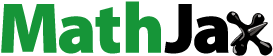
ABSTRACT
1. This study investigated the relationships of quality indices with the severity of wooden breast (WB) myopathy in chicken breast meat under refrigerated storage. The physicochemical properties, water-holding capacity (WHC), microbial quality and fatty acid profiles of normal chicken breast meat samples (NOR samples, n = 63), moderate WB (MWB, n = 63) myopathy and severe WB (SWB, n = 63) myopathy (MWB and SWB samples, respectively) were evaluated immediately after sampling and after 4 and 8 d of refrigerated storage at 4°C.
2. Total collagen, fat, saturated and monounsaturated fatty acid contents, redness and pH of the SWB and MWB samples were higher than the NOR samples. The SWB samples that were stored for 8 d had poor WHC, total viable counts (TVC) of higher than 7.0log colony-forming units, total volatile basic nitrogen (TVB-N) content of greater than 15 mg/100 g and a thiobarbituric acid – reactive substance level of higher than 1 mg/kg malondialdehyde.
3. No significant difference was observed in the TVB-N content and TVC of the MWB and NOR samples during storage. Polyunsaturated fatty acid content was lower in the SWB and MWB samples than in the NOR samples. The SWB samples were tougher than the MWB and NOR samples after 8 d of refrigeration.
4. In conclusion, the quality of chicken breast meat with SWB myopathy degraded considerably over time; thus, such meat should not be subjected to extended refrigeration for storage.
Introduction
The demand for chicken meat has increased considerably because of its health benefits, lower cost relative to red meat and amenability to further processing. Specifically, chicken meat has low fat and high protein contents and a balanced n-6/n-3 polyunsaturated fatty acid (PUFA) ratio (Soglia et al. Citation2017). To satisfy the growing demand for meat, breeders have begun breeding chickens with high growth rates and breast yields; however, these are more likely to exhibit muscle abnormalities, such as wooden breast (WB) myopathy (Petracci et al. Citation2019). This problem, which has been recognised as an emerging quality defect in broiler meat, is characterised macroscopically by the occurrence of pale, ridge-like bulges which are hard at the caudal end, as well as viscous exudate and haemorrhages (Sihvo, Immonen, and Puolanne Citation2014; Xing et al. Citation2020). Quality defects in meat samples with WB myopathy, such as decreased protein content and water-holding capacity (WHC), increased hardness and lipid and an unattractive appearance, result in commercial loss for poultry producers (K. Wang, Li, and Sun Citation2023). The incidence of WB myopathy has been reported worldwide. Dalle Zotte et al. (Citation2017) observed that 53.2% of broiler carcases exhibited WB myopathy at a processing facility in Italy and Xing et al. (Citation2020) reported that 30.8% of the commercial broiler population in China exhibited moderate (MWB) or severe WB (SWB) myopathy. The fresh chicken meat sold in markets is commonly stored in refrigerators at temperatures between 0°C and 7°C (Rocha et al. Citation2022).
Numerous studies have revealed that chicken meat with WB myopathy undergoes changes during refrigerated storage (Hasegawa et al. Citation2020; Rocha et al. Citation2022; Soglia et al. Citation2017, Citation2018; Sun et al. Citation2018; Tasoniero et al. Citation2017; Zhu et al. Citation2023). Byron et al. (Citation2020) claimed that the severity of WB myopathy exhibited by chicken meat samples can be reduced through the refrigerated storage of these samples for a few days. Moreover, Soglia et al. (Citation2018) and Sun et al. (Citation2018) observed that chicken meat with WB myopathy softens over cold storage, probably because of myofibril proteolysis. Similarly, De Oliveira et al. (Citation2021a) suggested that ageing might be a commercially viable alternative technique for improving the quality of meat with WB myopathy.
Fresh poultry meat is perishable and has a comparatively short shelf life (Chmiel et al. Citation2019). The high content of unsaturated fatty acids in chicken meat can deteriorate its sensory quality and shorten its shelf life because of oxidation (Chmiel et al. Citation2019). Moreover, chicken muscles that are affected by WB myopathy are particularly sensitive to oxidative reactions (Petracci et al. Citation2019). Soglia et al. (Citation2018) and Xing et al. (Citation2021) observed that chicken muscles affected by WB myopathy exhibited increased lipid oxidation and Pan et al. (Citation2021) and Xing et al. (Citation2021) showed increased protein oxidation in chicken meat with WB myopathy. Furthermore, changes in the muscle of chickens with WB myopathy cause modifications in the muscle fibre structures and microbial activity of the meat, which affects spoilage susceptibility (Dalgaard et al. Citation2018). Studies have revealed that severe WB myopathy markedly affects the quality of meat. For example, reducing the proportions of fibrotic and necrotic muscle cells increases meat hardness and reduces WHC (Dalgaard et al. Citation2018; Tijare et al. Citation2016; Xing et al. Citation2020). This phenomenon is a major concern in the poultry industry, because severe WB myopathy may reduce the quality and shelf life of poultry meat. Furthermore, the abundance of lipid is associated with oxidation reactions in WB, although few studies have focused on fatty acid profiles in WB (Cauble et al. Citation2020; Gratta et al. Citation2019). Although the quality of meat samples with various levels of WB severity changes during refrigerated storage (Byron et al. Citation2020; de Oliveira et al. Citation2021a; Sun et al. Citation2018), the understanding of the physicochemical properties and fatty acid profiles of these meat samples during refrigerated storage remains limited. Bridging this knowledge gap is crucial as the quality of fresh meat undergoes considerable changes within a limited shelf life. Therefore, the present study was conducted to investigate how the severity of WB myopathy affects the quality and oxidation of chicken breast meat during refrigerated storage.
Materials and methods
Sample preparation
Skinless and boneless breast fillet samples from the same flock of broiler chickens (Arbor Acres; age, 35 d of age, average body weight at slaughter approximately 2.2 kg), which were reared under an evaporative cooling system, fed commercial feed and harvested under standard commercial conditions, were analysed approximately at 3 h post-mortem in a commercial poultry slaughterhouse (Nantou, Taiwan). Through visual and tactile inspections, based on the criteria proposed by Sihvo et al. (Citation2014) and Tijare et al. (Citation2016), three trained panellists assessed the samples for the level of hardness, size of the hard area and colour of whole breast fillet surface. Briefly, NOR samples (n = 63) were flexible throughout and did not exhibit any sign of WB myopathy. The MWB samples (n = 63) were harder than NOR and specifically exhibited diffuse hardened areas at the cranial end as well as a slight ridge-like bulge at the caudal end. The SWB samples (n = 63) exhibited extensive rigidity throughout the meat with a ridge-like bulge from cranial region to caudal end and their surface was covered with viscous exudate along with petechiae, as shown in .
Figure 1. Breast fillets displaying different degrees of wooden breast (WB) severity. NOR: normal muscle; MWB: moderate wooden breast; SWB: severe wooden breast. In the WB fillets, the petechiae and outbulging areas are indicated by the arrows and circles, respectively. *Surface covered with exudate and hemorrhages.
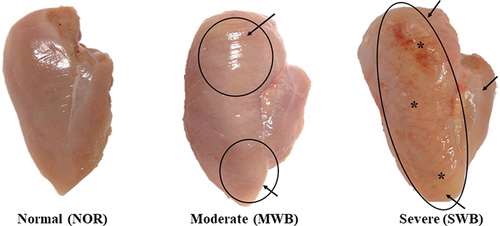
All collected fillet samples were transported in insulated boxes with ice packs to the laboratory within 30 min. The superficial fat, cartilage and connective tissues of the fillets were cut off and the prepares samples weighed and individually packaged in 0.908-L Ziploc bags. Samples from each category (NOR, MWB and SWB) were randomly assigned to the following three groups and then analysed. Samples were either analysed immediately (d 0), after refrigeration at 4°C for 4 d or refrigerated at 4°C for 8 d.
Physicochemical properties and TVC
The pH of the samples was determined at four locations in the cranial and caudal regions by using a meat pH metre (HI99163, Hanna Instruments, Inc., Woonsocket, RI), which was calibrated prior to use. The surface colours of the samples were determined in terms of L* (lightness), a* (redness) and b* (yellowness) values by using a colourimeter (NR-3000, Nippon denshoku, Japan) at three cranial, medial and caudal locations on the ventral side. Subsequently, proximate analysis was conducted to determine the moisture, protein and fat contents of the samples, in accordance with relevant AOAC (Citation1995) methods. The myofibril fragmentation index (MFI) was determined in accordance with the method described by De Oliveira et al. (Citation2021a). A 3 g minced sample was homogenised in 30 ml of cold extraction buffer (100 mM KCl, 20 mM Potassium phosphate (pH 7), 1 mM EGTA, 1 MgCl2, 1 mM NaN3) for 1.5 min and the homogenate was centrifuged at 14,400 ×g, at 4°C for 5 min (Heraeus Megafuge 16, Thermo Scientific, UK;). After the supernatant was discarded, the precipitate was dispersed in 30 ml of extraction buffer, stirred with a glass rod and centrifuged again. This operation was repeated twice. After the supernatant was discarded, 15 ml of extraction buffer was added to the precipitate and the suspension was then filtered through a polyethylene strainer to remove connective tissues. The biuret method was used to determine the protein concentration of the myofibril suspension. An aliquot from the suspension of myofibrils was diluted with extraction buffer to a protein concentration of 0.5 ± 0.05 mg/ml. The diluted suspension of myofibrils was stirred and its absorbance was then measured at 540 nm by using a spectrophotometer (U–2900, Hitachi, Japan). The MFI was calculated as follows:
The content of thiobarbituric acid – reactive substance (TBARS) was determined according to the method of De Oliveira et al. (Citation2021a). A 5 g minced sample was homogenised (Wiggins D-500, Straubenhardt, Germany) in 25 ml of 7.5% (w/v) trichloroacetic acid solution (25°C) at 10,000 rpm for 2 min and then vortexed for 30 min. After filtration (Whatman No. 1 paper) and the addition of 2.5 ml of 0.02 M 2-thiobarbituric acid to the supernatant solution, the mixture was boiled in a water bath at 100°C for 40 min. After the supernatant was cooled to ambient temperature for 30 min and centrifuged at 5,500 rpm (Mikro 220 R, Hettich, Germany) for 25 min, its absorbance was measured at 532 nm by using the spectrophotometer (U–2900, Hitachi, Japan) and the TBARS content was determined.
The total volatile basic nitrogen (TVB-N) content was examined using the method of Sujiwo et al. (Citation2018). A 10 g sample was mixed with 90 ml of distilled water, homogenised and then centrifuged at 800 × g (Heraeus Megafuge 16, Thermo Scientific, UK) for 10 min. The supernatant was filtered and 1 ml of 0.01 N boric acid and 50 μl of indicator (methyl red: bromocresol green = 1:1) were added to the inner section of a Conway microdiffusion cell and 1 ml of 50% potassium carbonate and 1 ml of the filtrate were added to the outer section of this cell. The cell was sealed, incubated at 37°C for 2 h and then titrated with 0.02 N sulphuric acid. The TVB-N content was calculated in ammonia equivalents as follows:
where A was the titration volume of the sample (ml), B the titration volume of the blank (ml), F the standardisation index of 0.02 N sulphuric acid and S the sample weight (g).
The total viable count (TVC) was determined using the method of Sujiwo et al. (Citation2018). A 10 g sample was homogenised in 90 ml of sterile NaCl (0.9%) and the TVC of the sample was determined using plate count agar after the sample was incubated at 37°C for 48 h. The TVC was reported as the logarithm of colony-forming units (CFUs) per gram of meat sample.
The total collagen and insoluble collagen contents were determined using the methods of Zhu et al. (Citation2023). A 2.5 g minced sample was transferred into a digestion tube containing 30 ml of 6 M sulphuric acid and the flask was covered with a watch glass. The sample was hydrolysed in a digester (Tecator Digestion System 20-1015, Tecator, Inc., Herndon, VA, U.S.A.) at 110°C for 16 h. A total of 100 ml of Milli-Q water was then used to dilute the hydrolysed sample and the diluted sample was subsequently filtered (Whatman No. 1 filter paper) and neutralised with 6 M NaOH. Then 2 ml aliquots of the sample were transferred in duplicate to test tubes, to which 1 ml of colour reagent (10 g of p-dimethylaminobenzaldehyde in 35 ml of 60% perchloric acid and 65 ml of isopropanol) and 1 ml of oxidation reagent (chloramine-T 1.41%) were added. The mixed samples were incubated at 60°C for 30 min, cooled and then allowed to stand for 25 min. Subsequently, their absorbance was measured at a wavelength (λ) of 560 nm by using the spectrophotometer (U–2900, Hitachi, Japan). The hydroxyproline content was determined against a standard calibration curve prepared in a similar manner to that described in the aforementioned text. Total collagen content was determined from the hydroxyproline content by using a conversion factor of 7.25 and expressed as a percentage. For the measurement of the insoluble collagen content, minced muscle samples were maintained in a water bath at 77°C for 65 min, the homogenates were then centrifuged at 5,800 rpm (Mikro 220 R, Hettich, Germany) for 10 min and the precipitates were considered the insoluble part. This was analysed using the same procedure as used for measuring total collagen content. The average values of six measurements of total and insoluble collagen contents were recorded for each sample.
Fatty acid profiles were determined using chloroform and methanol extraction, as described by Kaewkot et al. (Citation2022). The fatty acids were transformed to methyl esters and analysed using a gas chromatography – flame ionisation detector equipped with an RT-2560 capillary column with helium as the carrier gas. The initial oven temperature was set as 170°C for 40 min and the temperature was then increased at a rate of 3°C/min to 200°C; the temperature was then maintained at 200°C for 50 min. The temperatures of the injector and detector were 250°C and 300°C, respectively. The flow rate at the outlet terminal was maintained at 0.75 ml/min. The peak areas were identified by referring to the retention time of standards with known fatty acid contents (Food Industry Fame Mix; RESTEX, Bellefonte, PA, U.S.A.).
WHC and shear force
The fillets were weighed before packaging. After refrigerated storage, the fillets were patted dry by using tissue paper and then reweighed. The purge loss from the samples was determined by calculating their percentage weight loss during storage. At d 0 and after refrigerated storage for 4 and 8 d, the fillets were weighed and hung individually inside a polyethylene bag. After hanging for 24 h, the samples were weighed again and their drip loss was calculated using the following equation:
The samples were placed individually in a plastic bag and then cooked in an 80°C water bath (Shaking bath B603D; Firstek, Taichung, Taiwan) until their core temperature reached 75°C. Then, the samples were cooled to ambient temperature and patted dry with tissue paper and cooking loss was determined using the following equation:
Shear force was determined using a slightly modified version of the method of Kaewkot et al. (Citation2023). Rectangular samples (10 mm × 10 mm × 20 mm) were cut from the anteroventral cranial area of raw samples. Then, the shear force (N) required to cut the sample was determined using a CT3 texture analyser (Brookfield Engineering labs Inc., U.S.A.) equipped with a probe (TA 25/1000, 1 mm/s).
Microstructure analysis
The microstructures of the samples from the cranial part of the Pectoralis major muscles were prepared using formalin fixation, ethanol, dehydration and paraffin embedding, according to the methods of Soglia, Mudalal, et al. (Citation2016). Samples embedded with paraffin were cut into 6–8 μm slices (RM2245; Leica, Germany) and transferred onto gelatine-coated glass slides. The samples were deparaffinised using xylene, stained with Ehrlich’s haematoxylin and then covered with a drop of neutral balsam. The sample tissues were fixed on the slides and photographed using a microscope (BX43, Olympus, Japan) at 200× magnification.
Statistical analyses
The effects of meat type (NOR, MWB and SWB) and refrigeration duration (0, 4 or 8 d) on quality and any interactions were determined using the model:
where Mi was the meat type effect i = 1 to 3; Dj refrigeration duration effect j = 1 to 3 and Mi × Dj the meat type by refrigeration duration interaction effect.
The results were analysed using the GLM procedure and means were determined and the pdiff option was used to separate significant effects and interactions. Differences were considered significant when p < 0.05. All statistical analyses were conducted by using SAS software (Version 9.4 SAS Institute, Cary, NC, United States).
Results and discussion
pH, colour, oxidation and microbial quality
According to the results presented in , pH was affected by severity of WB myopathy and refrigeration duration; however, no interaction (p > 0.05) was observed between the severity of WB myopathy and refrigeration duration. The SWB and MWB samples had higher pH (6.11 and 6.08, respectively) than the NOR samples (5.80) on d 0 (p = 0.0061). This finding agreed with those of previous studies (Baldi et al. Citation2019; Byron et al. Citation2020; Dalle Zotte et al. Citation2017). De Oliveira et al. (Citation2021a) suggested that that meat with WB myopathy had higher pH because this problem leads to decreased glycolytic potentials and changes in metabolic pathways. In the present study, the pH of the meat increased after 4 d of refrigerated storage (p < 0.0001), regardless of the severity of WB and more probably because of the accumulation of metabolites produced by psychrotrophic bacteria, such as amines and ammonia (Cortez‐Vega, Pizato, and Prentice Citation2012). Refrigerated storage from 4 to 8 d resulted in a decrease in the pH, probably because of the accumulation of metabolites produced by lactic acid bacteria, which are responsible for discoloration, off-flavours, acid production, slime formation and meat spoilage (Lulietto et al. Citation2015). Moreover, the decline in the pH of meat with and without WB myopathy during refrigerated storage may influence the susceptibility of the protein in the meat to carbonylation (Rocha et al. Citation2022).
Table 1. Changes in pH, colour, myofibrillar fragmentation (MFI), thiobarbituric acid reactive substance (TBARS), total volatile basic nitrogen (TVB-N) and total viable count (TVC) of chicken breast with different severity of wooden breast myopathy during refrigerated storage.
The SWB samples had higher L*, a* and b* values than did the NOR samples (p< 0.05). Baldi et al. (Citation2019) demonstrated that compared with meat without WB myopathy, meat with WB myopathy has higher muscle lightness and yellowness values because of the tissue modifications that occur in it following histological degeneration and its higher fat content. De Oliveira et al. (Citation2021a) reported that certain changes, such as an increase in the moisture content on muscular surfaces because of a decrease in WHC as well as severe fibrosis caused by muscular tissue degeneration, lead to increases in the L* and b* values of muscles with SWB myopathy. An increased incidence and severity of petechial haemorrhagic lesions might be responsible for the increased haemoglobin content in the muscle exudate of fillets with WB myopathy (Zhuang and Bowker Citation2018). In the present study, the L*, a* and b* values of the samples increased with the refrigeration duration (p ˂ 0.05); this result is in agreement with that of Byron et al. (Citation2020). Vytejckova et al. (Citation2017) observed that chicken breast meat exhibited dramatic increases in a* and b* values after 5-day refrigerated storage. An interaction between the severity of WB myopathy and the duration of refrigeration was observed for b* values (p = 0.0004). The increase in the b* value during refrigerated storage was likely affected by the oxidation of oxygenised myoglobin and deoxymyoglobin in the presence of oxygen, leading to the formation of brown metmyoglobin (Luo et al. Citation2021). Rocha et al. (Citation2022) indicated that the increase in the b* value of WB meat over an extended refrigerated storage period was a result of frequent lipid and protein oxidation reactions. shows that the MFI increased during refrigerated storage, which is an indicator of protein degradation during meat ageing, processing and storage (Gerelt, Ikeuchi, and Suzuki Citation2000; p < 0.0001). The SWB samples exhibited lower MFI values than the MWB and NOR samples (p < 0.0001), which was in agreement with the report of De Oliveira et al. (Citation2021a).
The TVB-N value should be less than 15 mg/100 g for meat to be considered fresh (Sujiwo, Kim, and Jang Citation2018) and a bacterial count higher than 7 log CFU/g is commonly considered spoilt (ICMSF Citation2000). In meat, TBARS over 0.5 mg of malondialdehyde (MDA)/kg indicates the occurrence of some oxidation and, when above 1.0 mg MDA/kg, this is considered unacceptable (Reitznerova et al. Citation2017). In the present study (), after refrigerated storage for 4 d, TBARS increased and the highest content was seen for the SWB samples (0.68 mg MDA/kg), followed by the MWB and NOR samples (0.45 and 0.41 mg MDA/kg, respectively). The TVC and TVB-N values after 4 d storage for the SWB samples (6.03 log CFU/g and 15.55 mg/100 g, respectively) were higher than the MWB (5.52 log CFU/g and 11.75 mg/100 g, respectively) and NOR samples (5.41 log CFU/g and 11.61 mg/100 g, respectively). Among all the samples, the SWB samples that were refrigerated for 8 d had the highest TVC, TBARS and TVB-N values (7.60 log CFU/g, 1.07 mg MDA/kg and 18.84 mg/100 g, respectively; p < 0.0001). The TVC and TVB-N values from the MWB and NOR samples did not differ significantly during storage. However, the SWB samples exhibited significantly higher TBARS and TVB-N values during refrigerated storage than the MWB and NOR samples (p < 0.0001). Interactions between the severity of WB myopathy and the duration of refrigeration were observed for both TBARS and TVB-N values (p = 0.004 and p = 0.001, respectively). The presence of severe WB myopathy considerably increased the frequencies of lipid and protein oxidation reactions, particularly during extended refrigerated storage (Rocha et al. Citation2022). During the extended refrigeration of meat with WB myopathy, particularly SWB myopathy, the exudate on the meat might provide nutrients for microbial growth, which results in the accumulation of TVB-N (Xing et al. Citation2020). Dalgaard et al. (Citation2018) indicated that in addition to tissue composition, moisture content, drip loss, and mobile water fraction influenced the growth and composition of endogenous microflora in samples with WB myopathy. Rocha et al. (Citation2022) and De Oliveira et al. (Citation2021b) found that the TBARS values of samples with WB myopathy increased significantly after refrigerated storage. Fillets with WB myopathy exhibit higher oxidation than do normal fillets, which might be related to the higher lipid contents of fillets with WB myopathy (i.e., 2.13%, 1.95%, and 1.53% for the SWB, MWB, and NOR samples in the current study, respectively) and the higher oxidative instability of their myopathy-affected muscles compared with those of normal fillets (Estévez Citation2015; Rocha et al. Citation2022). Villegas-Cayllahua et al. (Citation2023) indicated that meat with WB myopathy is more susceptible to oxidation than is normal meat; thus, meat with WB myopathy has less nutritional value, probably because of its higher fat level and rancidity. Overall, the results of the current study indicated that the SWB samples were unsuitable for 8-day refrigerated storage.
Proximate composition, collagen content, WHC, shear force and microstructure analysis
The SWB and MWB samples had higher moisture and fat and lower protein content than the NOR samples (). A notable interaction between the severity of WB myopathy and the duration of refrigeration was observed for protein content (p = 0.044). De Oliveira et al. (Citation2021a) reported a considerable decrease in the content of soluble proteins in WB exudate after 7 d refrigerated storage compared with normal meat. This decrease may contribute to a reduction in protein in the WB samples. The SWB samples had higher fat content than the NOR samples (2.13% vs. 1.53%, p < 0.0001) but this did not change significantly during storage (p = 0.227). Soglia, Laghi, et al. (Citation2016), Soglia, Mudalal, et al. (Citation2016) and Rocha et al. (Citation2022) explained that meat with WB myopathy has a higher moisture content because of the presence of oedema due to inflammation. Moreover, the pooling of water with proteins, which assists in the repair of areas affected by myopathy, results in a higher moisture in meat with WB myopathy than in normal meat (Byron et al. Citation2020). Lipidosis, which refers to an abnormal accretion of lipids as a result of tissue injury, contributes to the elevated lipid content of muscles with WB myopathy (Carvalho et al. Citation2020; Radaelli et al. Citation2017). shows that, among the three types of samples, the SWB samples had the highest total and insoluble collagen content, followed by the MWB and NOR samples (p < 0.0001), which was not affected by refrigeration duration. These results agreed with the findings of previous studies (Soglia, Laghi, et al. Citation2016; Soglia, Mudalal, et al. Citation2016; Soglia et al. Citation2017; Petracci et al. Citation2019; Zhu, Puolanne, and Ertbjerg Citation2023). De Oliveira et al. (Citation2021b) reported higher total collagen in meat with MWB and SWB myopathy than in normal meat, as did Mudalal et al. (Citation2015) and Geronimo et al. (Citation2022) for meat samples with any WB myopathy. Using Azan staining of paraffin sections and scanning electron microscopy, Hasegawa et al. (Citation2020) demonstrated that the intramuscular connective tissue in meat with WB myopathy was thicker than that in normal meat. The purge, drip and cooking losses have been determined to assess the WHC of the samples (Byron et al. Citation2020; de Oliveira et al. Citation2021a; Xing et al. Citation2020), and SWB and MWB samples exhibited higher losses than the NOR samples on d 0 (p < 0.0001; ). The purge and drip loss increased during storage for all samples with there was no interaction between the severity of WB myopathy and refrigeration duration. Byron et al. (Citation2020) found that their SWB samples had higher purge loss than the NOR samples. Sun et al. (Citation2018) observed that drip loss increased with the severity of WB myopathy. Moreover, Zhu et al. (Citation2023) reported that purge loss from WB samples was significantly higher than for NOR samples. The aforementioned results were in agreement with Mudalal et al. (Citation2015) and the present study. Hasegawa et al. (Citation2020) observed that, at d 7 post-mortem, samples with WB myopathy exhibited higher calpain activities and higher degradations of the muscle protein molecules desmin and nebulin than NOR samples. Byron et al. (Citation2020) reported that the purge loss from meat increased over storage time and fillets with SWB myopathy exhibited higher purge loss than NOR fillets, possibly because they had lower protein, higher moisture and greater protein degradation, specifically greater z-line and desmin degradation. Tasoniero et al. (Citation2017) evaluated the influence of storage time on nuclear magnetic resonance relaxation and reported that the T21 population (i.e., water trapped in the myofibrillar matrix, which represents immobilised water) was generally higher in normal samples than in those with WB myopathy. This water population remained stable during storage for the NOR samples, whereas the T21 population was higher in samples with WB myopathy that were refrigerated for 72 h than in those refrigerated for 10 h. The presence of excess muscle exudate, which mainly contains a blend of water-soluble nucleotides, amino acids, peptides, proteins and vitamins, in raw meat samples reduces product yield and causes undesirable appearance and textures, which result in economic losses and quality deterioration (Xing et al. Citation2020).
Table 2. Changes in proximate composition, total and insoluble collagen, water-holding capacity and shear force of chicken breast with different severity of wooden breast myopathy during refrigerated storage.
In the present study, the cooking losses of all samples increased after 4 d of storage and then decreased on d 8 of storage and there was an interaction between the two factors (p < 0.0001; ). This result might have been caused by an increase in the purge loss over storage time (Byron et al. Citation2020). Previous studies showed that, irrespective of the sample conditions, i.e., whether it was fresh (Soglia, Mudalal, et al. Citation2016), previously frozen (Tijare et al. Citation2016) or marinated (Dalle Zotte et al. Citation2017), all the normal fillets had less cooking loss than those with WB myopathy. Pang et al. (Citation2020) suggested that the denaturation of collagens and myofibrillar proteins might affect the moisture loss of meat with WB myopathy during cooking. They observed higher cooking loss for WB meat at higher cooking temperatures (>57°C), which may have been caused by the denaturation of actin and connective tissue and the subsequent increased loss of intramyofibrillar and extramyofibrillar water. Through fluorescent microscopy, Velleman and Clark (Citation2015) and Byron et al. (Citation2020) found that meat with WB myopathy lacked muscle fibre bundle organisation and well-defined spacing in the endomysium and perimysium. They observed a low prevalence of extracellular matrix glycosaminoglycans, which are highly negatively charged and covalently bind to myofibrillar proteins, which participate in ionic interactions with water. The limited prevalence of extracellular matrix glycosaminoglycans resulted in the meat with WB myopathy having high cooking losses and low WHC. The reduction in the level of WHC of WB meat may, hence, be attributable to the degeneration of muscle fibres and myofibrils (Gratta et al. Citation2019; Soglia, Laghi, et al. Citation2016). The WB meat exhibited higher degrees of weight loss and higher amounts of exudate during refrigerated storage than the normal meat (de Oliveira et al. Citation2021a).
Among all the samples, the SWB meat, which was characterised by its hardness, exhibited the highest shear force (p < 0.0001; ). Hasegawa et al. (Citation2020) indicated that mechanical strength of meat is affected by components derived from myofibrils and intramuscular connective tissues. The content of collagen and the thickness of the perimysium are the main factors determining toughness in meat (Hasegawa et al. Citation2021). The thickening of connective tissues between muscle fibres results in an increase in the mechanical strength of muscles exhibiting WB myopathy (Hasegawa et al. Citation2020, Citation2021). Soglia et al. (Citation2018) stated that meat ageing, caused by proteolytic processes, affects the myofibrillar protein fractions during storage. In the present study, the shear force of the SWB samples refrigerated for 8 d was approximately 27% lower than that of the fresh SWB samples. However, for all three types of samples, no significant difference was observed in the shear force between the samples stored for 4 or 8 d. This was in agreement with Gratta et al. (Citation2019), who reported that shear force value of NOR and WB chicken breasts was not significantly different after storage for 5 d. Hasegawa et al. (Citation2020) observed that meat with WB myopathy had considerably higher mechanical strength than NOR meat on d 5 post-mortem because a considerable portion of the intramuscular connective tissues in the meat with WB myopathy barely changed.
Focal or diffuse hardening was observed macroscopically in the affected breast muscles of the MWB and SWB samples (). The SWB samples exhibited more obvious out-bulging and pale areas with petechial haemorrhages and viscous exudate than the other samples.
The histological observations displayed in indicated that the NOR samples exhibited relatively homogeneous normal-coloured and polygonal muscle fibres. However, the SWB and MWB samples exhibited a wider extracellular matrix, a higher degree of myopathy proliferation in connective tissues, degenerating muscle fibres with a larger size discrepancy and higher numbers of adipose tissues and hypercontracted fibres. The histological abundance of intermuscular connective tissues in the SWB meat might have contributed to the high shear force of these samples.
Figure 2. Histomicrograph of breast muscles affected by wooden breast (WB). Features of the muscle include degenerating muscle fibers (Dm), adipose tissue (Ap), hypertrophy of myofiber (Hc), and increased connective tissue (Ct) and extracellular matrix (*). HE, 200x.
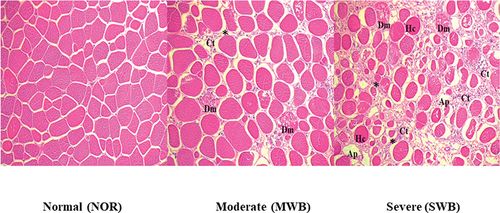
Sihvo et al. (Citation2014) investigated WB myopathy through histological analysis and found that these muscles showed active degeneration and regeneration of fibres and contained immune cells with high adipose deposition and more connective tissue. Hosotani et al. (Citation2020) examined the pathological features of muscles with SWB myopathy and suggested that, in addition to the accumulation of connective tissues, myofiber hypertrophy, breast muscle degeneration and reduced regeneration might be induced in broiler chickens by the accumulation of damaged mitochondria.
Fatty acid profiles
The results presented in indicated that, compared with the NOR samples, the MWB and SWB samples had more saturated fatty acid (SFA; 30.03% vs. 30.14% and 30.24%, respectively), particularly palmitic acid (C16:0) and stearic acid (C18:0) (p < 0.0001). Compared with the NOR samples, the SWB and MWB samples had more monounsaturated fatty acids (MUFA; 36.20 vs. 36.44% and 36.46%, respectively), particularly palmitoleic acid (C16:1) and oleic acid (C18:1n9) (p < 0.0001). Conversely, PUFA in the SWB and MWB samples (33.32% and 33.43%, respectively) were lower than that in the NOR samples (33.71%; p < 0.0001). Particularly significant differences were observed for the following omega-3 and omega-6 fatty acids: linoleic acid (C18:2n6), eicosadienoic acid (C20:2n6), arachidonic acid (C20:4n6) and docosahexaenoic acid (C22:6n3). Levels of C18:3n3 and C18:3n6 were significantly higher in the SWB samples than in the NOR samples. During storage, the percentages of C16:0, C18:0 and C18:1n9c increased in the NOR, MWB and SWB samples, with the SWB samples had the highest percentages of SFA and MUFA but the lowest percentages of PUFA (i.e., C18:2n6, C20:4n6 and C22:6n3) after storage for 8 d. Interactions between the severity of WB myopathy and the duration of refrigeration were evident, particularly for palmitoleic and oleic acids (p = 0.003 and p < 0.0001, respectively).
Table 3. Changes in fatty acid composition (% total fatty acid) of chicken breast with different severity of wooden breast myopathy during refrigerated storage.
Palmitoleic acid, a lipokine, prevents lipogenesis and proinflammatory macrophage polarisation (Sansone et al. Citation2016). Oleic acid, which is recognised for its health benefits, prevents oxidation, inflammation and microbial growth (Bhattacharjee et al. Citation2020). Furthermore, interactions between the severity of WB myopathy and the duration of refrigeration were observed for MUFA, PUFA and omega-6 fatty acids (p < 0.0001). The reduced levels of PUFA in the WB samples might have resulted from higher lipid peroxidation and the impairment of oxidation due to long-term storage. These findings aligned with those of Liu et al. (Citation2022), who reported increased levels of MUFA and reduced PUFA in WB-affected chicken breast samples. The reductions in PUFA led to relative increases in MUFA levels (Kaewkot, Hung, and Tan Citation2022).
Omega-3 and omega-6 fatty acids are essential nutrients and antioxidants for broilers. J. Wang et al. (Citation2020) indicated that supplementation with omega-3 fatty acids in the early post-hatch stage can reduce the severity of WB, thereby preserving the quality of meat during storage. Gratta et al. (Citation2019) stated that the degeneration and regeneration of muscle fibres in meat with WB myopathy might contribute to changes in the muscle fibre metabolism and, thus, fatty acid composition of the meat. Similarly, Cauble et al. (Citation2020) found that meat with WB myopathy had higher percentages of SFA (14:0, 15:0 and 17:0) and MUFA (14:1, 16:1c, 17:1t, 18:1c9 and 18:1c11) and lower percentages of PUFA (C18:2n6, C20:2n6, C20:4n6, C20:5n3 and C22:6n3) than normal meat.
Soglia, Mudalal, et al. (Citation2016) indicated that a high level of Ca2+ in meat with WB myopathy might promote degenerative processes in this meat through the activation of phospholipase A2, which is a lipolytic enzyme that affects the phospholipid membrane and contributes to the release of unsaturated fatty acids. The increase in the percentages of SFA with storage time may be related to changes in the PUFA contents, which are expected to decrease during storage because PUFA are more susceptible to oxidation than are SFA and MUFA (Kaewkot, Hung, and Tan Citation2022).
Conclusions
The existence of WB myopathy compromises the physicochemical properties of meat over an extended refrigerated storage period. This condition can adversely alter the surface colour of meat samples, reduce the content of nutrients and increase the frequencies of lipid and protein oxidation reactions. The fluctuating cooking loss observed in the SWB samples refrigerated for either 4 or 8 d indicated that extended storage exacerbates water loss in WB samples. In conclusion, the quality of SWB meat degraded considerably with increasing refrigeration duration. Consequently, chicken meat samples with SWB myopathy should not be subjected to extended refrigerated storage.
Acknowledgments
The assistant from the Ministry of Agriculture, National Animal Industry Foundation, Charoen Pokphand Enterprise (Taiwan) Co., LTD., Mr. Chen-Lung Shiao, Mr. Qu-Nan Zheng, Wen-Hsuan Lin, and Hsing-Yuan Chiu were grateful. Special thanks to Prof. San-Yuan Huang, Prof. Pin-Chi Tang, and Prof. Jiunn-Wang Liao for their kindly support.
Disclosure statement
No potential conflict of interest was reported by the author(s).
References
- AOAC. 1995. Official Methods of Analysis. 15th ed. Verginia, USA: Association of Official Analytical Chemists.
- Baldi, G., F. Soglia, L. Laghi, S. Tappi, P. Rocculi, S. Tavaniello, D. Prioriello, R. Mucci, G. Maiorano, and M. Petracci. 2019. “Comparison of Quality Traits Among Breast Meat Affected by Current Muscle Abnormalities.” Food Research International 115:369–376. https://doi.org/10.1016/j.foodres.2018.11.020.
- Bhattacharjee, B., P. K. Pal, A. Chattopadhyay, and D. Bandyopadhyay. 2020. “Oleic Acid Protects Against Cadmium Induced Cardiac and Hepatic Tissue Injury in Male Wistar Rats: A Mechanistic Study.” Life Sciences 244:117324. https://doi.org/10.1016/j.lfs.2020.117324.
- Byron, M., X. Zhang, M. von Staden, T. Jarvis, C. Crist, W. Zhai, and M. W. Schilling. 2020. “Impact of Refrigerated Storage Time on Woody Broiler Breast Severity and Instrumental Quality.” Meat and Muscle Biology 4 (1): 1–12. https://doi.org/10.22175/mmb.9477.
- Carvalho, L. M., M. S. Madruga, M. Estévez, A. T. Badaró, and D. F. Barbin. 2020. “Occurrence of Wooden Breast and White Striping in Brazilian Slaughtering Plants and Use of Near‐Infrared Spectroscopy and Multivariate Analysis to Identify Affected Chicken Breasts.” Journal of Food Science 85 (10): 3102–3112. https://doi.org/10.1111/1750-3841.15465.
- Cauble, R. N., E. S. Greene, S. Orlowski, C. Walk, M. Bedford, J. Apple, M. T. Kidd, and S. Dridi. 2020. “Dietary Phytase Reduces Broiler Woody Breast Severity via Potential Modulation of Breast Muscle Fatty Acid Profiles.” Poultry Science 99 (8): 4009–4015. https://doi.org/10.1016/j.psj.2020.05.005.
- Chmiel, M., M. Roszko, L. Adamczak, T. Florowski, and D. Pietrzak. 2019. “Influence of Storage and Packaging Method on Chicken Breast Meat Chemical Composition and Fat Oxidation.” Poultry Science 98 (6): 2697–2690. https://doi.org/10.3382/ps/pez029.
- Cortez‐vega, W. R., S. Pizato, and C. Prentice. 2012. “Quality of Raw Chicken Breast Stored at 5C and Packaged Under Different Modified Atmospheres.” Journal of Food Safety 32 (3): 360–368. https://doi.org/10.1111/j.1745-4565.2012.00388.x.
- Dalgaard, L. B., M. K. Rasmussen, H. C. Bertram, J. A. Jensen, H. S. Moller, M. D. Aaslyng, E. K. Hejbol, J. R. Pedersen, D. Elsser-gravesen, and J. F. Young. 2018. “Classification of Wooden Breast Myopathy in Chicken Pectoralis Major by a Standardised Method and Association with Conventional Quality Assessments.” International Journal of Food Science & Technology 53 (7): 1744–1752. https://doi.org/10.1111/ijfs.13759.
- Dalle Zotte, A., G. Tasoniero, E. Puolanne, H. Remignon, M. Cecchinato, E. Catelli, and M. Cullere. 2017. “Effect of “Wooden Breast” Appearance on Poultry Meat Quality, Histological Traits and Lesions Characterization.” Czech Journal of Animal Science 62 (2): 51–57. https://doi.org/10.17221/54/2016-CJAS.
- de Oliveira, R. F., J. L. M. de Mello, F. B. Ferrari, R. A. de Souza, M. R. Pereira, E. N. F. Cavalcanti, E. A. Villegas-cayllahua, et al. 2021a. “Effect of Aging on the Quality of Breast Meat from Broilers Affected by Wooden Breast Myopathy.” Animals 11 (7): 1960. https://doi.org/10.3390/ani11071960.
- de Oliveira, R. F., J. L. M. DE Mello, F. B. Ferrari, E. N. F. Cavalcanti, R. A. D. Souza, M. R. Pereira, A. Giampietro-ganeco, et al. 2021b. “Physical, Chemical and Histological Characterization of Pectoralis Major Muscle of Broilers Affected by Wooden Breast Myopathy.” Animals 11 (3): 596. https://doi.org/10.3390/ani11030596.
- Estévez, M. 2015. “Oxidative Damage to Poultry: From Farm to Fork.” Poultry Science 94 (6): 1368–1378. https://doi.org/10.3382/ps/pev094.
- Gerelt, B., Y. Ikeuchi, and A. Suzuki. 2000. “Meat Tenderization by Proteolytic Enzymes After Osmotic Dehydration.” Meat Science 56 (3): 311–318. https://doi.org/10.1016/S0309-1740(00)00060-7.
- Geronimo, B. C., S. H. Prudencio, and A. L. Soares. 2022. “Biochemical and Technological Characteristics of Wooden Breast Chicken Fillets and Their Consumer Acceptance.” Journal of Food Science and Technology 59 (3): 1185–1192. https://doi.org/10.1007/s13197-021-05123-3.
- Gratta, F., L. Fasolato, M. Birolo, C. Zomeño, E. Novelli, M. Petracci, A. Pascual, and G. Xiccato. 2019. “Effect of Breast Myopathies on Quality and Microbial Shelf Life of Broiler Meat.” Poultry Science 98 (6): 2641–2651. https://doi.org/10.3382/ps/pez001.
- Hasegawa, Y., T. Hara, T. Kawasaki, M. Yamada, T. Watanabe, and T. Iwasaki. 2020. “Effect of Wooden Breast on Postmortem Changes in Chicken Meat.” Food Chemistry 315:126285. https://doi.org/10.1016/j.foodchem.2020.126285.
- Hasegawa, Y., T. Kawasaki, N. Maeda, M. Yamada, N. Takahashi, T. Watanabe, and T. Iwasaki. 2021. “Accumulation of Lipofuscin in Broiler Chicken with Wooden Breast.” Animal Science Journal 92 (1): e13517. https://doi.org/10.1111/asj.13517.
- Hosotani, M., T. Kawasaki, Y. Hasegawa, Y. Wakasa, M. Hoshino, N. Takahashi, T. Watanabe, T. Takaya, T. Iwasaki, and T. Watanabe. 2020. “Physiological and Pathological Mitochondrial Clearance is Related to Pectoralis Major Muscle Pathogenesis in Broilers with Wooden Breast Syndrome.” Frontiers in Physiology 11:579. https://doi.org/10.3389/fphys.2020.00579.
- ICMSF. 2000. Sampling for Microbiological Analysis: Principles and Scientific Applications. 2nd ed. Toronto, Canada: University of Toronto Press.
- Kaewkot, C., W. L. Cheng, and F. J. Tan. 2023. “Physicochemical Properties and Structural Changes of Chicken Breast Meat Subjected to Radio Frequency Tempering Combined with Conventional Thawing Treatments.” Animal Science Journal 94 (1): e13836. https://doi.org/10.1111/asj.13836.
- Kaewkot, C., Y. H. Hung, and F. J. Tan. 2022. “Effect of Freeze–Thaw Cycles on the Physicochemical Properties, Water-Holding Status, and Nutritional Values of Broiler Chicken Drumstick.” Animal Science Journal 93 (1): e13742. https://doi.org/10.1111/asj.13742.
- Liu, R., F. Kong, S. Xing, Z. He, L. Bai, J. Sun, X. Tan, D. Zhao, G. Zhao, and J. Wen. 2022. “Dominant Changes in the Breast Muscle Lipid Profiles of Broiler Chickens with Wooden Breast Syndrome Revealed by Lipidomics Analyses.” Journal of Animal Science and Biotechnology 13 (1): 1–11. https://doi.org/10.1186/s40104-022-00743-x.
- Lulietto, M. F., P. Sechi, E. Borgogni, and B. T. Cenci-goga. 2015. “Meat Spoilage: A Critical Review of a Neglected Alteration Due to Ropy Slime Producing Bacteria.” Italian Journal of Animal Science 14 (3): 4011. https://doi.org/10.4081/ijas.2015.4011.
- Luo, X., K. Dong, L. Liu, F. An, D. Tang, L. Fu, H. Teng, and Q. Huang. 2021. “Proteins Associated with Quality Deterioration of Prepared Chicken Breast Based on Differential Proteomics During Refrigerated Storage.” Journal of the Science of Food and Agriculture 101 (8): 3489–3499. https://doi.org/10.1002/jsfa.10980.
- Mudalal, S., M. Lorenzi, F. Soglia, C. Cavani, and M. Petracci. 2015. “Implications of White Striping and Wooden Breast Abnormalities on Quality Traits of Raw and Marinated Chicken Meat.” Animal 9 (4): 728–734. https://doi.org/10.1017/S175173111400295X.
- Pan, X., L. Zhang, T. Xing, J. Li, and F. Gao. 2021. “The Impaired Redox Status and Activated Nuclear Factor-Erythroid 2-Related Factor 2/Antioxidant Response Element Pathway in Wooden Breast Myopathy in Broiler Chickens.” Animal Bioscience 34 (4): 652–661. https://doi.org/10.5713/ajas.19.0953.
- Pang, B., B. Bowker, G. Gamble, J. Zhang, Y. Yang, X. Yu, J. X. Sun, and H. Zhuang. 2020. “Muscle Water Properties in Raw Intact Broiler Breast Fillets with the Woody Breast Condition.” Poultry Science 99 (9): 4626–4633. https://doi.org/10.1016/j.psj.2020.05.031.
- Petracci, M., F. Soglia, M. Madruga, L. Carvalho, E. Ida, and M. Estevez. 2019. “Wooden‐Breast, White Striping and Spaghetti Meat: Causes, Consequences and Consumer Perception of Emerging Broiler Meat Abnormalities.” Comprehensive Reviews in Food Science and Food Safety 18 (2): 565–583. https://doi.org/10.1111/1541-4337.12431.
- Radaelli, G., A. Piccirillo, M. Birolo, D. Bertotto, F. Gratta, C. Ballarin, M. Vascellari, G. Xiccato, and A. Trocino. 2017. “Effect of Age on the Occurrence of Muscle Fiber Degeneration Associated with Myopathies in Broiler Chickens Submitted to Feed Restriction.” Poultry Science 96 (2): 309–319. https://doi.org/10.3382/ps/pew270.
- Reitznerova, A., M. Sulekova, J. Nagy, S. Marcincak, B. Semjon, M. Certik, and T. Klempova. 2017. “Lipid Peroxidation Process in Meat and Meat Products: A Comparison Study of Malondialdehyde Determination Between Modified 2-Thiobarbituric Acid Spectrophotometric Method and Reverse-Phase High-Performance Liquid Chromatography.” Molecules 22 (11): 1988. https://doi.org/10.3390/molecules22111988.
- Rocha, T. C. D., D. V. C. Filho, L. M. D. Carvalho, J. M. D. Carvalho, M. Estevez, and M. S. Madruga. 2022. “Effect of Refrigeration and Freezing on the Oxidative Stability of WB Chicken Breast.” LWT-Food Science and Technology 171:114108. https://doi.org/10.1016/j.lwt.2022.114108.
- Sansone, A., E. Tolika, M. Louka, V. Sunda, S. Deplano, M. Melchiorre, D. Anagnostopoulos, et al. 2016. “Hexadecenoic Fatty Acid Isomers in Human Blood Lipids and Their Relevance for the Interpretation of Lipidomic Profiles.” Public Library of Science One 11 (4): e0152378. https://doi.org/10.1371/journal.pone.0152378.
- Sihvo, H. K., K. Immonen, and E. Puolanne. 2014. “Myodegeneration with Fibrosis and Regeneration in the Pectoralis Major Muscle of Broilers.” Veterinary Pathology 51 (3): 619–623. https://doi.org/10.1177/0300985813497488.
- Soglia, F., J. Gao, M. Mazzoni, E. Puolanne, C. Cavani, M. Petracci, and P. Ertbjerg. 2017. “Superficial and Deep Changes of Histology, Texture and Particle Size Distribution in Broiler Wooden Breast Muscle During Refrigerated Storage.” Poultry Science 96 (9): 3465–3472. https://doi.org/10.3382/ps/pex115.
- Soglia, F., L. Laghi, L. Canonico, C. Cavani, and M. Petracci. 2016. “Functional Property Issues in Broiler Breast Meat Related to Emerging Muscle Abnormalities.” Food Research International 89:1071–1076. https://doi.org/10.1016/j.foodres.2016.04.042.
- Soglia, F., S. Mudalal, E. Babini, M. di Nunzio, M. Mazzoni, F. Sirri, C. Cavani, and M. Petracci. 2016. “Histology, Composition and Quality Traits of Chicken Pectoralis Major Muscle Affected by Wooden Breast Abnormality.” Poultry Science 95 (3): 651–659. https://doi.org/10.3382/ps/pev353.
- Soglia, F., Z. Zeng, J. Gao, E. Puolanne, C. Cavani, M. Petracci, and P. Ertbjerg. 2018. “Evolution of Proteolytic Indicators During Storage of Broiler Wooden Breast Meat.” Poultry Science 97 (4): 1448–1455. https://doi.org/10.3382/ps/pex398.
- Sujiwo, J., D. Kim, and A. Jang. 2018. “Relation Among Quality Traits of Chicken Breast Meat During Cold Storage: Correlations Between Freshness Traits and Torrymeter Values.” Poultry Science 97 (8): 2887–2894. https://doi.org/10.3382/ps/pey138.
- Sun, X., D. A. Koltes, C. N. Coon, K. Chen, and C. M. Owens. 2018. “Instrumental Compression Force and Meat Attribute Changes in Woody Broiler Breast Fillets During Short-Term Storage.” Poultry Science 97 (7): 2600–2606. https://doi.org/10.3382/ps/pey107.
- Tasoniero, G., H. C. Bertram, J. F. Young, A. D. Zotte, and E. Puolanne. 2017. “Relationship Between Hardness and Myowater Properties in Wooden Breast Affected Chicken Meat: A Nuclear Magnetic Resonance Study.” LWT-Food Science and Technology 86:20–24. https://doi.org/10.1016/j.lwt.2017.07.032.
- Tijare, V. V., F. L. Yang, V. A. Kuttappan, C. Z. Alvarado, C. N. Coon, and C. M. Owens. 2016. “Meat Quality of Broiler Breast Fillets with White Striping and Woody Breast Muscle Myopathies.” Poultry Science 95 (9): 2167–2173. https://doi.org/10.3382/ps/pew129.
- Velleman, S. G., and D. L. Clark. 2015. “Histopathologic and Myogenic Gene Expression Changes Associated with Wooden Breast in Broiler Breast Muscles.” Avian Diseases 59 (3): 410–418. https://doi.org/10.1637/11097-042015-Reg.1.
- Villegas-cayllahua, E. A., J. L. M. D. Mello, D. R. Dutra, R. F. D. Oliveira, E. F. Cavalcanti, M. R. Pereira, F. B. Ferrari, et al. 2023. “Effect of Freezing on the Quality of Breast Meat from Broilers Affected by Wooden Breast Myopathy.” Poultry Science 102 (8): 102702. https://doi.org/10.1016/j.psj.2023.102702.
- Vytejckova, S., L. Vapenka, J. Hradecky, J. Dobias, J. Hajslova, C. Loriot, L. Vannini, and J. Poustka. 2017. “Testing of Polybutylene Succinate Based Films for Poultry Meat Packaging.” Polymer Testing 60:357–364. https://doi.org/10.1016/j.polymertesting.2017.04.018.
- Wang, J., D. L. Clark, S. K. Jacobi, and S. G. Velleman. 2020. “Effect of Vitamin E and Omega-3 Fatty Acids Early Posthatch Supplementation on Reducing the Severity of Wooden Breast Myopathy in Broilers.” Poultry Science 99 (4): 2108–2119. https://doi.org/10.1016/j.psj.2019.12.033.
- Wang, K., Y. Li, and J. Sun. 2023. “Quality Improvement and Comprehensive Utilization of Abnormal Broiler Breast Meat: A Review.” Food Materials Research 3 (1): 1–13. https://doi.org/10.48130/FMR-2023-0001.
- Xing, T., X. Pan, L. Zhang, and F. Gao. 2021. “Hepatic Oxidative Stress, Apoptosis and Inflammation in Broiler Chickens with Wooden Breast Myopathy.” Frontiers in Physiology 12:659777. https://doi.org/10.3389/fphys.2021.659777.
- Xing, T., X. Zhao, X. Xu, J. Li, L. Zhang, and F. Gao. 2020. “Physiochemical Properties, Protein and Metabolite Profiles of Muscle Exudate of Chicken Meat Affected by Wooden Breast Myopathy.” Food Chemistry 316:126271. https://doi.org/10.1016/j.foodchem.2020.126271.
- Zhu, X., E. Puolanne, and P. Ertbjerg. 2023. “Changes of Raw Texture, Intramuscular Connective Tissue Properties and Collagen Profiles in Broiler Wooden Breast During Early Storage.” Foods 12 (7): 1530. https://doi.org/10.3390/foods12071530.
- Zhuang, H., and B. Bowker. 2018. “The Wooden Breast Condition Results in Surface Discoloration of Cooked Broiler Pectoralis Major.” Poultry Science 97 (12): 4458–4461. https://doi.org/10.3382/ps/pey284.