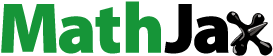
ABSTRACT
1. An experiment was conducted to determine the effect of the source of fat (soybean oil or tallow) on the ileal endogenous amino acid (EAA) losses in broilers.
2. Three nitrogen (N)-free diets; a control diet with no added fat and test diets with 60 g/kg of either soybean oil or tallow were formulated. Titanium dioxide (5 g/kg) was added to all diets as an indigestible marker. Each diet was assigned to six replicate cages (eight birds per cage) from d 18 to 21 post-hatch. On d 21, the digesta were collected from the lower half of the ileum.
3. The endogenous losses of nitrogen and amino acids (AA) were lower (p = 0.08; p = 0.001) in broilers fed diets with soybean oil or tallow, respectively, compared to those fed the diet with no fat. Source of fat had no influence (p > 0.05) on EAA losses.
4. The most abundant AA in the ileal endogenous protein was glutamic acid, followed by aspartic acid, threonine, leucine, serine, valine and proline. In general, the concentrations of AA in the endogenous protein were lower (p < 0.05) with added fat. The exceptions were methionine, cysteine, proline and serine, which were unaffected. The effect of fat source on the AA contents of endogenous protein were inconsistent and differed depending on the AA.
5. The inclusion of fats decreased EAA losses which implied they have beneficial effects beyond direct energy contribution. It can be proposed that the reduction of EAA flow may be an additional mechanism contributing to the extra-caloric effect of dietary fats.
Introduction
The term endogenous amino acid (EAA) losses refers to those in the digesta leaving the terminal ileum, which are not of food origin. The measurement of EAA losses is needed to correct the apparent amino acid (AA) digestibility to true values (Lemme, Ravindran, and Bryden Citation2004; Ravindran and Bryden Citation1999). These losses represent a significant metabolic cost to the animal (Cowieson et al. Citation2009) and a better understanding of influencing factors is of fundamental interest to improve the nitrogen (N) utilisation efficiency of animals. A number of dietary factors have already been identified, including methodology, the amount and type of dietary protein and fibre, and presence of anti-nutritional factors (Adedokun et al. Citation2011; Ravindran Citation2021).
Animal fats and vegetable oils are energy dense, providing a unique and cost-effective opportunity to increase the energy supply in broiler diets. Typical fat inclusions in broiler diets range from 30 to 60 g/kg and even a small change in levels can have meaningful effects on energy supply. These two fat sources differ in their physical and chemical properties, and their digestion dynamics are inherently different. Unsaturated fatty acids dominate vegetable oils, whereas animal fats are more heavily composed of saturated fatty acids (Blanch et al. Citation1995). Saturated fatty acids are non-polar and so cannot form mixed micelles spontaneously and need bile salts for emulsification to form micelles prior to hydrolysis. However, unsaturated fatty acids in soybean oil are readily emulsified and, hence, better digested and absorbed (Ravindran et al. Citation2016).
A protein-sparing effect of fat in pig diets has been observed, which was presumed to be the result of slowing the rate of passage. Imbeah and Sauer (Citation1991) and Cervantes-Pahm and Stein (Citation2008) reported improvements (up to 4.9%) in the ileal digestibility of some AA when canola oil was added to diets for growing pigs. Li and Sauer (Citation1994) found that AA ileal digestibility was linearly increased when canola oil inclusion was raised from 32 to 122 g/kg. Cowieson et al. (Citation2010) similarly observed that addition of 20 g/kg soybean oil to a broiler starter diets increased ileal AA digestibility by an average of 3.5%. The greatest increase was seen in the digestibility of threonine, serine, isoleucine, aspartic acid, valine and glycine; the predominant AA in endogenous protein. These observations implied improvements in AA digestibility with added fat and it can be speculated that the mechanisms involved may, in part, reflect reductions in EAA losses. In addition, the composition and digestion kinetics of vegetable oils and animal fats differ (Tancharoenrat et al. Citation2014), and may be relevant on their influence on endogenous AA secretions and losses.
The present study tested the hypotheses that the addition of fat can lower ileal EAA losses in broilers and that, owing to differences in emulsification, digestion and absorption, the degree of reduction can be influenced by fat source.
Materials and methods
Ethical statement
The experimental procedures were approved by the Massey University ethics committee
Animal husbandry
Day-old male broilers (Ross 308) were raised in the floor until d 14 post-hatch and fed a commercial broiler starter diet (12.9 MJ/kg metabolisable energy, 226 g/kg crude protein, 11.0 g/kg Ca, 6.7 g/kg total P). On d 14, birds were transferred to grower cages and were maintained on the same diet until the introduction of test diets on d 18 post-hatch. Both the floor facility and cages were in an environmentally controlled house. Each cage was equipped with a linear feeder in the front and a nipple drinker with a cup in the back. The temperature was maintained at 31°C on d 1 and reduced to 20°C by d 21. A lighting schedule of 20 hours per day was applied by fluorescent tubes.
Three N-free diets, namely with no added fat source, 60 g/kg soybean oil or 60 g/kg tallow (), were developed. Titanium dioxide (5.0 g/kg) was included in the diets as an indigestible marker. On d 18, birds were individually weighed, and 144 birds were assigned to 18 cages containing eight birds each. Following 4 h of feed withdrawal, the assay diets were introduced and offered ad libitum. Water was available at all times.
Table 1. Ingredient composition (g/kg as fed) of the experimental diets.
On d 21, all birds were euthanised by intravenous injection of sodium pentobarbitone and the contents of the lower ileum were collected. The ileum was divided into two halves and the contents were collected from the lower half towards the ileo-caecal junction (Ravindran and Bryden Citation1999). Digesta were flushed out with reverse-osmosis water, pooled within each cage, mixed, sub-sampled and lyophilised. Samples of diets and digesta were ground to pass through 0.5 mm sieve and stored in airtight plastic containers at 4°C until chemical analyses.
Chemical analyses
Fatty acid composition of the two fat sources was determined following the procedure of Sukhija and Palmquist (Citation1988). The diet and digesta samples were analysed for dry matter, titanium dioxide, nitrogen (N) and AA, including sulphur-containing AA. Dry matter was determined by drying samples at 105°C for 16 h in a pre-weighed dried crucible in a convection oven (AOAC International Citation2016 method no: 930.15). Nitrogen was determined by using an N determinator (model FP-428, LECO® Corporation, St Joseph, MI). Titanium was determined as per the procedures of Short et al. (Citation1996). For AA analysis, samples were prepared by hydrolysing the samples with HCl (containing phenol) for 24 h at 110 ± 2°C in glass tubes sealed under vacuum. Amino acids were then detected on a Waters ion exchange HPLC system, and the chromatograms were integrated using dedicated software (Maxima 820, Waters, Millipore, Milford, MA) with the AA identified and quantified using a standard AA solution (Pierce, Rockford, IL). Cysteine and methionine were analysed as cysteic acid and methionine sulphone by oxidation with performic acid for 16 h at 0°C and neutralisation with hydrobromic acid prior to hydrolysis.
The EAA flow at the terminal ileum was calculated as mg lost per kg dry matter intake (DMI) using the following formula (Moughan et al. Citation1990).
The AA concentrations were also calculated as percentage of crude protein (N x 6.25) and presented as g/100 g crude protein.
Statistical analysis
The data were analysed using the General Linear Model procedure (SAS Citation2015). Differences were considered significant at p < 0.05 and significant differences between means were separated by the Least Significant Difference test. Cage served as the experimental unit.
Results
The analysed fatty acid composition of soybean oil and tallow are presented in . The major fatty acids in both fat sources were palmitic (16:0), stearic (18:0), oleic (18:1) and linoleic (18:2) acids. Unsaturated fatty acids (18:1 and 18:2) were higher in soybean oil and saturated fatty acids (16:0 and 18:0) content were higher in tallow. The ratio of unsaturated to saturated fatty acids in soybean oil and tallow were 5.66 and 1.17, respectively.
Table 2. Fatty acid composition of soybean oil and tallow (g/kg fat, as received)1.
The influence of dietary treatments on ileal EAA losses is summarised in . Addition of fat lowered the endogenous flows of N and all AA (p = 0.08 to p = 0.001, respectively). The reductions in ileal endogenous N losses due to the addition of soybean oil and tallow were 38.0 and 47.8%, respectively. The corresponding reductions in total EAA losses were 47.1 and 55.9%, respectively. The influence of the source of fat on EAA losses were similar (p > 0.05).
Table 3. Influence of fat type on the ileal endogenous nitrogen and amino acid flows (g/kg dry matter intake) in broilers1.
The AA profile of endogenous protein (g/100 g crude protein), as influenced by fat addition, is shown in . In all three treatments, the most abundant AA in the ileal endogenous protein was glutamic acid, followed by aspartic acid, threonine, leucine, serine, proline and glycine. In general, the concentrations of AA in the endogenous protein were lowered (p < 0.05) by added fat. The exceptions were methionine, cysteine, proline and serine, which were unaffected. The effect of fat source on individual AA contents of the endogenous protein, however, were inconsistent and differed depending on each AA.
Table 4. Influence of fat type on the composition of endogenous protein (g/100 g crude protein)1.
Discussion
When N-containing diets are fed, the amount of nitrogenous materials leaving the ileum represents the net balance between N intake and secretions minus the absorption of dietary N and re-absorption of endogenous N (Moughan Citation2003). However, there is no N ingestion when a N-free diet is fed and that found in the digesta at terminal ileum is the arithmetic difference between the secretion and re-absorption of N of endogenous origin. Thus, the reduction in EAA losses with fat addition can only be explained by lower endogenous secretions, enhanced re-absorption, or both. Of these possibilities, lower secretion of endogenous N due to fat addition is counter-intuitive, whereas increased re-absorption provides a more likely explanation. Among the possible reasons for increased re-absorption, two are worth considering. First, the well-established deceleration in digesta transit time through the intestine with fat inclusion that results in digesta having a prolonged contact time with enzymes and absorptive sites. Mateos and Sell (Citation1981) used this argument to account for the ‘extra-metabolic effect’ of dietary fats. It has been suggested that fats inhibit gastric emptying and slow the digesta passage rate (Mateos, Sell, and Eastwood Citation1982). It is possible that, through this mechanism, fats improve the utilisation of non-fat nutrients, including AA. Second, there may have been intestinal morphological changes due to added fat. Goda and Takase (Citation1994) found that a high-fat diet increased the crypt depth and, length and proliferation of microvilli in the intestine of rats. A study by Li et al. (Citation1990) similarly revealed that pigs fed a combination of soybean and coconut oils had longer villi and increased crypt depth when compared to those fed diets with no fat. An increase in the absorptive area could increase the efficiency of absorption, allowing better re-absorption of endogenous proteins in broilers fed diets with added fat.
Changes in the microbiome with added fat may be another possible cause. Microbial mass contributes substantially to the N content in the ileal digesta (Miner-Williams, Moughan, and Fuller Citation2009), but are not considered part of the endogenous N fraction. The caeca is the primary area of bacterial activity in poultry, but the small intestine has a significant presence of bacterial population (Bindari and Gerber Citation2022). The intestinal bacteria are particularly associated with mucosa (Tomas et al. Citation2016). Some bacterial species colonise the intestinal wall and use mucin a source of energy and AA (Dai et al. Citation2015; Pan and Yu Citation2014). They disrupt mucus production and the lower the release of digestive enzymes (Pan and Yu Citation2014; Tomas et al. Citation2016). It has been suggested that dietary fats may indirectly influence intestinal microbiome through its impact on intestinal transit time (Knarreborg et al. Citation2002). In the current study, however, changes in intestinal microbiome with fat addition were not measured.
The present results supported the test hypothesis in that the inclusion of dietary fat decreased the endogenous losses. However, no differences were observed between EAA losses in broilers fed diets with tallow and soybean oil, which suggested that, despite possible differences in digesta transit time, fat type had no effect on the EAA losses in broilers. These results further suggested that presence of fats can increase true AA digestibility. Studies in pigs have indicated that fat composition may be relevant (D. F. Li et al. Citation1990; Merriman et al. Citation2016). Li et al. (Citation1990) found that improvements in AA digestibility with added fat sources were higher in those with unsaturated fatty acids.
The concentrations of EAA in the ileal digesta were within the range reported in the literature (Adedokun et al. Citation2011; Ravindran Citation2021). The higher proportions of glutamic acid, aspartic acid, threonine, leucine, serine, valine and proline determined in the endogenous protein were as expected. These seven AA accounted for 69–70% of the total in endogenous protein. The high proportions of glutamic acid, aspartic acid, threonine, serine and leucine and valine in the endogenous protein may have been due to their dominance in mucins and digestive enzymes, and the slower rate of re-absorption compared with other AA. The lower concentrations of Met and His can be attributed to the fact that these AA were absorbed in greatest proportions compared to the others (Ravindran Citation2021).
Endogenous proteins originate from various digestive secretions (bile, pancreatic enzymes and, gastric and intestinal secretions), mucoproteins and desquamated intestinal epithelial cells. It is known that these individual sources differ in their AA composition (Cowieson et al. Citation2009; Ravindran Citation2021; Tancharoenrat, Zaefarian, and Ravindran Citation2022). The current findings suggested that the profile of the EAA flow was altered by dietary fats, ostensibly due to changes in the relative contribution of its components. In general, the concentrations of AA in the endogenous protein were lowered by added fat. The exceptions were methionine, cysteine, proline and serine, which were unaffected. The effect of fat source on AA content in endogenous protein, however, were not consistent and differed depending on the AA. This inconsistency reflected the complexity of ascertaining the changes in the contribution of individual endogenous components.
A unique phenomenon associated with dietary fats is their ‘extra-caloric’ effect, wherein AME values are sometimes determined to be greater than the GE in the fat. This effect has been attributed to the decelerating effect of fats on digesta passage rate (Mateos and Sell Citation1981), giving more time for the digestion and absorption of other energy-yielding nutrients. The present work provided evidence for an additional mechanism, by which fat addition improved metabolisable energy through reductions in EAA losses. As discussed by Cowieson et al. (Citation2009), the loss of EAA is nutritionally expensive because the synthesis and secretion of digestive enzymes and mucoproteins constitute a net energy cost to the animal.
In the current study, fat sources replaced (w/w) dextrose in the experimental diets resulting in increased AME contents (). This approach may be questioned because of the possibility that dietary energy content may influence endogenous AA flows. To the authors’ knowledge, however, no published reports are available on the effects of AME on endogenous secretions or protein losses in monogastric animals. The only alternative design would have been to balance the AME content by adjustments in starch or protein, but this would have confounded the stated aim.
Conclusions
To the authors’ knowledge, this is the first study examining the relationship between dietary fat and ileal EAA losses in birds. The hypotheses tested in the current research were that fat inclusion will lower the EAA losses and that the effects will be more pronounced with soybean oil. The former thesis was supported by the present results, but not the latter. Overall, the findings indicated that that the presence of fat in broiler diets lowers EAA flow, implying that dietary fats have a beneficial effects on N utilisation efficiency.
Disclosure statement
No potential conflict of interest was reported by the author(s).
References
- Adedokun, S. A., O. Adeola, C. M. Parsons, M. S. Lilburn, and T. J. Applegate. 2011. “Factors Affecting Endogenous Amino Acid Flow in Chickens and the Need for Consistency in Methodology.” Poultry Science 90 (8): 1737–1748. https://doi.org/10.3382/ps.2010-01245.
- Aoac International. 2016. Official Methods of Analysis. 20th ed. Washington, DC: Association of Official Analytical Chemists.
- Bindari, Y. R., and P. F. Gerber. 2022. “Centennial Review: Factors Affecting the Chicken Gastrointestinal Microbial Composition and Their Association with Gut Health and Productive Performance.” Poultry Science 101 (1): 101612. https://doi.org/10.1016/j.psj.2021.101612.
- Blanch, A., A. C. Barroeta, M. D. Baucells, and F. Puchal. 1995. “The Nutritive Value of Dietary Fats in Relation to their Chemical Composition, Apparent Fat Availability and Metabolizable Energy in Two-Week-Old Chicks.” Poultry Science 74 (8): 1335–1340. https://doi.org/10.3382/ps.0741335.
- Cervantes-pahm, S. K., and H. H. Stein. 2008. “Effect of Dietary Soybean Oil and Soybean Protein Concentration on the Concentration of Digestible Amino Acids in Soybean Products Fed to Growing Pigs.” Journal of Animal Science 86 (8): 1841–1849. https://doi.org/10.2527/jas.2007-0721.
- Cowieson, A. J., M. R. Bedford, and V. Ravindran. 2010. “Interactions Between Xylanase and Glucanase in Corn-Soy Based Diets for Broilers.” British Poultry Science 51 (2): 246–257. https://doi.org/10.1080/00071661003789347.
- Cowieson, A. J., M. R. Bedford, P. H. Selle, and V. Ravindran. 2009. “Phytate and Microbial Phytase: Implications for Endogenous Nitrogen Losses and Nutrient Availability.” World’s Poultry Science Journal 65:401–418. https://doi.org/10.1017/S0043933909000294.
- Dai, Z., Z. Wu, S. Hang, W. Zhu, and G. Wu. 2015. “Amino Acid Metabolism in Intestinal Bacteria and its Potential Implications for Mammalian Reproduction.” Molecular Human Reproduction 21 (5): 389–409. https://doi.org/10.1093/molehr/gav003.
- Goda, T., and S. Takase. 1994. “Effect of Dietary Fat Content on Microvillus in Rat Jejunum.” Journal of Nutritional Science and Vitaminology 40 (2): 127–136. https://doi.org/10.3177/jnsv.40.127.
- Imbeah, M., and W. C. Sauer. 1991. “The Effect of Dietary Level of Fat on Amino Acid Digestibilities in Soybean Meal and Canola Meal and on Rate of Passage in Growing Pigs.” Livestock Production Science 29 (2–3): 227–239. https://doi.org/10.1016/0301-6226(91)90068-2.
- Knarreborg, A., M. A. Simon, R. M. Engberg, B. B. Jensen, and G. W. Tannock. 2002. “Effects of Dietary Fat Source and Subtherapeutic Levels of Antibiotic on the Bacterial Community in the Ileum of Broiler Chickens at Various Ages.” Applied and Environmental Microbiology 68 (12): 5918–5924. https://doi.org/10.1128/AEM.68.12.5918-5924.2002.
- Lemme, A., V. Ravindran, and W. L. Bryden. 2004. “Ileal Digestibility of Amino Acids in Feed Ingredients for Broilers.” World’s Poultry Science Journal 60:421–435. https://doi.org/10.1079/WPS200426.
- Li, S., and W. C. Sauer. 1994. “The Effect of Dietary-Fat Content on Amino-Acid Digestibility in Young-Pigs.” Journal of Animal Science 72 (7): 1737–1743. https://doi.org/10.2527/1994.7271737x.
- Li, D. F., R. C. Thaler, J. L. Nelssen, D. L. Harmon, G. L. Allee, and T. L. Weeden. 1990. “Effect of Fat Sources and Combinations on Starter Pig Performance, Nutrient Digestibility and Intestinal Morphology.” Journal of Animal Science 68 (11): 3694–3704. https://doi.org/10.2527/1990.68113694x.
- Mateos, G. G., and J. L. Sell. 1981. “Nature of Extra-Metabolic Effect of Supplemental Fat Used in Semi-Purified Diets for Laying Hens.” Poultry Science 60 (8): 1925–1930. https://doi.org/10.3382/ps.0601925.
- Mateos, G. G., J. L. Sell, and J. A. Eastwood. 1982. “Rate of Food Passage (Transit Time) as Influenced by Level of Supplemental Fat.” Poultry Science 61 (1): 94–100. https://doi.org/10.3382/ps.0610094.
- Merriman, L. A., C. L. Walk, C. M. Parsons, and H. H. Stein. 2016. “Effects of Tallow, Choice White Grease, Palm Oil, Corn Oil, or Soybean Oil on Apparent Total Tract Digestibility of Minerals in Diets Fed to Growing Pigs.” Journal of Animal Science 94 (10): 4231–4238. https://doi.org/10.2527/jas.2016-0682.
- Miner-williams, W., P. J. Moughan, and M. F. Fuller. 2009. “Endogenous Components of Digesta Protein from the Terminal Ileum of Pigs Fed a Casein-Based Diet.” Journal of Agricultural and Food Chemistry 57 (5): 2072–2078. https://doi.org/10.1021/jf8023886.
- Moughan, P. J. 2003. “Amino Acid Availability: Aspects of Chemical Analysis and Bioassay.” Nutrition Research Reviews 16 (2): 127–141. https://doi.org/10.1079/NRR200365.
- Moughan, P. J., A. J. Darragh, W. C. Smith, and C. A. Butts. 1990. “Perchloric and Trichloroacetic Acids as Precipitant of Protein in Endogenous Ileal Digesta from the Rat.” Journal of the Science of Food and Agriculture 52:13–21. https://doi.org/10.1002/jsfa.2740520103.
- Pan, D., and Z. Yu. 2014. “Intestinal Microbiome of Poultry andiIts Interaction with Host and Diet.” Gut Microbes 5 (1): 108–119. https://doi.org/10.4161/gmic.26945.
- Ravindran, V. 2021. “Progress in Ileal Endogenous Amino Acid Flow Research in Poultry.” Journal of Animal Science and Biotechnology 12 (1): 5. https://doi.org/10.1186/s40104-020-00526-2.
- Ravindran, V., and W. L. Bryden. 1999. “Amino Acid Availability in Poultry—In Vitro and In Vivo Measurements.” Australian Journal of Agricultural Research 50 (5): 889–908. https://doi.org/10.1071/AR98174.
- Ravindran, V., P. Tancharoenrat, F. Zaefarian, and G. Ravindran. 2016. “Fats in Poultry Nutrition: Digestive Physiology and Factors Influencing Their Utilisation.” Animal Feed Science and Technology 213:1–21. https://doi.org/10.1016/j.anifeedsci.2016.01.012.
- SAS. 2015. Statistical Analysis System, Version 9.4. Cary, NC: SAS Institute Inc.
- Short, F. J., P. Gorton, J. Wiseman, and K. N. Boorman. 1996. “Determination of Titanium Dioxide Added as an Inert Marker in Chicken Digestibility Studies.” Animal Feed Science and Technology 59 (4): 215–221. https://doi.org/10.1016/0377-8401(95)00916-7.
- Sukhija, P. S., and D. L. Palmquist. 1988. “Rapid Method for Determination of Total Fatty Acid Content and Composition of Feedstuffs and Feces.” Journal of Agricultural and Food Chemistry 36 (6): 1202–1206. https://doi.org/10.1021/jf00084a019.
- Tancharoenrat, P., V. Ravindran, F. Zaefarian, and G. Ravindran. 2014. “Digestion of Fat and Fatty Acids Along the Gastrointestinal Tract of Broiler Chickens.” Poultry Science 93 (2): 412–419. https://doi.org/10.3382/ps.2013-03344.
- Tancharoenrat, P., F. Zaefarian, and V. Ravindran. 2022. “Composition of Chicken Gallbladder Bile.” British Poultry Science 63 (4): 548–551. https://doi.org/10.1080/00071668.2022.2044451.
- Tomas, J., C. Mulet, A. Saffarian, J. B. Cavin, R. Ducroc, B. Regnault, C. Tan, et al. 2016. “High-Fat Diet Modifies the PPAR-Γ Pathway Leading to Disruption of Microbial and Physiological Ecosystem in Murine Small Intestine.” Proceedings of the National Academy of Sciences of the United States of America, 113:E5934–E5943. https://doi.org/10.1073/pnas.1612559113.