition, patterns of IBV-specific IgY levels closely followed IgA, although COD values were substantially lower for IgY. This was similar to previous reports in SPF chickens that had been vaccinated with IBV (Toro et al. Citation1994). In previous experiments measuring IBV-specific IgA levels in chickens challenged with a non-M41 strain (Toro and Fernandez Citation1994; Gelb et al. Citation1998), mucosal immunity was not monitored on a daily basis. The breed/type of chicken used in the current study was different to previous work, as they used specific pathogen free birds. Findings from this study suggested that, as early as 4–5 dpc, both IBV-specific IgA and IgY in lachrymal fluid can be used as important indicators of mucosal immune responses of IBV M41 infection in commercial broiler chickens.
Past publications highlighted the benefit of analysing TLR3, MDA5, IFN-α, IFN-β and IL-6 to better understand the immunopathogenesis of vaccine or virulent IBVs (Wang et al. Citation2006; Kameka et al. Citation2014; Okino et al. Citation2017; Chhabra et al. Citation2018), but examination was almost always confined to the trachea and occasionally, the kidneys. To explore host transcription response in tissues in the passage of IBV M41 before reaching trachea, the current trial examined the daily kinetics of early viral load and immune responses in tissues of turbinate, HG, choanal cleft and trachea. Transcriptional data showed up-regulation of TLR3, MDA5 and IL-6 for all tissues, with the exception of IL-6 in the HG at 1 dpc. Analysis showed that pattern and magnitude of fold-change for each respective gene differs based on tissue type and dpc. In general, for TLR3 and MDA5, up-regulation occurred at 1–2 dpc, except for MDA5 in the HG where up-regulation persisted from 2 to 5 dpc. Tracheal mRNA expression was similar to previous reports (He et al. Citation2016; Okino et al. Citation2017; Chhabra et al. Citation2018) but contradicted the findings of Wang et al. (Citation2006), who found that TLR3 expression increased at 3 dpc following M41 challenge. For IL-6, there was no set pattern for up-regulation in the HG, choanal cleft and turbinate, whereas this lasted from 1 to 2 dpc in the trachea. It is possible that severe tracheal deciliation (ciliostasis) from 3 to 5 dpc may have impacted the IL-6 expression, or transcription may have subsided. The findings of IL-6 in the trachea agreed with previous studies in different chicken lines following infection with IS/885/00-like, QX-like, IBV/Brazil/PR05 or M41 IBV strains (Fernando et al. Citation2015; Asif et al. Citation2007; Chhabra et al. Citation2018). Interleukin-6 has been shown to play a central role in the activation of the innate response following infection (Okino et al. Citation2014), and has been associated with tissue damage, increased viral load and tissue tropism (Chhabra et al. Citation2018).
Type I IFN response is an essential defence mechanism against viruses (Qu et al. Citation2013). Interferon-β is the end product of TLR3 and MDA5 pathways, and early induction of interferon promotes up-regulation of genes encoding interferon-inducible transmembrane protein (IFITM) 1, 2, 3 and 5 in the trachea (Steyn et al. Citation2020), which are known to possess antiviral properties (Zhao et al. Citation2018). Suppression of the host IFN innate immune response following IBV infection has been previously reported (Kint et al. Citation2016), and this was evident in the HG in the current study at 1 dpc. However, in the challenged group, IFN-α and/or IFN-β were significantly up-regulated during the early sampling days (1–3 dpc) in all other tissues. This response was prolonged in the HG (IFN-β; 4 dpc) and trachea (IFN-β; 5 dpc). For IFN-α, there was a surge of expression in turbinate tissue at 4 dpc. These findings were similar to those reported by Okino et al. (Citation2017) after a challenge with two Brazilian IBV strains, and those reported by Chhabra et al. (Citation2018) following infection with IS/885/00-like, QX-like and M41 IBV strains (Okino et al. Citation2017; Chhabra et al. Citation2018). However, while these researchers used SPF chicks, the current study used commercial broiler chickens. Although data emphasised transcriptional up-regulation in naïve birds, it would be interesting to explore the possibility of including one or more of these early immune mediator responses in vaccinated-M41 challenged chickens, as the pattern and/or magnitude of fold changes could be useful in identifying quantitative vaccination-protection biomarkers.
Viral load and immune responses in the turbinate, HG and choanal cleft showed that tissues other than the trachea should be considered in IBV immunopathogenesis studies. Findings from this study showed active IBV replication in head-associated lymphoid (HG and choanal cleft) and turbinate tissues, and the limited subset of immunity-related genes provided further understanding on the immunobiology of IBV in naïve 21-day-old commercial broiler chickens. Such effects were dependent on the tissue type, with significant changes in TLR3, MDA5, IFN-α and IL-6 mRNA expression in the turbinate and trachea being most notable. Importantly, the data highlighted the significant presence of both IgA and IgY in lachrymal fluid following IBV M41 challenge. Further work is in-progress to assess if one or more of the immune parameters discussed above could be used as quantitative biomarkers in vaccination-challenge studies.
Supplementary Figure 1. Measurement of anti-IBV antibodies using a commercial IBV ELISA kit (IDEXX, UK).
Download TIFF Image (182.2 KB)Acknowledgments
Mohammed Al-Rasheed is in receipt of a studentship from the Saudi Arabia government. The funders had no involvement with the study design, analysis or decision to publish the data.
Disclosure statement
No potential conflict of interest was reported by the author(s).
Supplementary material
Supplemental data for this article can be accessed here.
Additional information
Funding
References
- Abaidullah, M., S. Peng, X. Song, Y. Zou, L. Li, R. Jia, and Z. Yin. 2021. “Chlorogenic Acid Is a Positive Regulator of MDA5, TLR7 and NF-κB Signaling Pathways Mediated Antiviral Responses against Gammacoronavirus Infection.” International Immunopharmacology 96: 107671. doi:10.1016/j.intimp.2021.107671.
- Asif, M., J. W. Lowenthal, M. E. Ford, K. A. Schat, W. G. Kimpton, and A. G. Bean. 2007. “Interleukin-6 Expression after Infectious Bronchitis Virus Infection in Chickens.” Viral Immunology 20 (3): 479–486. doi:10.1089/vim.2006.0109.
- Awad, F., A. Forrester, M. Baylis, S. Lemiere, K. Ganapathy, H. A. Hussien, and I. Capua. 2015. “Protection Conferred by Live Infectious Bronchitis Vaccine Viruses against Variant Middle East IS/885/00-like and IS/1494/06-like Isolates in Commercial Broiler Chicks.” Veterinary Record Open 2 (2). doi:10.1136/vetreco-2014-000111.
- Awad, F., S. Hutton, A. Forrester, M. Baylis, and K. Ganapathy. 2016. “Heterologous Live Infectious Bronchitis Virus Vaccination in Day-old Commercial Broiler Chicks: Clinical Signs, Ciliary Health, Immune Responses and Protection against Variant Infectious Bronchitis Viruses.” Avian Pathology 45 (2): 169–177. doi:10.1080/03079457.2015.1137866.
- Ball, C., S. Bennett, A. Forrester, and K. Ganapathy. 2016. “Genetic Mutations in Live Infectious Bronchitis Vaccine Viruses following Single or Dual in Vitro Infection of Tracheal Organ Cultures.” Journal of General Virology 97 (12): 3232–3237. doi:10.1099/jgv.0.000628.
- Ball, C., A. Forrester, A. Herrmann, S. Lemiere, and K. Ganapathy. 2019. “Comparative Protective Immunity Provided by Live Vaccines of Newcastle Disease Virus or Avian Metapneumovirus When Co-administered Alongside Classical and Variant Strains of Infectious Bronchitis Virus in Day-old Broiler Chicks.” Vaccine 37 (52): 7566–7575. doi:10.1016/j.vaccine.2019.09.081.
- Cavanagh, D. 2007. “Coronavirus Avian Infectious Bronchitis Virus.” Veterinary Research 38 (2): 281–297. doi:10.1051/vetres:2006055.
- Chhabra, R., J. Chantrey, and K. Ganapathy. 2015a. “Immune Responses to Virulent and Vaccine Strains of Infectious Bronchitis Viruses in Chickens.” Viral Immunology 28 (9): 478–488. doi:10.1089/vim.2015.0027.
- Chhabra, R., A. Forrester, S. Lemiere, F. Awad, J. Chantrey, and K. Ganapathy. 2015b. “Mucosal, Cellular, and Humoral Immune Responses Induced by Different Live Infectious Bronchitis Virus Vaccination Regimes and Protection Conferred against Infectious Bronchitis Virus Q1 Strain.” Clinical Vaccine Immunology 22 (9): 1050–1059. doi:10.1128/cvi.00368-15.
- Chhabra, R., S. V. Kuchipudi, J. Chantrey, and K. Ganapathy. 2016. “Pathogenicity and Tissue Tropism of Infectious Bronchitis Virus Is Associated with Elevated Apoptosis and Innate Immune Responses.” Virology 488: 232–241. doi:10.1016/j.virol.2015.11.011.
- Chhabra, R., C. Ball, J. Chantrey, and K. Ganapathy. 2018. “Differential Innate Immune Responses Induced by Classical and Variant Infectious Bronchitis Viruses in Specific Pathogen Free Chicks.” Developmental and Comparative Immunology 87: 16–23. doi:10.1016/j.dci.2018.04.026.
- Cook, J. K., J. H. Darbyshire, and R. W. Peters. 1976. “The Use of Chicken Tracheal Organ Cultures for the Isolation and Assay of Avian Infectious Bronchitis Virus.” Archives of Virology 50 (1–2): 109–118. doi:10.1007/BF01318005.
- Cook, J. K., S. J. Orbell, M. A. Woods, and M. B. Huggins. 1999. “Breadth of Protection of the Respiratory Tract Provided by Different Live-attenuated Infectious Bronchitis Vaccines against Challenge with Infectious Bronchitis Viruses of Heterologous Serotypes.” Avian Pathology 28 (5): 477–485. doi:10.1080/03079459994506.
- Darbyshire, J. H., J. K. A. Cook, and R. W. Peters. 1978. “Growth Comparisons of Avian Infectious Bronchitis Virus Strains in Organ Cultures of Chicken Tissues.” Archives of Virology 56 (4): 317–325. doi:10.1007/BF01315282.
- Davelaar, F. G., A. Noordzij, and J. A. Vanderdonk. 1982. “A Study on the Synthesis and Secretion of Immunoglobulins by the Jarderian Gland of the Fowl after Eyedrop Vaccination against Infectious Bronchitis at 1‐day‐old.” Avian Pathology 11 (1): 63–79. doi:10.1080/03079458208436082.
- DEFRA. 2002. “Meat Chickens and Breeding Chickens. Code of Recommendations for the Welfare of Livestock.” PB7275.
- Dolz, R., J. Vergara-Alert, M. Perez, J. Pujols, and N. Majo. 2012. “New Insights on Infectious Bronchitis Virus Pathogenesis: Characterization of Italy 02 Serotype in Chicks and Adult Hens.” Veterinary Microbiology 156 (3–4): 256–264. doi:10.1016/j.vetmic.2011.11.001.
- Eldemery, F., K. S. Joiner, H. Toro, and V. L. van Santen. 2017. “Protection against Infectious Bronchitis Virus by Spike Ectodomain Subunit Vaccine.” Vaccine 35 (43): 5864–5871. doi:10.1016/j.vaccine.2017.09.013.
- Fernando, F. S., C. H. Okino, K. R. Silva, C. C. Fernandes, M. C. M. Gonçalves, M. F. S. Montassier, R. O. Vasconcelos, and H. J. Montassier. 2015. “Increased Expression of Interleukin-6 Related to Nephritis in Chickens Challenged with an Avian Infectious Bronchitis Virus Variant.” Pesquisa Veterinária Brasileira 35 (3): 216–222. doi:10.1590/S0100-736X2015000300002.
- Fournier-Caruana, J., B. Poirier, G. Haond, C. Jallet, F. Fuchs, N. Tordo, and P. Perrin. 2003. “Inactivated Rabies Vaccine Control and Release: Use of an ELISA Method.” Biologicals 31 (1): 9–16. doi:10.1016/S1045-1056(02)00070-2.
- Ganapathy, K., P. W. Cargill, and R. C. Jones. 2005. “A Comparison of Methods of Inducing Lachrymation and Tear Collection in Chickens for Detection of Virus-specific Immuoglobulins after Infection with Infectious Bronchitis Virus.” Avian Pathology 34 (3): 248–251. doi:10.1080/03079450500112344.
- Gelb, J., Jr, W. A. Nix, and S. D. Gellman. 1998. “Infectious Bronchitis Virus Antibodies in Tears and Their Relationship to Immunity.” Avian Diseases 42 (2): 364–374. doi:10.2307/1592487.
- Gillette, K. G. 1980. “Avian Infectious Bronchitis in Specific-pathogen-free Chickens: Quantitation of Serum Immunoglobulins by Electroimmunoassay.” Avian Diseases 24 (2): 345–357. doi:10.2307/1589702.
- Guo, X., A. J. Rosa, D. G. Chen, and X. Wang. 2008. “Molecular Mechanisms of Primary and Secondary Mucosal Immunity Using Avian Infectious Bronchitis Virus as a Model System.” Veterinary Immunology and Immunopathology 121 (3–4): 332–343. doi:10.1016/j.vetimm.2007.09.016.
- Gurjar, R. S., S. L. Gulley, and F. W. van Ginkel. 2013. “Cell-mediated Immune Responses in the Head-associated Lymphoid Tissues Induced to a Live Attenuated Avian Coronavirus Vaccine.” Developmental & Comparative Immunology 41 (4): 715–722. doi:10.1016/j.dci.2013.08.002.
- Hawkes, R. A., J. H. Darbyshire, R. W. Peters, A. P. A. Mockett, and D. Cavanagh. 1983. “Presence of Viral Antigens and Antibody in the Trachea of Chickens Infected with Avian Infectious Bronchitis Virus.” Avian Pathology 12 (3): 331–340. doi:10.1080/03079458308436175.
- He, Y., Z. Xie, J. Dai, Y. Cao, J. Hou, Y. Zheng, T. Wei, M. Mo, and P. Wei. 2016. “Responses of the Toll-like Receptor and Melanoma Differentiation-associated Protein 5 Signaling Pathways to Avian Infectious Bronchitis Virus Infection in Chicks.” Virologica Sinica 31 (1): 57–68. doi:10.1007/s12250-015-3696-y.
- Ignjatovic, J., and P. G. McWaters. 1991. “Monoclonal Antibodies to Three Structural Proteins of Avian Infectious Bronchitis Virus: Characterization of Epitopes and Antigenic Differentiation of Australian Strains.” Journal of General Virology 72 (12): 2915–2922. doi:10.1099/0022-1317-72-12-2915.
- Jackwood, M. W. 2012. “Review of Infectious Bronchitis Virus around the World.” Avian Diseases 56 (4): 634–641. doi:10.1637/10227-043012-Review.1.
- Joiner, K. S., F. J. Hoerr, S. J. Ewald, V. L. Van Santen, J. C. Wright, F. W. Van Ginkel, and H. Toro. 2007. “Pathogenesis of Infectious Bronchitis Virus in Vaccinated Chickens of Two Different Major Histocompatibility B Complex Genotypes.” Avian Diseases 51 (3): 758–763. doi:10.1637/0005-2086(2007)51[758:POIBVI]2.0.CO;2.
- Kameka, A. M., S. Haddadi, D. S. Kim, S. C. Cork, and M. F. Abdul-Careem. 2014. “Induction of Innate Immune Response following Infectious Bronchitis Corona Virus Infection in the Respiratory Tract of Chickens.” Virology 450-451: 114–121. doi:10.1016/j.virol.2013.12.001.
- Kint, J., M. A. Langereis, H. J. Maier, P. Britton, F. J. van Kuppeveld, J. Koumans, G. F. Wiegertjes, and M. Forlenza. 2016. “Infectious Bronchitis Coronavirus Limits Interferon Production by Inducing a Host Shutoff that Requires Accessory Protein 5b.” Journal of Virology 90 (16): 7519–7528. doi:10.1128/JVI.00627-16.
- Kuchipudi, S. V., M. Tellabati, R. K. Nelli, B. B. Perez, S. Sebastian, S. P. Dunham, K. C. Chang, et al. 2012. “18S rRNA Is a Reliable Normalisation Gene for Real Time PCR Based on Influenza Virus Infected Cells.” Virology Journal 9 (1). doi:10.1186/1743-422X-9-230.
- Kuchipudi, S. V., M. Tellabati, S. Sebastian, B. Z. Londt, C. Jansen, L. Vervelde, S. M. Brookes, I. H. Brown, S. P. Dunham, and K. Chang. 2014. “Highly Pathogenic Avian Influenza Virus Infection in Chickens but Not Ducks is Associated with Elevated Host Immune and Pro-Inflammatory Responses.” Veterinary Research 45 (1): 1–18. doi:10.1186/s13567-014-0118-3.
- Lay, D. C., Jr., R. M. Fulton, P. Y. Hester, D. M. Karcher, J. B. Kjaer, J. A. Mench, B. A. Mullens, et al. 2011. “Hen Welfare in Different Housing Systems 1.” Poultry Science 90 (1): 278–294. doi:10.3382/ps.2010-00962.
- McMartin, D. A. 1993. “Infectious Bronchitis.” In Virus Infections of Birds, edited by J. B. McFerran and M. S. McNulty, 249–275. Amsterdam: Elsevier Science.
- Mockett, A. P., and J. K. Cook. 1986. “The Detection of Specific IgM to Infectious Bronchitis Virus in Chicken Serum Using an ELISA.” Avian Pathology 15 (3): 437–446. doi:10.1080/03079458608436305.
- Okino, C. H., A. C. Alessi, F. Montassier Mde, A. J. Rosa, X. Wang, and H. J. Montassier. 2013. “Humoral and Cell-mediated Immune Responses to Different Doses of Attenuated Vaccine against Avian Infectious Bronchitis Virus.” Viral Immunology 26 (4): 259–267. doi:10.1089/vim.2013.0015.
- Okino, C. H., I. L. Dos Santos, F. S. Fernando, A. C. Alessi, X. Q. Wang, and H. J. Montassier. 2014. “Inflammatory and Cell-mediated Immune Responses in the Respiratory Tract of Chickens to Infection with Avian Infectious Bronchitis Virus.” Viral Immunology 27 (8): 383–391. doi:10.1089/vim.2014.0054.
- Okino, C. H., M. A. Mores, I. M. Trevisol, A. Coldebella, H. J. Montassier, and L. Brentano. 2017. “Early Immune Responses and Development of Pathogenesis of Avian Infectious Bronchitis Viruses with Different Virulence Profiles.” PLoS One 12 (2): e0172275. doi:10.1371/journal.pone.0172275.
- Oladele, O. A., D. F. Adene, T. U. Obi, and H. O. Nottidge. 2009. “Comparative Susceptibility of Chickens, Turkeys and Ducks to Infectious Bursal Disease Virus Using Immunohistochemistry.” Veterinary Research Communincations 33 (2): 111–121. doi:10.1007/s11259-008-9078-2.
- Orr-Burks, N., S. Gulley, R. A. Gallardo, H. Toro, and F. Van Ginkel. 2014. “Immunoglobulin A as an Early Humoral Responder after Mucosal Avian Coronavirus Vaccination.” Avian Diseases 58 (2): 279–286. doi:10.1637/10740-120313-Reg.1.
- Otsuki, K., M. B. Huggins, and J. K. A. Cook. 1990. “Comparison of the Susceptibility to Avian Infectious Bronchitis Virus Infection of Two Inbred Lines of White Leghorn Chickens.” Avian Pathology 19 (3): 467–475. doi:10.1080/03079459008418700.
- Qu, H., L. Yang, S. Meng, L. Xu, Y. Bi, X. Jia, J. Li, L. Sun, and W. Liu. 2013. “The Differential Antiviral Activities of Chicken Interferon α (ChIFN-α) and ChIFN-β are Related to Distinct Interferon-stimulated Gene Expression.” PLoS One 8 (3): e59307. doi:10.1371/journal.pone.0059307.
- Raggi, L., and G. Lee. 1965. “Lack of Correlation between Infectivity, Serologic Response and Challenge Results in Immunization with an Avian Infectious Bronchitis Vaccine.” The Journal of Immunology 94 (4): 538–543.
- Raj, G. D., and R. C. Jones. 1996. “Local Antibody Production in the Oviduct and Gut of Hens Infected with a Variant Strain of Infectious Bronchitis Virus.” Veterinary Immunology and Immunopathology 53 (1–2): 147–161. doi:10.1016/0165-2427(95)05545-2.
- Raj, G. D., and R. C. Jones. 1997. “Infectious Bronchitis Virus: Immunopathogenesis of Infection in the Chicken.” Avian Pathology 26 (4): 677–706. doi:10.1080/03079459708419246.
- Reed, L. J., and H. Muench. 1938. “A Simple Method of Estimating Fifty per Cent Endpoints.” American Journal of Epidemiology 27 (3): 493–497. doi:10.1093/oxfordjournals.aje.a118408.
- Sharma, B., N. Kakker, S. Bhadouriya, and R. Chhabra. 2020. “Effect of TLR Agonist on Infections Bronchitis Virus Replication and Cytokine Expression in Embryonated Chicken Eggs.” Molecular Immunology 120: 52–60. doi:10.1016/j.molimm.2020.02.001.
- Smith, J., J. R. Sadeyen, D. Cavanagh, P. Kaiser, and D. W. Burt. 2015. “The Early Immune Response to Infection of Chickens with Infectious Bronchitis Virus (IBV) in Susceptible and Resistant Birds.” BMC Veterinary Research 11 (1): 256. doi:10.1186/s12917-015-0575-6.
- Steyn, A., S. Keep, E. Bickerton, and M. Fife. 2020. “The Characterization of chIFITMs in Avian Coronavirus Infection in Vivo, Ex Vivo and in Vitro.” Genes (Basel) 11 (8): 918. doi:10.3390/genes11080918.
- Toro, H., and I. Fernandez. 1994. “Avian Infectious Bronchitis: Specific Lachrymal IgA Level and Resistance against Challenge.” Journal of Veterinary Medicine, Series B 41 (1‐10): 467–472. doi:10.1111/j.1439-0450.1994.tb00252.x.
- Toro, H., H. Hidalgo, and A. N. Collingwood-Selby. 1994. “Lachrymal Antibody Response of Specific Pathogen Free Chickens and Chickens with Maternal Immunity after Infectious Bronchitis Virus Vaccination.” Preventive Veterinary Medicine 18 (4): 267–274. doi:10.1016/0167-5877(94)90051-5.
- van Ginkel, F. W., S. L. Gulley, A. Lammers, F. J. Hoerr, R. Gurjar, and H. Toro. 2012. “Conjunctiva-associated Lymphoid Tissue in Avian Mucosal Immunity.” Developmental and Comparitive Immunology 36 (2): 289–297. doi:10.1016/j.dci.2011.04.012.
- van Ginkel, F. W., V. L. van Santen, S. L. Gulley, and H. Toro. 2008. “Infectious Bronchitis Virus in the Chicken Harderian Gland and Lachrymal Fluid: Viral Load, Infectivity, Immune Cell Responses, and Effects of Viral Immunodeficiency.” Avian Diseases 52 (4): 608–617. doi:10.1637/8349-050908-Reg.1.
- van Santen, V. L., H. Toro, F. W. van Ginkel, K. S. Joiner, and F. J. Hoerr. 2006. “Effects of CAV And/or IBDV on IBV Infection and Immune Responses.” Paper presented at the Proceedings of the V International Symposium on Avian Corona-and Pneumoviruses and Complicating Pathogens, Rauischholzhausen, Germany.
- Wang, X., A. J. M. Rosa, H. N. Oliverira, G. J. M. Rosa, X. Guo, M. Travnicek, and T. Girshick. 2006. “Transcriptome of Local Innate and Adaptive Immunity during Early Phase of Infectious Bronchitis Viral Infection.” Viral Immunology 19 (4): 768–774. doi:10.1089/vim.2006.0051.
- Wu, B., A. Peisley, C. Richards, H. Yao, X. Zeng, C. Lin, F. Chu, T. Walz, and S. Hur. 2013. “Structural Basis for dsRNA Recognition, Filament Formation, and Antiviral Signal Activation by MDA5.” Cell 152 (1–2): 276–289. doi:10.1016/j.cell.2012.11.048.
- Yang, X., J. Li, H. Liu, P. Zhang, D. Chen, S. Men, X. Li, and H. Wang. 2018. “Induction of Innate Immune Response following Introduction of Infectious Bronchitis Virus (IBV) in the Trachea and Renal Tissues of Chickens.” Microbial Pathogenesis 116: 54–61. doi:10.1016/j.micpath.2018.01.008.
- Yu, L. P., X. R. Zhang, T. Q. Wu, J. Su, Y. Y. Wang, Y. X. Wang, B. Y. Ruan, X. S. Niu, and Y. T. Wu. 2017. “Avian Infectious Bronchitis Virus Disrupts the Melanoma Differentiation Associated Gene 5 (MDA5) Signaling Pathway by Cleavage of the Adaptor Protein MAVS.” BMC Veterinary Research 13 (1). doi:10.1186/s12917-017-1253-7.
- Zegpi, R. A., K. S. Joiner, V. L. van Santen, and H. Toro. 2020a. “Infectious Bronchitis Virus Population Structure Defines Immune Response and Protection.” Avian Diseases 64 (1): 60–68. doi:10.1637/0005-2086-64.1.60.
- Zegpi, R. A., C. Breedlove, S. Gulley, and H. Toro. 2020b. “Infectious Bronchitis Virus Immune Responses in the Harderian Gland upon Initial Vaccination.” Avian Diseases 64 (1): 92–95. doi:10.1637/0005-2086-64.1.92.
- Zhang, T., L. Deyin, Z. Jia, J. Chang, and X. Hou. 2017. “Cellular Immune Response in Chickens Infected with Avian Infectious Bronchitis Virus (IBV).” European Journal of Inflammation 15 (1): 35–41. doi:10.1177/1721727x17703886.
- Zhao, X., J. Li, C. A. Winkler, P. An, and J. T. Guo. 2018. “IFITM Genes, Variants, and Their Roles in the Control and Pathogenesis of Viral Infections.” Frontiers in Microbiology 9: 3228. doi:10.3389/fmicb.2018.03228.