ABSTRACT
1. The poultry microbiome and its stability at every point in time, either free range or reared under different farming systems, is affected by several environmental and innate factors. The interaction of the poultry birds with their microbiome, as well as several inherent and extraneous factors contribute to the microbiome dynamics. A poor understanding of this could worsen poultry heath and result in disease/metabolic disorders.
2. Many diseased states associated with poultry have been linked to dysbiosis state, where the microbiome experiences some perturbation. Dysbiosis itself is too often downplayed; however, it is considered a disease which could lead to more serious conditions in poultry. The management of interconnected factors by conventional and emerging technologies (sequencing, nanotechnology, robotics, 3D mini-guts) could prove to be indispensable in ensuring poultry health and welfare.
3. Findings showed that high-throughput technological advancements enhanced scientific insights into emerging trends surrounding the poultry gut microbiome and ecosystem, the dysbiotic condition, and the dynamic roles of intrinsic and exogenous factors in determining poultry health. Yet, a combination of conventional, -omics based and other techniques further enhance characterisation of key poultry microbiome actors, their mechanisms of action, and roles in maintaining gut homoeostasis and health, in a bid to avert metabolic disorders and infections.
4. In conclusion, there is an important interplay of innate, environmental, abiotic and biotic factors impacting on poultry gut microbiome homoeostasis, dysbiosis, and overall health. Associated infections and metabolic disorders can result from the interconnected nature of these factors. Emerging concepts (interkingdom or network signalling and neurotransmitter), and future technologies (mini-gut models, cobots) need to include these interactions to ensure accurate control and outcomes.
Introduction
The microbiome, poultry productivity and health are all interconnected and show significant variability due to nutritional, environmental and host-related factors (; Kers et al. Citation2019).
Figure 1. A conceptual overview of interacting factors, issues and concepts covered in this article.
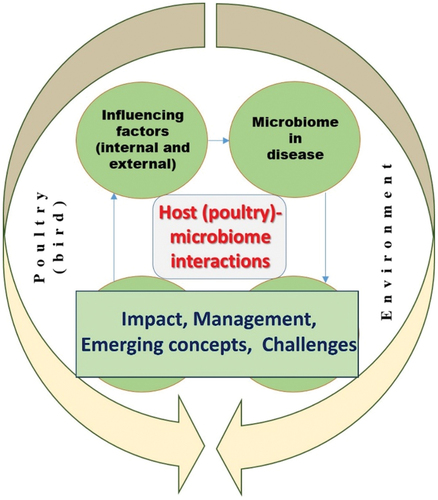
These factors affect the type and amount of pathogenic and commensal microorganisms which poultry is exposed to during growth in various farming systems. Growth stage and feeding phase and type influence the host-microbiome function and composition (Tan et al. Citation2019). The gut microbiome is essential to health and homoeostasis maintenance and modulation of physiological activities (immunity, metabolism, nutrition) in the host. A healthy microbiome contributes to enhancement of bird performance and productivity (Bindari and Gerber Citation2022; Diaz Carrasco, Casanova, and Miyakawa Citation2019).
There is a geometric growth in demand for poultry products (Organization for Economic Co-operation and Development/Food and Agricultural Organization Citation2017) and birds are effective feed converters which is key to meeting growing global demands for protein. Nonetheless, poultry are still prone to disease outbreaks especially in locations with changing micro- and macroclimates (Pin Viso et al. Citation2021).
The gastrointestinal tract (GIT) is densely populated with microorganisms that interact with the host and ingested feed (Pan and Yu Citation2014). The GIT in birds harbours a complex microbiota that conducts the key tasks of nutrient absorption and pathogen defence (O’Hara and Shanahan Citation2006). In pathogen defence, a protective barrier produced by the gut microbiota reduces the colonisation of pathogenic bacteria (Yegani and Korver Citation2008). Changes in diet and treatment interventions enhance the growth of poultry and reduce the risk of enteric infections (Kohl Citation2012). Interactions within the intestinal microbiome with micro-organisms, host and diet have profound effects on poultry nutrition and health (Daniel, Lecuyer, and Chassaing Citation2021). These interactions are a result of nutrient exchange, host gut morphology, physiology and immunity and are of great importance to poultry production (Pan and Yu Citation2014).
An important role for beneficial microbiota is the maintenance of normal physiological homoeostasis, host immune system modulation and metabolism (Sommer and Bäckhed Citation2013). Changes in the microbial community in the GIT can adversely affect poultry feed conversion efficiency, productivity and health (Kohl Citation2012), as well as local and systemic immune responses (Abaidullah et al. Citation2019).
The resident microbiota in the GIT regulates extra-intestinal immunoregulatory network of acquired immunity and promotes elimination of pathogens through opsonisation (W. H. Kim and Lillehoj Citation2019). The beneficial attributes of poultry commensal microbiota include competitive exclusion, antibody production (immune system stimulation and development) and cytokine activation, all of which are used to protect against pathogens or non-indigenous microbes (Abaidullah et al. Citation2019). Stimulated immune systems include the mucus layer, epithelial monolayer and the intestinal immune cells (cytotoxic and helper T cells, immunoglobulin producing cells and phagocytic cells) (Oakley et al. Citation2014). These tissues play a role in developing barriers between the host and microbes to contest undesirable gut microorganisms (Mwangi et al. Citation2010).
The important roles for poultry GIT microbiota include nutrient absorption (Cole and Boyd Citation1967); enzymatic breakdown of indigestible dietary polysaccharides to their compositional fermentable sugars and short chain fatty acids (SCFA) (Dunkley et al. Citation2007), and welfare of the host (Hai et al. Citation2010). The SCFA stimulate the proliferation of epithelial cells present in the gut, increasing the absorption surface area (Dibner and Richards Citation2005). Additionally, the bacteriostatic properties of SCFA allows for the eradication of foodborne pathogens such as Salmonella spp (Ricke Citation2003). In addition, the microbiota stimulates metabolism of nitrogen, which is incorporated into bacterial cellular proteins allowing bacteria to serve as sources of amino acids or proteins and vitamins to the host (LeBlanc et al. Citation2013).
Several technologies have focussed in giving relevant insights into the interconnected nature of biotic and abiotic factors impacting poultry health and the identification and detection of associated beneficial of harmful microbiota. Some of them include bioinformatics and next-generation sequencing (NGS), ‘big data’ analytics, robotics and sensors, artificial intelligence (AI) and machine learning (ML) based workflows. New detection and monitoring technologies could drive the future of poultry systems management (Gowda Citation2023).
In light of this, this paper reviews on factors affecting the evolution of the poultry gut microbiome, associated host–microbiome interactions and the influence of microbiome disturbance (dysbiosis) in poultry disease, health and productivity. The roles of various current and emerging technologies in advancing scientific insight on the aforementioned topic under investigations are discussed.
Factors influencing the poultry gut microbiome
A healthy gut is critical to the development of intestinal immunity (Kogut Citation2019). The poultry microbiome is influenced by factors such as diet (Schokker et al. Citation2021), host (breed, sex), age or stage of life, habitat fragmentation (wild or laboratory reared) (Morrow et al. Citation2015) and/or environment (poultry handlers, proximity to humans, litter, access to feed, climate, location; Bindari et al. Citation2021). The microbiome affects animal adaptation to environmental changes, immunity development or maintenance and nutritional supplementation (Clavijo et al. Citation2022).
Profiling the GI microbiome is particularly important for understanding both host and gut health (Dietert and Silbergeld Citation2015). Fluctuations in microbiota alter the microbiome profile. Poor GI health has been linked to nutrient malabsorption and growth depression in affected poultry (Bailey Citation2010). The major domains related to gastrointestinal physiology, animal health and welfare include diet, digestion and absorption of dietary nutrients, resident microbiota and immune system development for disease prevention (Celi et al. Citation2017; Willing and Van Kessel Citation2010). As such, the gut plays an important role in regulating host physiological functions (Weber Citation2017).
Interplay of chemical and host-related factors
The interplay of physiological, nutritional, microbiological, immunological and physical characteristics of a healthy gut dictates the maintenance of intestinal homoeostasis (Polansky et al. Citation2016). Imbalances in the GI microbiome adversely impacts health and the GIT microbiome is key to maintaining a mutualistic relationship between the immune system of the host and the microbiome (Aruwa et al. Citation2021). The gut has a large surface area and is in constant interaction with the environment. Therefore, its epithelial lining has an effect in reducing exposure to toxins in the environment and provides a barrier against pathogen colonisation (Roberts et al. Citation2015). In a study by Lan et al. (Citation2004), it was noted that changes in microbiome profile may result in stunted growth, weakened host resistance and susceptibility to infectious diseases. The composition of the gut microbiota is influenced by the usage of antimicrobials and exogenous feed enzymes (H. Rehman et al. Citation2008). The gut microbiota promotes digestion and fermentation of indigestible feed to provide essential amino acids and vitamins required by the host (Oakley and Kogut Citation2016). The establishment of a more favourable microbiome is necessary for optimal poultry growth and performance and, if not established, can lead to enteric infections, depressed growth rates and an increase in mortality rates (Kogut Citation2019).
During the passage of feed through the gut, starch hydrolysis and lactate fermentation by predominant resident Lactobacillus spp. (at 109 cells/g) occurs in the crop of poultry (Stanley, Hughes, and Moore Citation2014). Both mechanical and chemical degradation of the feed occurs within the gizzard (H. U. Rehman et al. Citation2007) and acidic conditions created in the stomach by hydrochloric acid and pepsin are key to decreasing the microbial populations to lower than 108/g (Yeoman et al. Citation2012). Larger bacterial populations are found in the small intestine and dominated by Lactobacillus, Enterococcus and Clostridiaceae spp (Waite and Taylor Citation2015). Mash feed in broiler chickens has been shown to decrease the number of Enterococcus spp. and coliforms and promotes the growth of Lactobacillus spp. and Clostridium perfringens when compared to pelleted feed (Knarreborg et al. Citation2002). It has been reported that wheat-based diets promote bifidobacteria presence (with higher percentage of guanine-cytosine (GC) content), while corn favours a lower GC percentage of Clostridia, Enterococci and Lactobacilli spp (Apajalahti, Kettunen, and Graham Citation2004). Within the caeca, Firmicutes, Bacteroides and Proteobacteria are the dominant phyla followed by populations of Actinobacteria. The majority belong to various Clostridiales spp., known as important contributors to SCFA production (Sergeant et al. Citation2014). Bacteriodetes were reported to be of greater abundance, with low abundance of Firmicutes from d 0 to 42 as poultry birds moved from a starter to a finisher diet (Kumar et al. Citation2018).
The gut microbiome is an inoculum source that can be transferred from one flock to another (Liljebjelke et al. Citation2003). The gut is naturally colonised prior to and after hatch through transfer of microbes through eggshell pores (S. Lee et al. Citation2019). The transfer of innate colonisers is possible from parent fowls to offspring (Medvecky et al. Citation2018) and can be altered by extraneous environmental factors at various stages of development (Ding et al. Citation2017). Newly hatched commercial chicks generally have no contact with adult birds and the environmental microbiota promotes the development of the microbiome (Oakley et al. Citation2013). The post-hatch period is important for growth and health as the newly hatched chick moves from yolk to a carbohydrate and protein rich-diet (Cheled-Shoval et al. Citation2011). Of significance, in the developmental stages, is the microbial succession in hatchlings which start with members of the Enterobacteriaceae (at one-day-old poultry), followed by Firmicutes (at 7 d old) (Ballou et al. Citation2015). However, this succession with specific strains may be associated with the inherent microbiota seeded from drinking water, feed or bird interaction (Kubasova et al. Citation2019). The richness of the microbiome increases with time and age until it reaches maturation (Jurburg et al. Citation2019).
Microbiome maturation takes about 21 d in broiler chickens, but varies with farm practices and the bird’s genetic background (Ngunjiri et al. Citation2019). Studies have shown that microbial succession in the microbiome occurs in response to the prevailing cytokine profile, such that Proteobacteria spp. increase in a pro-inflammatory state, while anti-inflammatory conditions favour an increase in Firmicutes (Oakley and Kogut Citation2016). Hence, the importance of the microbiome in immune balance/homoeostasis. Current studies are focused on favourably influencing the innate/early microbiome profile of poultry birds from birth via the infusion of probiotics, prebiotics and specific microbial consortia directly on, or into, the eggs (The Nutrition Source Citation2023; Rubio Citation2019). Interestingly, Fisinin et al. (Citation2016) showed that the GI microbiome formation began from the embryonic stage in chicks and deemed this essential for developing healthy birds at hatching. Microbiome diversity increased with age and maturity from d 6 to 150 and only showed a decline at 300 d old. Changes in the GI microbiome composition have been observed throughout the life span (42 d) of commercial broilers. The higher abundance of beneficial bacteria and a decreased level or absence of pathogens could lead to an improvement in broiler performance (Yeoman et al. Citation2012) and a reduction in dietary energy requirements in chickens when unfed for long periods (Muramatsu, Nakajima, and Okumura Citation1994).
The use of antimicrobials in poultry farming are a necessity for disease control (Mughini-Gras et al. Citation2020), growth improvement and reduction in poultry morbidity and mortality (Niewold Citation2007). However, the removal of antibiotics in feeds can alter the homoeostasis of the gut microbiome of poultry. Poultry age and dose can further influence antibiotic effects on the gut microbiome (Zhou et al. Citation2007, Pedroso et al. (Citation2013) reported that, in poultry groups grown with and without antibiotics, variations in the microbiome profile were insignificant and that it managed to stabilise and resist diversity changes after withdrawal of medication (Dethlefsen et al. Citation2008). Nevertheless, in recent efforts, a Salmonella-based phage cocktail did not show adverse impact on normal microbiome development and had the potential to serve as an alternative to antibiotics. The cocktail drastically decreased Campylobacter spp. populations and up-regulated levels of commensal GI microbiota that could have favourable impacts on poultry metabolism and health (Clavijo et al. Citation2022). In addition, the use of plant products like Moringa oleifera powder as alternative to antibiotics when added to feed showed favourable modulation of microbiome balance through upregulation of beneficial Bacteroides spp. and their metabolites (Soundararajan et al. Citation2023).
Given the ban on antibiotics as poultry bird growth promoters, high fibre diets are being explored as their use in moderate quantities have been linked to nutrient digestibility, enzyme secretion and organs development in poultry as a result of an enhanced gizzard function and better nutrient-enzyme contact (Mateos et al. Citation2012). The contribution of some amount of fibre can favourably impact poultry health. It has been suggested that fibre content of between 2% and 3% in feed, which is inclusive of insoluble fibres such as oat hulls, could enhance growth and productivity in broilers (Mateos et al. Citation2012). In some studies, fibre meal ratios prepared from corn, soybean and wheat bran were fed to layers and broilers to determine any effect on the caecum microbiome and SCFA levels (Sun, Hou, and Yang Citation2021; Walugembe et al. Citation2015). Fatty acid levels were shown to be higher in broilers than layers and microbiota changed under the influence of diet and chick breed. The relative prevalence of Enterobacteriaceae spp., Bacteroides spp., Faecalibacterium spp., Megamonas hypermegale, Helicobacter pullorum and Campylobacter spp. varied across chicken groups (Walugembe et al. Citation2015).
In addition, feed incorporated with enzymes are widely used in poultry production. These improve the performance of poultry birds by modifying the gut microbiota and gut environment (Bindari and Gerber Citation2022). Similarly, the use of Saccharomyces cerevisiae in broiler feed favourably impacts gut morphology (goblet cells, atrophy and inflammation) and reduce aflatoxin levels in chickens fed contaminated diets (Poloni et al. Citation2020). This can be achieved without reducing the nutritional value of the feed compared to known feed additives, for example, zeolites and clinoptilolite, which may have deleterious effects (Zain Citation2011), besides being used as an adsorbent for aflatoxins (Nemati et al. Citation2014). Likewise, S. cerevisiae inclusion in postbiotic feed formulations have been shown to decrease burden of Salmonella enterica in chicken broilers (Chaney et al. Citation2022). Some members of the Bacillus genus, for example, Bacillus subtilis, have been used to enhance broilers total mean weight and GIT health under challenge conditions (Keerqin et al. Citation2021). Feed conversion efficiency was improved in broiler chickens with L. pentosus ITA23 and L. acidophilus ITA44 added to feed at 109 cells/kg of feed. Escherichia coli was significantly reduced, while number of Lactobacillus spp. increased in the caecal microbiome following supplementation (Altaher et al. Citation2015). Lactobacillus spp. (as a probiotic) in poultry feeds can contribute to effective feed conversion and absorption, as well as weight gain in poultry (Oakley et al. Citation2014). Villus height and perimeter within the jejunum and GI muscle thickness have been increased with the use of Bifidobacterium thermophilum and Enterococcus faecium (Chichlowski et al. Citation2007). Broilers serum cholesterol reduction have been achieved using an assortment of Lactobacillus spp (Jin et al. Citation1998).
In addition, prebiotic supplementation has an impact on gut microbiota composition (S. H. Lee et al. Citation2020). Compared to pelleted feed, coliforms and Enterococcus spp. populations have been reduced in poultry fed mash feed, followed by incremental increases of C. perfringens and Lactobacillus spp. in the ileum of broiler chickens (Knarreborg et al. Citation2002). More Bacteroidetes than Firmicutes spp. have been recorded in 0 to 42 d old birds when diets changed from starter to finisher diets. This was attributed to Bacteroidetes spp. ability to produce sugars from starch fermentation (Kumar et al. Citation2018).
Poultry health could be directly enhanced using the prebiotic fructo-oligosaccharide (FOS), which was not seen with mannan-oligosaccharide (MOS) (Xu et al. Citation2003). The use of FOS has been reported to improve activity of beneficial species, without impeding pathogen activity (Xu et al. Citation2003). The secretion of enteric mucus (mucins) is improved in the presence of MOS which contributes to the maintenance of poultry gut health. Mucus production and thickness serves as an integral line of defence against invading pathogens (Stanley, Hughes, and Moore Citation2014). In mildly stressed environments, MOS can increase the gut surface-area to volume ratio via higher goblet cell numbers in poultry (Stanley, Hughes, and Moore Citation2014), suppressing enteric pathogens and enhancing the immune system (Baurhoo, Phillip, and Ruiz-Feria Citation2007). In other studies, Bacteroidetes spp. populations were unaffected, while the number of Firmicutes spp. was higher with MOS treatment (Turnbaugh et al. Citation2008). Enhancement in nutrient absorption capacity of chickens has been reported for Firmicutes spp (Jumpertz et al. Citation2011). Supplementation with MOS decreased Salmonella spp. abundance in the caecum (Fernandez, Hinton, and Van Gils Citation2002) and reduced E. coli populations (Baurhoo, Phillip, and Ruiz-Feria Citation2007) in infected chickens. This was linked to mannose being able to attach to lectins in Gram-negative bacteria with type 1 fimbriae, thus decreasing the number of possible attachment sites in the epithelial cells of the gut (Ganner and Schatzmayr Citation2012).
Galacto-oligosaccharide (GOS) supplementation can influence the chicken gut flora by regulating the bird’s innate immunity (upregulated interleukin-17A (IL-17A) expression in chicken caecum and ileum) and microbiome profile (increased relative abundance of Lactobacillus johnsonii in GOS-fed broilers compared to Lactobacillus crispatus), giving improvements in performance (Richards et al. Citation2020). An increase in Bifidobacterium and Lactobacillus spp. facilitated by FOS and GOS results in an increase in SCFAs and lactate and a decrease in the colonisation of Salmonella spp. in both female and male broilers (Emami et al. Citation2012). Fructo-oligosaccharide is used as a promoter for growth and fermentation by Bifidobacteria spp. such as Bifidobacterium fiagilk, Bifidobacterium thetaiotaomicron, Bifidobacterium vulgatus, Bifidobacterium dktasonk and Bifidobacterium ovatus (Hidaka and Hirayama Citation1991). The secretion of the ß-fructosidase enzyme leads to FOS degradation and fermentation. In addition, the inclusion of FOS in the diets have resulted in a decline in population growth of C. perfringens and E. coli and an increase in Lactobacillus spp. in the broiler GIT (H. J. Kim et al. Citation2011). Synergistic effects can be created by supplementing with a combination of probiotics and prebiotics to create synbiotics (The Nutrition Source Citation2023). This has been shown to improve poultry GIT health, promote weight gain and inhibition of Salmonella and Campylobacter spp. colonisation (Ricke Citation2015).
Interplay of management systems and physical factors
The housing environment, climatic conditions and litter influence the presence of a more complex microbial community in the poultry gut (Shang et al. Citation2018). The role played by relative humidity and temperature associated with seasonal changes and external climatic conditions, especially in commercial intensive farms, are relevant factors to consider in poultry growth (Farag and Alagawany Citation2018). Microbiome richness and diversity in caecal samples collected during summer months has been demonstrated to be double that reported in winter (Diaz Carrasco Citation2019) and similar reports are available from several broiler chicken flocks in America (Oakley et al. Citation2018). In addition, particular management systems () employed within poultry farms, such as time of feeding, feed type, free range access, ventilation, heat stress, biosecurity, hygiene processes, means of treatment, vaccination program and fowl stocking density, influence the microbiome and bird productivity level (Diaz Carrasco, Casanova, and Miyakawa Citation2019; Göransson et al. Citation2023).
Bedding material can impact the prevailing microbiome (Wang et al. Citation2018) as birds ingest litter which accumulates in droppings, indicating a constant interaction among birds and their gut microbiome and the environment (Diaz Carrasco Citation2019). When litter is reused to cut poultry production costs or when high moisture litter beds are used, an increase in bacterial load is recorded which implies that disease-causing microorganisms could be transmitted among flocks (Cressman et al. Citation2010). Microbial colonisation of poultry from environmental sources has major biosecurity and management implications especially when there is transfer of human pathogens to consumers via the poultry supply chain. These risks can be mitigated by constant monitoring of the entire food supply chain (farm-to-fork) as outlined in the Food Safety Modernisation Act (Aruwa Citation2020; Rittenberry Citation2011) and should be practiced as a continuum (Oakley et al. Citation2013).
In other efforts, copper and zinc metals made available in a nanoscale alloy form have shown promise as mineral additives for broiler feeding and could serve as replacements for organic or inorganic sources in poultry nutrition and health. Nanomaterials have high penetrative abilities and the metals may enter the gut and bypass transport proteins (Sizova et al. Citation2020). Similar results have been obtained when the arginine, methionine and lysine were delivered through iron nanoparticles in broiler chickens, leading to better weight gain (20%) and may help in increasing meat yields (Yausheva et al. Citation2016).
Poultry microbiome, dysbiosis, infectious and metabolic disorders
The poultry gut microbiome evolves such that, when disturbed (in a dysbiotic state), it can cause metabolic disorders. The microbiome develops immediately after hatching and diversifies to a relatively stable dynamic state (Ducatelle et al. Citation2023), but is readily changeable by diet, ingestion of antibiotics, infection by pathogens and other host- and environmental-dependent factors (Chow and Mazmanian Citation2010). Certain nutrients recognised by the innate immune system in poultry may trigger pathways that instigate disorder in nutrient metabolism and immunity, thus affecting the microbiome (Kogut Citation2013). The interconnected parts which make up the GI ecosystem include the commensal microbiota, immune system and intestinal epithelium. Host nutrients influence commensal diversity and host immunity impacts the microbiome (Nicholson et al. Citation2012). Metabolic responses and immunity are closely related, such that metabolites from the diet and microbial populations could influence inflammatory responses which give rise to metabolic disorders (Caesar, Backhed, and Bäckhed Citation2010). However, metabolic disease pathogenesis and aetiology in poultry may involve multifaceted conditions including gut microbiome shifts (Lavelle et al. Citation2010), changes in metabolism of certain nutrient-like glucose and lipids (Wen, Ting, and O’Neill Citation2012), rapid growth induction, excess nutrient intake which causes inflammation (Hotamisligil Citation2006), stress, infectious agents and toxin exposure (Julian Citation2005).
The GIT microbiota serves as a barrier for prevention of incoming pathogens and plays an important role in competitive exclusion for microbial attachment sites or nutrient sources (Sekirov et al. Citation2010). Poor gut health from an unbalanced microbiome or enteric disease results in poor poultry production (Danzeisen et al. Citation2013). Nutrients affect the morphology and function of the gut microbiome, as well as microbiome diversity and microbial genetics (Kogut Citation2022; Schokker et al. Citation2021). Physiological roles are provided by the gut microbiome which the host cannot provide themselves (L. C. H. Yu et al. Citation2012). During metabolism in microorganisms, fat storage is regulated, essential vitamins are produced and energy yield is upregulated due to the breakdown of indigestible polysaccharides in fermentation (Stecher et al. Citation2007). The microbiome likewise renews the gut epithelium that doubles as a barrier (Koch and Asma Citation2012) and contributes to xenobiotic processing, gene expression, cell differentiation and host energy. The poultry feed-microbiome interaction affects physiology, shapes immune response, balances energy, nutrient digestion and absorption, regulation and production of biologically active metabolites (Kogut Citation2022).
The chicken gut houses around a million bacterial genomes and over 450 phylotypes. Most caecal bacteria have only recently been identified by metagenomic methods and some remain unculturable (Sergeant et al. Citation2014). Microbiome plasticity is involved in some disease conditions in poultry and could facilitate dysbiosis (DB), which could give rise to infections contacted from various microorganisms (). The DB state causes a surge in circulating microbial products, such as lipopolysaccharides from the microbiome, and which innate immunity entities recognise. This state alters the ratio of beneficial to harmful strains in the gut (Kipanyula et al. Citation2013); hence, energy intake and generation from feed is negatively impacted (Torok et al. Citation2011). Complications surrounding the poultry microbiome have a significant impact on T cell receptor reserve dynamics and T lymphocytes (Ivanov et al. Citation2008). This suggests the presence of potent species with immunomodulatory functions. Metabolic balance/homoeostasis is directed towards host protection/immunity. The organisation of organs (adipose tissue and liver), where immune cells and macrophages are close to metabolic cells (adipocytes and hepatocytes), make maintenance of metabolic homoeostasis more evident (Hotamisligil and Erbay Citation2008) and these cells share signalling pathways (Henao-Mejia et al. Citation2012). Antibiotics are known to upset the balance of the host normal microbiota and induce a DB state. Therefore, sub-therapeutic doses of antibiotics are incorporated in a broiler diet as growth promoters and prevent diseases (Torok et al. Citation2011). The precise mode of action of antibiotic growth promoters (AGPs) are still not fully understood; however, withdrawal of AGPs from feed have been associated with an increased incidence of intestinal disorders (Broom Citation2017). Feeds supplemented with antibiotics such as avoparcin or virginiamycin have previously been used in necrotic enteritis prevention as these antibiotics alter the structure of the GIT microbiome (M. D. Lee Citation2008). In addition, the poultry GIT microbiome serves as a source of bacterial pathogens such as Salmonella and Campylobacter spp. that could disseminate to humans and as reservoirs of antibiotic-resistance they pose serious risks to public health via the food chain (Kumar et al. Citation2018).
Table 1. Poultry microbiome groups associated with various poultry diseases.
In metabolically induced inflammation (‘meta-inflammation’ or MI), which is associated with nutrient sensing, nutrient and a surplus of metabolites serve as triggers. The MI is a chronic condition, considered a metabolic disease which leads to chronic allostasis (Hotamisligil Citation2006). In contrast, classical inflammation (CI) is considered acute as it triggers immune defences, is induced by invading microbes or presence of injury to the gut and is resolved by reverting to a homoeostatic state. Typical responses to CI include pain, redness, fever and swelling (Barton Citation2008).
In a different scenario, it has been hypothesised that the immunity-gut microbiome interaction may be linked to lameness in poultry (Kogut Citation2013). Due to their impact on performance and welfare, lameness and/or musculoskeletal disorders are classified as metabolic diseases (Angel Citation2007). Less lameness in 1 d old birds through diet supplementation with probiotics has been reported (Wideman et al. Citation2012). Poultry lameness is caused by bacteria, that is, chondronecrosis and osteomyelitis () (Dinev Citation2009), which are translocated to the legs from the blood and GIT in a dysbiotic state and has been linked to immune suppression under stressed rearing conditions (Wideman et al. Citation2012).
Any alterations to the chicken microbiome can promote proliferation of pathogenic organisms (Morishita and Mitsuoka Citation1976). Recognised enteric disease syndromes in poultry include the runting-stunting syndrome (RSS) in young broiler chickens and PEC in young turkeys (Barnes and Guy Citation2003; M. J. Pantin-Jackwood Citation2013). Clinical symptoms of enteric disease in poultry include diarrhoea, wet litter in housing environments, reduced feed intake, dehydration, depressed growth and fluctuations in size (Barnes, Guy, and Vaillancourt Citation2000). Campylobacter jejuni is the most common cause of food-borne bacterial enteritis (European Food Safety Authority (EFSA) Citation2011). Still, in certain cases understanding the interplay between C. jejuni and poultry commensals could be key to developing new strategies to reducing its populations in broilers (Al Hakeem, Acevedo Villanueva, and Selvaraj Citation2023). However, this microorganism could overcome the host’s defence system and compete for space and nutrients with the commensal microbial community (Awad et al. Citation2016). Clinically affected birds usually have pale, thin-walled intestines consisting of undigested feed. With microscopic analyses, the observation of blunted absorptive intestinal villi indicates the inability of the intestines to absorb nutrients from the feed (Songserm et al. Citation2000).
Diets fed to poultry before maturation and which are low in phosphorus and high in calcium can cause visceral gout leading to chronic kidney failure and bird death. Caged or sedentary hens and turkeys with unbalanced protein and energy intakes develop fatty liver syndrome (Mwato Citation2023). The incorporation of microencapsulated enzymes (to enhance stability and recoverability for reuse) in poultry feed could boost feed digestibility and poultry wellbeing and reduce feed cost (Maxxperformance Citation2023). In certain practices, chickens are hatched in the clean environment of hatcheries and the neonatal GIT may not be colonised by beneficial bacteria. This however increases the susceptibility of the chicks to pathogenic microbes that out-compete the beneficial microbes, once in direct exposure to a potent source of pathogens. Therefore, with the incorporation of probiotics or other bioactive compounds, early colonisation with healthy bacteria promotes the establishment of the intestinal microbiota and protection from pathogens (Pender et al. Citation2016).
The pathogenic microbiome in poultry diseases
Associated bacteriome
Some qualitative similarities in poultry microbiota have been linked to the existence of pathogens, altered GI microbiota, nutrient absorption deficiencies or poor immune development in poultry (Calvert Citation2012; Moussavi et al. Citation2007). Given the role of the GI microbiome in the production of various metabolites, studying this relative to other factors, rather than just evaluating the impact of eubiotic interventions constitutes an explorable study niche (Kang et al. Citation2021). For example, in dysbacteriosis, the microbiome has been associated with visible changes in the thickness, appearance, muscle tone and tensile strength of the poultry GI wall (Collier et al. Citation2008). While it is common practice for poultry feed to contain high protein (Velthof, Hou, and Oenema Citation2015), unused nitrogen is released as ammonia (NH3) which has a detrimental impact on health and the housing environments (Dai and Karring Citation2014). In broilers, NH3 is toxic to enterocytes when absorbed through the intestinal epithelium (Rinttilä and Apajalahti Citation2013). Nonetheless, the incorporation of fermentable carbohydrates in the diet may reduce harmful metabolites formation from proteinaceous feeds and increase SCFAs concentrations (Heo et al. Citation2013).
Clostridia spp. have been implicated in dysbacteriosis, necrotic enteritis, gangrenous dermatitis and non-specific enteritis (Petersen and Round Citation2014) although the role played by the microbiome in diagnosis remains debateable (Daniel, Lecuyer, and Chassaing Citation2021). Whatever the cause of microbiome imbalances or enteric disease, the ability of poultry to efficiently utilise available nutrients in feeds is usually impacted. Indeed, financial losses are usually higher for asymptomatic and acute infections such as necrotic enteritis (Choct Citation2009). Bacterial pathogens that affect poultry include Staphylococcus, E. coli, Clostridium, Campylobacter and Salmonella spp (Oakley et al. Citation2014). Other poultry normal bacterial flora may become opportunistic when transferred to humans (Newell et al. Citation2011). In line with this, the concept of competitive exclusion has been floated as an efficient therapy to block pathogens transfer, thus enhancing GI regulation. Imitation of binding sites for pathogens in the GI epithelium using false signal binders has been shown to impede a pathogen’s ability to recognise and bind to gut target sites (Oakley et al. Citation2014).
Associated virome
The most prevalent viruses causing runting-stunting syndrome (RSS) in chickens are the Parvoviridae, Astroviridae, Picornaviridae, Caliciviridae, Myoviridae, Siphoviridae and Coronaviridae families (Devaney et al. Citation2016). However, some distinct families remain unidentified and unclassified. Others include the chicken calicivirus, astrovirus, parvovirus, avian nephritis virus and novel chicken sicinivirus 1 and megrivirus. Likewise, the Circoviridae, Adenoviridae, Picobirnaviridae, Caliciviridae, Parvoviridae, Reoviridae and Picornaviridae families have been reported in healthy chicken faecal samples (Lima et al. Citation2017).
Asymptomatic wild birds act as pathogen reservoirs linked to emerging infectious viral disease such as West Nile virus (WNV), avian influenza virus A (H10N7) and severe acute respiratory syndrome (SARS), all of which are transmissible to humans (Mackenzie and Jeggo Citation2013). As reservoirs, these birds constitute a great risk to domesticated poultry. Comprehending viral diversity detection enhances the ability to predict outbreaks and transmission risks of viral diseases. Asymptomatic pathogen reservoirs contribute to virus-host coevolution and increased risk of recombinant or mutant viral strains. The asymptomatic state in poultry could be an adaptive immune response or linked to peculiar bird metabolism (Ayginin et al. Citation2018). A molecular technique, like DNA barcoding, has not been sufficient for the detection of many viruses since it targets majorly and only conserved regions in the genome, limiting their ability to detect new viral strains. Again, there is the lack of a general genetic marker for viral identification. Hence, universal primers for viral genome amplification and differentiation could enhance poultry virome detection, and characterisation. Interestingly, oligonucleotide designs for significant coverage and detection of known and novel viral variants in a sample, mixture of samples or biological materials have been developed (Ayginin et al. Citation2018), and are used to detect circovirus, avian coronavirus, Turnip yellow mosaic Virus, Tunis virus, Lake Sarah-associated circular virus-32 in Siberian Thrush (Geokichla sibirica) and Bean Goose (Anser fabalis johanseni) (Ayginin et al. Citation2018).
The elucidation of virome across environments, time and host species has been explored (Bergner et al. Citation2019) using non-invasive, untargeted shotgun metagenomic sequencing. Although difficult to characterise, the PEC and runting-stunting syndrome (RSS), among others (), in broilers are some of the syndromes associated with poultry viral infections. These infections may be mono- or polymicrobial in nature (Roussan et al. Citation2012). Rotaviruses cause infections alongside reovirus and astrovirus, but are detected in healthy (asymptomatic) poultry (M. Pantin-Jackwood et al. Citation2008). Of the five astrovirus strains, two are of turkey origin (TAstV-1 and 2), two of chicken origin (CAstV and ANV) and one originates from ducks (DAstV). While four strains are entirely host-specific, ANV was detected in both chicken and turkeys (M. Pantin-Jackwood et al. Citation2008). For the coronavirus family, Coronaviridae and genus Gammacoronavirus cause infectious viral enteritis in turkey (Chen, Wu, and Lin Citation2015). Infectious bronchitis in chickens is caused by the Infectious Bronchitis Virus (IBV), a coronavirus and can further predispose poultry to colibacillosis, followed by bacterial infections resulting in increased mortality (Matthijs et al. Citation2003). Stunted bird growth, reduced feed conversion efficiency, poor production, high cost of treatment, compromised immune systems and death are among the economic significances attributed to poultry diseases. The preventative control of viral disease mostly involves vaccination (Kogut et al. Citation2021).
Associated parasitome in poultry diseases
Parasites of the Apicomplexa phylum cause coccidiosis in many animals including poultry (Shirley, Smith, and Tomley Citation2005). This intestinal disease is caused by Eimeria species, but poultry response to infections caused by Eimeria spp. require more scientific insight (MacDonald et al. Citation2019). Seven species infect the Gallus gallus domesticus (domesticated chicken) causing malabsorptive enteritis (Eimeria acervulina, Eimeria maxima, Eimeria mitis, Eimeria praecox) or haemorrhagic enteritis (Eimeria brunetti, Eimeria necatrix, Eimeria tenella) (Shirley, Smith, and Tomley Citation2005). Three Eimeria spp., namely, E. acervulina, E. maxima and E. tenella are mostly found in intensively reared chickens (Clark et al. Citation2016). The co-infection of Eimeria species with bacterial pathogens such as C. perfringens or S. enterica serovars enteritidis or typhimurium could also intensify the infection. However, a healthy GIT microbiome could limit the colonisation of Salmonella and Campylobacter spp., as well as transform fungal mycotoxins present in feed to non-toxic derivatives (Hai et al. Citation2010). In one study, the oral administration of a chick peptide (Nk-12) expressed in B. subtilis improved poultry gut and host health in broilers infected with coccidiosis (Wickramasuriya et al. Citation2023).
Parasite interactions with bacterial members of the poultry microbiome could be harmful or beneficial. Adverse effects include body weight loss, death, morbidity and increased costs for vaccination and control. While parasites may worsen or protect the host against inflammatory infections and DB, the microbiome could affect the parasite’s virulence and ability to colonise and replicate (Leung, Graham, and Knowles Citation2018). The presence of Eimeria enhance C. perfringens growth in poultry gut with inhibition of beneficial bacteria (Hauck Citation2017). In poultry co-infection, E. tenella caused increase in number of C. jejuni gut pathogens (MacDonald et al. Citation2019). In another scenario, E. tenella coccidial disease adversely affected the caecal microbiome profile (increase in harmful Prevotella pectinovora and Alistipes sp. and reduction in probiotic microbes) and chicken health and immune responses showed expression of host transcriptomes from Colidextribacter sp., Butyricicoccus porcoru and P. pectinovora (H. Yu et al. Citation2023). Chicken lesions are also caused by the parasite Histomonas meleagridis, but only in the presence of bacteria (Hauck Citation2017). The same microbe is associated with histomonosis in turkeys (Landim de Barros et al. Citation2022).
Not much is known of the interaction of Blastocystis species with bacteria in chickens. Nevertheless, in humans, the presence of Blastocystis has been linked to a shift in the GIT microbiome. A similar microbiome change may be observed between the chicken gut microbiome and Ascaridia galli, but with the secretion of antimicrobial moieties by the parasite. While these correlations are known, the mechanisms behind these parasite-gut bacteria interactions remain a key focus of scientific inquiry (Hauck Citation2017). Treatment regimens for poultry parasitic infections include medications (hygromycin B, ivermectin levamisole, sulphonamide) in feed or water coupled with improved management of poultry environment (Belete, Addis, and Ayele Citation2016).
Associated mycobiome in poultry diseases
Most filamentous fungal species linked to poultry are contaminants of poultry feed and pathogens, but some are useful as probiotics (S. cerevisiae) to improve broiler fitness by producing antioxidant molecules and enzymes useful in enhancing feed quality, digestibility and poultry bird ability to resist diseases (Sugiharto Citation2019). The major fungal feed contaminants are the Aspergillus species, that is, Aspergillus niger and Aspergillus flavus. Not so dominant species include Fusarium sp., Cladosporium spp., Mortierella sp. and Epicoecum sp., which produce spores and mycotoxins (citrinin, ochratoxins, aflatoxins, oosporein, trichothecenes, ergot alkaloids) during growth (Vera, Arosemena, and Calvo-Torras Citation2016). The presence of allergenic fungal spores of Scopulariopsis, Penicillium crysogenum, Aspergillus fumigatus and Cladosporium cladosporioides may also initiate health challenges in poultry (Sugiharto Citation2019). Among the diseases caused by fungi in poultry are favus caused by Microsporum gallinae, dactylariosis caused by Dactylaria gallopava and aspergillosis linked to A. fumigatus (), mycotic dermatitis, among others. Such diseases lead to deadly encephalitis, mycotoxin intoxications, stunted growth and other fatal infections, amounting to economic losses. The chick’s innate response tries to fight these reactions, but in the process cause further damage by activating a hypersensitivity response that brings about damage to tissues and allergies. Antifungal agents (fluco- and ketoconazole) have been used to reduce the severity of poultry fungal diseases, alongside improved sanitation, regular testing and sometimes replacement of birds with new ones (Dhama et al. Citation2013).
Emerging concepts and challenges in the poultry industry
The commensal poultry microbiome interacts with their host either directly (attachment to host epithelial cells) or indirectly (via interkingdom signalling). Bacterial molecules make up most of the signals recognised by the host using G-protein coupled receptors (GPRs) in the epithelium. Interkingdom signalling should constitute a key study area as protein receptors and their respective signal molecules play a key role in poultry GI health. The poultry lower gut area expresses the butyrate molecule as a signal that is central to both gut and overall poultry wellbeing (Ducatelle et al. Citation2023). Another key moiety in microbiome network signalling is lactate which is generated from prebiotics break down by lactobacilli or converted in broilers to butyrate by members of the family Lachnospiraceae. Unfortunately, lactate does not suffice as a metabolisable energy source for many microbes and the impact of its excess derivation and accumulation could be explored (Ducatelle et al. Citation2023), alongside neurotransmitter molecules producing members of the poultry microbiome and their impact on health, and additive or beneficial microbiome-induced mechanisms of action behind the use of non-starch polysaccharides (NSPs) (Ducatelle et al. Citation2023; E. Kim et al. Citation2022).
The ‘omics’ methods are highly automated and have greatly advanced the ability to profile microbiome features and enhance insight into pathogen and commensal functions in the gut and immune system (Kang et al. Citation2021). Yet, a large part of the virome network remain uncharacterised, alongside bacterium-virus co-interactions in poultry. A mechanism proposed for such co-interactions lies in the action of a mixture of phages which shape the GI function and constitution. The regulation (by viruses) of bacterial pathogenicity-related genes is another co-interaction mode of action and it is exemplified in the increased virulence in S. enterica in response to viral presence (Patel and Skłodowska Citation2023). However, significantly varied outcomes may be derived from such co-interaction studies, thus demonstrating the difficulty in comprehending co-interactions research. Still, the outcomes do not undermine the negative impact of bacterial DB and gut viral infections on poultry growth and health (Patel and Skłodowska Citation2023).
In spite of the foregoing, DB remains a cause and effect (Petersen and Round Citation2014). This scenario presents an intricate challenge because DB as an indicator of infection may also be indicative of microbiome adaptation (adaptive shift). In the latter scenario, microbiome strains which compete effectively with pathogens are increased in population and serve as an immune response (Zaneveld, McMinds, and Thurber Citation2017). Just as the upward regulation of beneficial to competitive microbiome does not always result in host morbidity, it may not further result in or worsen a dysbiotic state. The determinant of whether a host yields to infection or survives is virtually a consequence of the microbiome’s ability to respond to a pathogenic invasion rapidly and effectively. Beneficial microbiome shifts may achieve restoration or prevention based on the therapeutic target. It may also result from microbiome engineering (Aruwa et al. Citation2021; Fathima et al. Citation2022). Characterizing poultry GI complex microbiome and studying their impact on disease incidence and emergence, poultry performance and human health constitute an evolving research field with economic significance (Day et al. Citation2015). Advancements in nucleic acid sequencing techniques have expanded research possibilities regarding the exploration of microbiomes and at an unparalleled level (Yeoman et al. Citation2012).
Overall, poultry rearing methods could be evolved by embracing new technological frontiers in preparation for future economic shocks such as those experienced to date with the emergence of COVID-19 and to avoid significant losses in poultry meat production. Some of these include the incorporation of real time and on-site remote sensing of interconnected farming system factors. This would lead to generation of relevant big data and digitisation of the industry. The inclusion of robotics [cobots or collaborative robots that working alongside humans; and autonomous artificial intelligence (AI) based robots and sensors], use of composite housing (antimicrobial and corrosion resistant gearboxes), as well as application of nanotechnology, bioinformatics and/or big data analytics workflows (for serovars identification and detection e.g. Salmonella), farming system simulation prototypes, feed cast/level reporting online portals, block-chain based value management and transport genie alerting sensors (micro-climate or environment condition recorder and feedback system) could further improve poultry farming systems, production metrics, welfare and overall working conditions (Gowda Citation2023). Mini-gut models have also been developed and used to decrease the use of animal models in poultry research, thus facilitating the development of novel preventative and therapeutic strategies against poultry disorders (Doughman Citation2023). These technologies have a high propensity to shape the future of the poultry industry and contribute to enhanced production of safe and healthy poultry meats.
Conclusions
While technological advancements have improved our understanding of the poultry microbiome and their function in poultry disease and health, increasing study insight into the GIT ecosystem, range of influencing factors and host (poultry)-microbiome interactions could aid scientists in fully grasping the extent of poultry microbiome dynamics. These could also help with better understanding the emerging science of intestinal ecosystems and chat a healthier course for the treatment and management of poultry diseases. How poultry microbiome domains shift in response to environmental and growth parameters and monitoring shifts in prevalence of certain microbial species relative to particular feed formulations should remain relevant study foci. Factor to feed combinations also provide endless possibilities for exploration. Also, it is essential to combine several efficient techniques for the identification of beneficial poultry microbiome strains that play an integral role in poultry health maintenance. Nanotechnological application to diet factors could be explored to improve poultry health. Lastly, poultry GIT disease studies could be enhanced with the use of 3D mini-guts to deepen our comprehension on the poultry gut to poultry health functional interplays.
Author contributions
C. E. Aruwa: Data processing and analysis; Investigation; Resources; Original draft writing, review & editing. S. Sabiu: Conceptualization; Supervision; Resources; Writing review & editing.
Acknowledgments
Authors acknowledge the support received in part from the National Research Foundation (NRF) of South Africa (Reference number PSTD2204133389) to C. E. Aruwa; the Directorate of Research and Postgraduate Support, Durban University of Technology (DUT); the South African Medical Research Council (SAMRC), NRF Research Development Grant for Rated Researchers (Grant No. 120433), and the Competitive Programme for Rated Researchers Support (SRUG2204193723) awarded to S. Sabiu.
Disclosure statement
No potential conflict of interest was reported by the author(s).
References
- Abaidullah, M., S. Peng, M. Kamran, X. Song, and Z. Yin. 2019. “Current Findings on Gut Microbiota Mediated Immune Modulation against Viral Diseases in Chicken.” Viruses 11 (8): 681. https://doi.org/10.3390/v11080681.
- Al Hakeem, W. G., K. Y. Acevedo Villanueva, and R. K. Selvaraj. 2023. “The Development of Gut Microbiota and its Changes Following C. Jejuni Infection in Broilers.” Vaccines 11 (3): 595. https://doi.org/10.3390/vaccines11030595.
- Altaher, Y. W., M. F. Jahromi, R. Ebrahim, I. Zulkifli, and J. B. Liang. 2015. “Lactobacillus Pentosus ITA23 and L. Acidipiscis ITA44 Enhance Feed Conversion Efficiency and Beneficial Gut Microbiota in Broiler Chickens.” Brazilian Journal of Poultry Science 17 (2): 159–164. https://doi.org/10.1590/1516-635x1702159-164.
- Angel, R. 2007. “Metabolic Disorders: Limitations to Growth of and Mineral Deposition into the Broiler Skeleton after Hatch and Potential Implications for Leg Problems.” Journal of Applied Poultry Research 16 (1): 138–149. https://doi.org/10.1093/japr/16.1.138.
- Apajalahti, J., A. Kettunen, and H. Graham. 2004. “Characteristics of the Gastrointestinal Microbial Communities, with Special Reference to the Chicken.” World’s Poultry Science Journal 60 (2): 223–232. https://doi.org/10.1079/WPS20040017.
- Arega, A. M. 2018. “Review on Infectious Bursal Disease: Threat for Ethiopian Poultry Industry.” International Journal of Advanced Life Sciences 11 (1): 52–65. https://doi.org/10.26627/IJALS/2018/11.01.0047.
- Aruwa, C. E. 2020. “Food Regulations and Governance.” In Food Science and Technology: Trends and Future Prospects, Part VI, Food Business: Entrepreneurship and Regulation, edited by O. A. Ijabadeniyi, 487–510. Germany: De Gruyter.
- Aruwa, C. E., C. Pillay, M. N. Nyaga, and S. Sabiu. 2021. “Poultry Gut Health – Microbiome Functions, Environmental Impacts, Microbiome Engineering and Advancements in Characterization Technologies.” Journal of Animal Science and Biotechnology 12 (1): 119. https://doi.org/10.1186/s40104-021-00640-9.
- Attoui, H., P. P. C. Mertens, J. Becnel, S. Belaganahalli, S. Bergoin, C. P. Brussaard, J. D. Chappell. 2012. “Reoviridae.” In Virus Taxonomy, edited by A. M. Q. King, M. J. Adams, E. B. Carstens, and E. Lefkowitz, 541–637. London, United Kingdom: Elsevier Academic Press.
- Awad, W. A., E. Mann, M. Dzieciol, C. Hess, S. Schmitz-esser, M. Wagner, and M. Hess. 2016. “Age-Related Differences in the Luminal and Mucosa-Associated Gut Microbiome of Broiler Chickens and Shifts Associated with Campylobacter Jejuni Infection.” Frontiers in Cellular and Infection Microbiology 6:154. https://doi.org/10.3389/fcimb.2016.00154.
- Ayginin, A. A., E. V. Pimkina, A. D. Matsvay, A. S. Speranskaya, M. V. Safonova, E. A. Blinova, I. V. Artyushin, V. G. Dedkov, G. A. Shipulin, and K. Khafizov. 2018. “The Study of Viral RNA Diversity in Bird Samples Using de Novo Designed Multiplex Genus-Specific Primer Panels.” Advances in Virology 2018:1–10. https://doi.org/10.1155/2018/3248285.
- Bailey, R. A. 2010. “Intestinal Microbiota and the Pathogenesis of Dysbacteriosis in Broiler Chickens.” PhD thesis, United Kingdom: University of East Anglia.
- Ballou, A. L., R. A. Ali, M. A. Mendoza, J. C. Ellis, H. M. Hassan, W. J. Croom, and M. D. Koci. 2015. “Development of the Chick Microbiome: How Early Exposure Influences Future Microbial Diversity.” Frontiers in Veterinary Science 3:2. https://doi.org/10.3389/fvets.2016.00002.
- Barnes, H. J., and J. S. Guy. 2003. “Poult Enteritis Mortality Syndrome.” In Diseases of Poultry, edited by Y. M. Saif, H. J. Barnes, A. Fadly, J. R. Glisson, L. R. McDougald, and D. E. Swayne, 1171–1180. Ames, Los Angeles, USA: Iowa State University Press.
- Barnes, H. J., J. S. Guy, and J. P. Vaillancourt. 2000. “Poult Enteritis Complex.” Revue Scientifique et Technique International Office of Epizootics 19 (2): 565–588. https://doi.org/10.20506/rst.19.2.1234.
- Barton, G. M. 2008. “A Calculated Response: Control of Inflammation by the Innate Immune System.” Journal of Clinical Investigation 118 (2): 413–420. https://doi.org/10.1172/JCI34431.
- Baurhoo, B., L. Phillip, and C. A. Ruiz-feria. 2007. “Effects of Purified Lignin and Mannan Oligosaccharides on Intestinal Integrity and Microbial Populations in the Ceca and Litter of Broiler Chickens.” Poultry Science 86 (6): 1070–1078. https://doi.org/10.1093/ps/86.6.1070.
- Belete, A., M. Addis, and M. Ayele. 2016. “Review on Major Gastrointestinal Parasites that Affect Chickens.” Journal of Biology, Agriculture and Healthcare 6:11–21. https://core.ac.uk/download/pdf/234662019.pdf.
- Bergner, L. M., R. J. Orton, A. da Silva Filipe, A. E. Shaw, D. J. Becker, C. Tello, R. Biek, and D. G. Streicker. 2019. “Using Noninvasive Metagenomics to Characterize Viral Communities from Wildlife.” Molecular Ecology Resources 19 (1): 128–143. https://doi.org/10.1111/1755-0998.12946.
- Bindari, Y. R., and P. F. Gerber. 2022. “Centennial Review: Factors Affecting the Chicken Gastrointestinal Microbial Composition and their Association with Gut Health and Productive Performance.” Poultry Science 101 (1): 101612. https://doi.org/10.1016/j.psj.2021.101612.
- Bindari, Y. R., R. J. Moore, T. T. H. van, M. Hilliar, S.-B. Wu, S. W. Walkden-brown, and P. F. Gerber. 2021. “Microbial Communities of Poultry House Dust, Excreta and Litter are Partially Representative of Microbiota of Chicken Caecum and Ileum.” PLoS One 16 (8): e0255633. https://doi.org/10.1371/journal.pone.0255633.
- Broom, L. J. 2017. “Necrotic Enteritis: Current Knowledge and Diet-Related Mitigation.” World’s Poultry Science Journal 73 (2): 281–292. https://doi.org/10.1017/S0043933917000058.
- Caesar, R., F. Backhed, and F. Bäckhed. 2010. “Effects of Gut Microbiota on Obesity and Atherosclerosis via Modulation of Inflammation and Lipid Metabolism.” Journal of Internal Medicine 268 (4): 320–328. https://doi.org/10.1111/j.1365-2796.2010.02270.x.
- Calvert, A. 2012. “Light Turkey Syndrome: Field Study and Inoculation Trial.” Master’s Dissertation, USA: University of Minnesota.
- Celi, P., A. J. Cowieson, F. Fru-nji, R. E. Steinert, A. M. Kluenter, and V. Verlhac. 2017. “Gastrointestinal Functionality in Animal Nutrition and Health: New Opportunities for Sustainable Animal Production.” Animal Feed Science and Technology 234:88–100. https://doi.org/10.1016/j.anifeedsci.2017.09.012.
- Chaney, W. E., S. A. Naqvi, M. Gutierrez, A. Gernat, T. J. Johnson, and D. Petry. 2022. “Dietary Inclusion of a Saccharomyces cerevisiae-Derived Postbiotic is Associated with Lower Salmonella enterica Burden in Broiler Chickens on a Commercial Farm in Honduras.” Microorganisms [Internet] 10 (3): 544. https://doi.org/10.3390/microorganisms10030544.
- Cheled-shoval, S. L., E. Amit-romach, M. Barbakov, and Z. Uni. 2011. “The Effect of In Ovo Administration of Mannan Oligosaccharide on Small Intestine Development During the Pre- and Posthatch Periods in Chickens.” Poultry Science 90 (10): 2301–2310. https://doi.org/10.3382/ps.2011-01488.
- Chen, Y.-N., C. C. Wu, and L. T. Lin. 2015. “Turkey Coronavirus: An Updated Review.” Taiwan Veterinary Journal 41 (1): 1–10. https://doi.org/10.1142/S1682648515300014.
- Chichlowski, M., W. J. Croom, F. W. Edens, B. W. McBride, R. Qiu, C. C. Chiang, L. R. Daniel, G. B. Havenstein, and M. D. Koci. 2007. “Microarchitecture and Spatial Relationship Between Bacteria and Ileal, Cecal and Colonic Epithelium in Chicks Fed a Direct-Fed Microbial, PrimaLac and Salinomycin.” Poultry Science 86 (6): 1121–1132. https://doi.org/10.1093/ps/86.6.1121.
- Choct, M. 2009. “Managing Gut Health Through Nutrition.” British Poultry Science 50 (1): 9–15. https://doi.org/10.1080/00071660802538632.
- Chow, J., and S. K. Mazmanian. 2010. “A Pathobionts of the Microbiota Balances Host Colonization and Intestinal Inflammation.” Cell Host & Microbe 7 (4): 265–276. https://doi.org/10.1016/j.chom.2010.03.004.
- Clark, E. L., S. E. Macdonald, V. Thenmozhi, K. Kundu, R. Garg, S. Kumar, S. Ayoade, et al. 2016. “Cryptic Eimeria Genotypes are Common Across the Southern but Not Northern Hemisphere.” International Journal for Parasitology 46 (9): 537–544. https://doi.org/10.1016/j.ijpara.2016.05.006.
- Clavijo, V., T. Morales, M. J. Vives‑flores, and A. R. Muñoz. 2022. “The Gut Microbiota of Chickens in a Commercial Farm Treated with a Salmonella Phage Cocktail.” Scientific Reports 12 (1): 991. https://doi.org/10.1038/s41598-021-04679-6.
- Cole, J. R., Jr, and F. M. Boyd. 1967. “Fat Absorption from the Small Intestine of Gnotobiotic Chicks.” Applied Microbiology 15 (5): 1229–1234. https://doi.org/10.1128/am.15.5.1229-1234.1967.
- Collier, C. T., C. L. Hofacre, A. M. Payne, D. B. Anderson, P. Kaiser, R. I. Mackie, and H. R. Gaskins. 2008. “Coccidia-Induced Mucogenesis Promotes the Onset of Necrotic Enteritis by Supporting Clostridium perfringens Growth.” Veterinary Immunology and Immunopathology 122 (1–2): 104–115. https://doi.org/10.1016/j.vetimm.2007.10.014.
- Cressman, M. D., Z. Yu, M. C. Nelson, S. J. Moeller, M. S. Lilburn, and H. N. Zerby. 2010. “Interrelations between the Microbiotas in the Litter and in the Intestines of Commercial Broiler Chickens.” Applied and Environmental Microbiology 76 (19): 6572–6582. https://doi.org/10.1128/AEM.00180-10.
- Dai, X., and H. Karring. 2014. “A Determination and Composition of Urease Activity in Feces and Fresh Manure from Pig and Cattle in Relation to Ammonia Production and pH Changes.” Public Library of Science ONE 9 (11): e110402. https://doi.org/10.1371/journal.pone.0110402.
- Daniel, N., E. Lecuyer, and B. Chassaing. 2021. “Host/Microbiota Interactions in Health and Diseases—Time for Mucosal Microbiology!” Mucosal Immunology 14 (5): 1006–1016. https://doi.org/10.1038/s41385-021-00383-w.
- Danzeisen, J. L., A. J. Calvert, S. L. Noll, B. McComb, J. S. Sherwood, C. M. Logue, and T. J. Johnson. 2013. “Succession of the Turkey Gastrointestinal Bacterial Microbiome Related to Weight Gain.” Peer Journal 1:e237. https://doi.org/10.7717/peerj.237.
- Day, J. M., L. L. Ballard, M. V. Duke, B. E. Scheffler, and L. Zsak. 2010. “Metagenomic Analysis of the Turkey Gut RNA Virus Community.” Virology Journal 7 (1): 313. https://doi.org/10.1186/1743-422X-7-313.
- Day, J. M., B. B. Oakley, B. S. Seal, and L. Zsak. 2015. “Comparative Analysis of the Intestinal Bacterial and RNA Viral Communities from Sentinel Birds Placed on Selected Broiler Chicken Farms.” PLOS ONE 10:e0117210. https://doi.org/10.1371/journal.pone.0117210.
- Dethlefsen, L., S. Huse, M. L. Sogin, and D. A. Relman. 2008. “The Pervasive Effects of an Antibiotic on the Human Gut Microbiota, As Revealed by Deep 16S rRNA Sequencing.” PLOS Biology 6 (11): e280. https://doi.org/10.1371/journal.pbio.0060280.
- Devaney, R., J. Trudgett, A. Trudgett, C. Meharg, and V. A. Smyth. 2016. “A Metagenomic Comparison of Endemic Viruses from Broiler Chickens with Runting Stunting Syndrome and from Normal Birds.” Avian Pathology 45 (6): 1–14. https://doi.org/10.1080/03079457.2016.1193123.
- Dhama, K., S. Chakraborty, A. K. Verma, R. Tiwari, R. Barathidasan, A. Kumar, and S. D. Singh. 2013. “Fungal/Mycotic Diseases of Poultry-Diagnosis, Treatment and Control: A Review.” Pakistan Journal of Biological Science 16 (23): 1626–1640. https://doi.org/10.3923/pjbs.2013.1626.1640.
- Diaz Carrasco, J. M. 2019. “Seasonality As a Driver of Cecal and Litter Microbiota Variation in Commercial Broiler Chickens.” In Proceedings of the Symposium on Gut Health in Production of Food Animals. St, 13. Louis, USA.
- Diaz Carrasco, J. M., N. A. Casanova, and M. E. Miyakawa. 2019. “Microbiota, Gut Health and Chicken Productivity: What Is the Connection?” Microorganisms [Internet] 7:374. https://doi.org/10.3390/microorganisms7100374.
- Dibner, J. J., and J. D. Richards. 2005. “Antibiotic Growth Promoters in Agriculture: History and Mode of Action.” Poultry Science 84 (4): 634–643. https://doi.org/10.1093/ps/84.4.634.
- Dietert, R. R., and E. K. Silbergeld. 2015. “Biomarkers for the 21st Century: Listening to the Microbiome.” Toxicology Science 144 (2): 208–216. https://doi.org/10.1093/toxsci/kfv013.
- Dinev, I. 2009. “Clinical and Morphological Investigations on the Prevalence of Lameness Associated with Femoral Head Necrosis in Broilers.” British Poultry Science 50 (3): 284–290. https://doi.org/10.1080/00071660902942783.
- Ding, J., R. Dai, L. Yang, C. He, K. Xu, S. Liu, W. Zhao, et al. 2017. “Inheritance and Establishment of Gut Microbiota in Chickens.” Frontiers in Microbiology 8:1967. https://doi.org/10.3389/fmicb.2017.01967.
- Doughman, E. 2023. “3D Miniguts Could Advance Chicken Gut Disease Research.” Retrieved on 01 August 2023 from https://www.wattagnet.com/poultry-future/article/15537525/3d-miniguts-could-advance-chicken-gut-disease-research.
- Ducatelle, R., E. Goossens, V. Eeckhaut, and F. van Immerseel. 2023. “Poultry Gut Health and Beyond.” Animal Nutrition 13:240–248. https://doi.org/10.1016/j.aninu.2023.03.005.
- Dunkley, K. D., C. S. Dunkley, N. L. Njongmeta, T. R. Callaway, M. E. Hume, L. F. Kubena, D. J. Nisbet, and S. C. Ricke. 2007. “Comparison of In Vitro Fermentation and Molecular Microbial Profiles of High-Fiber Feed Substrates Incubated with Chicken Cecal Inocula.” Poultry Science 86 (5): 801–810. https://doi.org/10.1093/ps/86.5.801.
- Dunn, J. R., and I. M. Gimeno. 2003. “Current Status of Marek’s Disease in the United States and Worldwide Based on a Questionnaire Survey.” Avian Disease 57 (2s1): 483–490. https://doi.org/10.1637/10373-091412-ResNote.1.
- Emami, N. K., A. Samie, H. R. Rahmani, and C. A. Ruiz-feria. 2012. “The Effect of Peppermint Essential Oil and Fructooligosaccharides, As Alternatives to Virginiamycin, on Growth Performance, Digestibility, Gut Morphology and Immune Response of Male Broilers.” Animal Feed Science and Technology 175:57–64. https://doi.org/10.1016/j.anifeedsci.2012.04.001.
- European Food Safety Authority (EFSA). 2011. “Scientific Opinion on Campylobacter in Broiler Meat Production: Control Options and Performance Objectives and/or Targets at Different Stages of the Food Chain.” EFSA Journal 9 (4): 2105. https://doi.org/10.2903/j.efsa.2011.2105.
- Farag, M. R., and M. Alagawany. 2018. “Physiological Alterations of Poultry to the High Environmental Temperature.” Journal of Thermal Biology 76:101–106. https://doi.org/10.1016/j.jtherbio.2018.07.012.
- Fathima, S., R. Shanmugasundaram, D. Adams, and R. K. Selvaraj. 2022. “Gastrointestinal Microbiota and Their Manipulation for Improved Growth and Performance in Chickens.” Foods 11 (10): 1401. https://doi.org/10.3390/foods11101401.
- Fernandez, F., M. Hinton, and B. van Gils. 2002. “Dietary Mannan-Oligosaccharides and Their Effect on Chicken Caecal Microflora in Relation to Salmonella Enteritidis Colonization.” Avian Pathology 31 (1): 49–58. https://doi.org/10.1080/03079450120106000.
- Fisinin, V. I., G. Yu Laptev, I. N. Nikonov, L. A. Il’Ina, E. A. Jyldyrym, V. A. Filippova, N. I. Novikova, et al. 2016. “Poultry Gastrointestinal Microbiome Changes During Ontogenesis.” Agricultural Biology 51 (6): 883–890. https://doi.org/10.15389/agrobiology.2016.6.883eng.
- Fregolente, M. C., and M. S. Gatti. 2009. “Nomenclature Proposal for Picobirnavirus.” Archives in Virology 154 (12): 1953–1954. https://doi.org/10.1007/s00705-009-0537-z.
- Ganner, A., and G. Schatzmayr. 2012. “Capability of Yeast Derivatives to Adhere Enteropathogenic Bacteria and to Modulate Cells of the Innate Immune System.” Applied Microbiology and Biotechnology 95 (2): 289–297. https://doi.org/10.1007/s00253-012-4140-y.
- Göransson, L., S. Abeyesinghe, J. Yngvesson, and S. Gunnarsson. 2023. “How are they Really Doing? Animal Welfare on Organic Laying Hen Farms in Terms of Health and Behaviour.” British Poultry Science 64 (5): 552–564. https://doi.org/10.1080/00071668.2023.2241829.
- Gowda, S. 2023. “Modern Innovations in Poultry Farming. SR Publications 2024.” Accessed January 26, 2024. https://www.srpublication.com/modern-innovations-in-poultry-farming/.
- Hai, Y., T. Zhou, J. Gong, C. Young, X. Su, X. Z. Li, H. Zhu, R. Tsao, and R. Yang. 2010. “Isolation of Deoxynivalenol-Transforming Bacteria from the Chicken Intestines Using the Approach of PCR-DGGE Guided Microbial Selection.” BMC Microbiology 10 (1): 182. https://doi.org/10.1186/1471-2180-10-182.
- Hauck, R. 2017. “Interactions between Parasites and the Bacterial Microbiota of Chickens.” Avian Disease 61 (4): 428–436. https://doi.org/10.1637/11675-051917-Review.1.
- Henao-mejia, J., E. Elinav, T. Strowig, and R. A. Flavell. 2012. “Inflammasomes: Far Beyond Inflammation.” Nature Immunology 13 (4): 321–324. https://doi.org/10.1038/ni.2257.
- Heo, J. M., F. O. Opapeju, J. R. Pluske, J. C. Kim, D. J. Hampson, and C. M. Nyachoti. 2013. “Gastrointestinal Health and Function in Weaned Pigs: A Review of Feeding Strategies to Control Post-Weaning Diarrhoea without Using In-Feed Antimicrobial Compounds.” Journal of Animal Physiology and Animal Nutrition 97:207–237. https://doi.org/10.1111/j.1439-0396.2012.01284.x.
- Hidaka, H., and M. Hirayama. 1991. “Useful Characteristics and Commercial Applications of Fructo-Oligosaccharides.” Biochemical Society Transactions 19 (3): 561–565. https://doi.org/10.1042/bst0190561.
- Hotamisligil, G. S. 2006. “Inflammation and Metabolic Disorders.” Nature 444 (7121): 860–867. https://doi.org/10.1038/nature05485.
- Hotamisligil, G. S., and E. Erbay. 2008. “Nutrient Sensing and Inflammation in Metabolic Diseases.” Nature Reviews Immunology 8 (12): 923–934. https://doi.org/10.1038/nri2449.
- Ivanov, I. I., R. L. Frutos, N. Manel, K. Yoshinaga, D. B. Rufkin, R. B. Sartor, B. B. Finlay, and D. R. Littman. 2008. “Specific Microbiota Direct the Differentiation of IL-17-producing T-Helper Cells in the Mucosa of the Small Intestine.” Cell Host & Microbe 4 (4): 337–349. https://doi.org/10.1016/j.chom.2008.09.009.
- Jackwood, D. J. 2017. “Advances in Vaccine Research Against Economically Important Viral Diseases of Food Animals: Infectious Bursal Disease Virus.” The Veterinary Journal 206:121–125. https://doi.org/10.1016/j.vetmic.2016.11.022.
- Jackwood, M. W., T. O. Boynton, D. A. Hilt, E. T. McKinley, J. C. Kissinger, A. H. Paterson, J. Robertson, et al. 2010. “Emergence of a Group 3 Coronavirus through Recombination.” Virology 398 (1): 98–108. https://doi.org/10.1016/j.virol.2009.11.044.
- Jindal, N., D. P. Patnayak, A. F. Ziegler, A. Lago, and S. M. Goyal. 2009. “A Retrospective Study on Poult Enteritis Syndrome in Minnesota.” Avian Disease 53 (2): 268–275. https://doi.org/10.1637/8513-110308-Reg.1.
- Jin, L. Z., Y. W. Ho, N. Abdullah, and S. Jalaludin. 1998. “Growth Performance, Intestinal Microbial Populations, and Serum Cholesterol of Broilers Fed Diets Containing Lactobacillus Cultures.” Poultry Science 77 (9): 1259–1265. https://doi.org/10.1093/ps/77.9.1259.
- Julian, R. J. 2005. “Production and Growth Related Disorders and Other Metabolic Diseases of Poultry – a Review.” The Veterinary Journal 169 (3): 350–369. https://doi.org/10.1016/j.tvjl.2004.04.015.
- Jumpertz, R., D. S. le, P. J. Turnbaugh, C. Trinidad, C. Bogardus, J. I. Gordon, and J. Krakoff. 2011. “Energy-Balance Studies Reveal Associations Between Gut Microbes, Caloric Load and Nutrient Absorption in Humans.” The American Journal of Clinical Nutrition 94 (1): 58–65. https://doi.org/10.3945/ajcn.110.010132.
- Jurburg, S. D., M. S. M. Brouwer, D. Ceccarelli, J. van der Goot, A. J. M. Jansman, and A. Bossers. 2019. “Patterns of Community Assembly in the Developing Chicken Microbiome Reveal Rapid Primary Succession.” MicrobiologyOpen 8 (9): e00821. https://doi.org/10.1002/mbo3.821.
- Kang, K., Y. Hu, S. Wu, and S. Shi. 2021. “Comparative Metagenomic Analysis of Chicken Gut Microbial Community, Function and Resistome to Evaluate Noninvasive and Cecal Sampling Resources.” Animal 11 (6): 1718. https://doi.org/10.3390/ani11061718.
- Keerqin, C., L. Rhayat, Z.-H. Zhang, K. Gharib-naseri, S. Kheravii, E. Devillard, T. Crowley, and S. B. Wu. 2021. “Probiotic Bacillus subtilis 29,784 Improved Weight Gain and Enhanced Gut Health Status of Broilers Under Necrotic Enteritis Condition.” Poultry Science 100:100981. https://doi.org/10.1016/j.psj.2021.01.004.
- Kers, J. G., F. C. Velkers, E. A. Fischer, G. D. Hermes, D. M. Lamot, J. A. Stegeman, and H. Smidt. 2019. “Take Care of the Environment: Housing Conditions Affect the Interplay of Nutritional Interventions and Intestinal Microbiota in Broiler Chickens.” Animal Microbiome 1 (1): 10. https://doi.org/10.1186/s42523-019-0009-z.
- Kim, H. J., S. J. Eom, S. J. Park, C. J. Cha, and G. B. Kim. 2011. “Lactobacillus alvi sp. Nov. Isolated from the Intestinal Tract of Chicken.” FEMS Microbiology Letters 323:83–87. https://doi.org/10.1111/j.1574-6968.2011.02361.x.
- Kim, W. H., and H. S. Lillehoj. 2019. “Immunity, Immunomodulation and Antibiotic Alternatives to Maximize the Genetic Potential of Poultry for Growth and Disease Response.” Animal Feed Science and Technology 250:41–50. https://doi.org/10.1016/j.anifeedsci.2018.09.016.
- Kim, E., N. K. Morgan, A. F. Moss, L. Li, P. Ader, and M. Choct. 2022. “The Flow of Non-Starch Polysaccharides along the Gastrointestinal Tract of Broiler Chickens Fed either a Wheat- or Maize-Based Diet.” Animal Nutrition 9:138–142. https://doi.org/10.1016/j.aninu.2021.11.004.
- Kipanyula, M. J., P. F. S. Etet, L. Vecchio, M. Farahna, E. N. Nukenine, and A. H. N. Kamdje. 2013. “Signalling Pathways Bridging Microbial-Triggered Inflammation and Cancer.” Cellular Signalling 25 (2): 403–416. https://doi.org/10.1016/j.cellsig.2012.10.014.
- Knarreborg, A., M. A. Simon, R. M. Engberg, B. B. Jensen, and G. W. Tannock. 2002. “Effects of Dietary Fat Source and Subtherapeutic Levels of Antibiotic on the Bacterial Community in the Ileum of Broiler Chickens at Various Ages.” Applied and Environmental Microbiology 68 (12): 5918–5924. https://doi.org/10.1128/AEM.68.12.5918-5924.2002.
- Koch, S., and N. Asma. 2012. “The Life and Death of Epithelia During Inflammation: Lessons Learned from the Gut.” Annual Reviews in Pathology 7 (1): 35–60. https://doi.org/10.1146/annurev-pathol-011811-120905.
- Kogut, M. H. 2013. “The Gut Microbiota and Host Innate Immunity: Regulators of Host Metabolism and Metabolic Diseases in Poultry?” Journal of Applied Poultry Research 22 (3): 637–646. https://doi.org/10.3382/japr.2013-00741.
- Kogut, M. H. 2019. “The Effect of Microbiome Modulation on the Intestinal Health of Poultry.” Animal Feed Science and Technology 250:32–40. https://doi.org/10.1016/j.anifeedsci.2018.10.008.
- Kogut, M. H. In Avian Immunology Shat, T., Vervelde, L., Kaspers, B., edited by. 2021. Impact of the Gut Microbiota on the Immune System, 353–364. Amsterdam, The Netherlands: Elsevier Publishing.
- Kogut, M. H. 2022. “Role of Diet-Microbiota Interactions in Precision Nutrition of the Chicken: Facts, Gaps and New Concepts.” Poultry Science 101 (3): 101673. https://doi.org/10.1016/j.psj.2021.101673.
- Kohl, K. D. 2012. “Diversity and Function of the Avian Gut Microbiota.” Journal of Comparative Physiology B: Biochemical, Systemic and Environmental Physiology 182 (5): 591–602. https://doi.org/10.1007/s00360-012-0645-z.
- Kubasova, T., M. Kollarcikova, M. Crhanova, D. Karasova, D. Cejkova, A. Sebkova, J. Matiasovicova, et al. 2019. “Contact with Adult Hen Affects Development of Caecal Microbiota in Newly Hatched Chicks.” Public Library of Science ONE 14 (3): e0212446. https://doi.org/10.1371/journal.pone.0212446.
- Kumar, S., C. Chen, N. Indugu, G. O. Werlang, M. Singh, W. K. Kim, and H. Thippareddi. 2018. “Effect of Antibiotic Withdrawal in Feed on Chicken Gut Microbial Dynamics, Immunity, Growth Performance and Prevalence of Foodborne Pathogens.” PLoS One 13 (2): e0192450. https://doi.org/10.1371/journal.pone.0192450.
- Landim de Barros, T., C. N. Vuong, G. Tellez-isaias, and B. M. Hargis. 2022. “Uncontroversial Facts and New Perspectives on Poultry Histomonosis: A Review.” World’s Poultry Science Journal 78:913–933. https://doi.org/10.1080/00439339.2022.2119915.
- Lan, P. T., M. Sakamoto, and Y. Benno. 2004. “Effects of Two Probiotic Lactobacillus Strains on Jejunal and Cecal Microbiota of Broiler Chicken Under Acute Heat Stress Condition As Revealed by Molecular Analysis of 16S rRNA Genes.” Microbiology and Immunology 48 (12): 917–929. https://doi.org/10.1111/j.1348-0421.2004.tb03620.x.
- Lavelle, E. C., C. Murphy, L. A. J. o’neill, and E. M. Creagh. 2010. “The Role of TLRs, NLRs and RLRs in Mucosal Innate Immunity and Homeostasis.” Mucosal Immunology 3 (1): 17–28. https://doi.org/10.1038/mi.2009.124.
- LeBlanc, J. G., C. Milani, G. S. de Giori, F. Sesma, D. van Sinderen, and M. Ventura. 2013. “Bacteria as Vitamin Suppliers to their Host: A Gut Microbiota Perspective.” Current Opinion in Biotechnology 24 (2): 160–168. https://doi.org/10.1016/j.copbio.2012.08.005.
- Lee, M. D. 2008. “Managing Disease Resistance: Applying Advanced Methods to Understand Gastrointestinal Microbial Communities.” In Gut Efficiency, the Key Ingredient in Pig and Poultry Production, edited by J. A. Taylor-Pickard and P. Spring, 109–124. Wageningen, the Netherlands: Wageningen Academic Publishers.
- Lee, S. H., S. Bang, H. H. Jang, E. B. Lee, B. S. Kim, S. H. Kim, S. H. Kang, et al. 2020. “Effects of Allium Hookeri on Gut Microbiome Related to Growth Performance in Young Broiler Chickens.” PLoS One 15 (1): e0226833. https://doi.org/10.1371/journal.pone.0226833.
- Lee, S., T. M. la, H. Lee, I. S. Choi, C. S. Song, S. Y. Park, J. B. Lee, et al. 2019. “Characterization of Microbial Communities in the Chicken Oviduct and the Origin of Chicken Embryo Gut Microbiota.” Scientific Report 9 (1): 6838. https://doi.org/10.1038/s41598-019-43280-w.
- Leung, J. M., A. L. Graham, and S. C. L. Knowles. 2018. “Parasite-Microbiota Interactions with the Vertebrate Gut: Synthesis Through an Ecological Lens.” Frontiers in Microbiology 9:843. https://doi.org/10.3389/fmicb.2018.00843.
- Liljebjelke, K., C. Hofacre, L. Tongrui, and J. Maurer. 2003. “Molecular Epidemiology of Salmonella on Poultry Farms in NE Georgia.” In Program and Abstracts, Congress of the World Veterinary Poultry Association. World Veterinary Poultry Association, 19–23. Denver: World Veterinary Poultry Association, Denver, CO.
- Lima, D. A., S. P. Cibulski, F. Finkler, T. F. Teixeira, A. P. M. Varela, C. Cerva, M. R. Loiko, et al. 2017. “Faecal Virome of Healthy Chickens Reveals a Large Diversity of the Eukaryote Viral Community, Including Novel Circular SsDNA Viruses.” Journal of General Virology 98 (4): 690–703. https://doi.org/10.1099/jgv.0.000711.
- MacDonald, S. E., M. J. Nolan, K. Harman, K. Boulton, D. A. Hume, F. M. Tomley, R. A. Stabler, and D. P. Blake. 2017. “Effects of Eimeria Tenella Infection on Chicken Caecal Microbiome Diversity, Exploring Variation Associated with Severity of Pathology.” PLOS ONE 12 (9): e0184890. https://doi.org/10.1371/journal.pone.0184890.
- MacDonald, S. E., P. M. van Diemen, H. Martineau, M. P. Stevens, F. M. Tomley, R. A. Stabler, and D. P. Blake. 2019. “Impact of Eimeria Tenella Coinfection on Campylobacter Jejuni Colonization of the Chicken.” Infection and Immunity 87 (2): e00772–18. https://doi.org/10.1128/IAI.00772-18.
- Mackenzie, J. S., and M. Jeggo. 2013. “Reservoirs and Vectors of Emerging Viruses.” Current Opinions in Virology 3 (2): 170–179. https://doi.org/10.1016/j.coviro.2013.02.002.
- Maes, P., G. K. Amarasinghe, M. A. ayllón, C. F. Basler, S. Bavari, K. R. Blasdell, T. Briese, et al. 2019. “Taxonomy of the Order Mononegavirales: Second Update 2018.” Archives in Virology 164 (4): 1233–1244. https://doi.org/10.1007/s00705-018-04126-4.
- Mateos, G. G., E. Jiménez-moreno, M. P. Serrano, and R. P. Lázaro. 2012. “Poultry Response to High Levels of Dietary Fibre Sources Varying in Physical and Chemical Characteristics.” Journal of Applied Poultry Research 21 (1): 156–174. https://doi.org/10.3382/japr.2011-00477.
- Matthijs, M. G., J. H. van Eck, W. J. Landman, and J. A. Stegeman. 2003. “Ability of Massachusetts-Type Infectious Bronchitis Virus to Increase Colibacillosis Susceptibility in Commercial Broilers: A Comparison Between Vaccine and Virulent Field Virus.” Avian Pathology 32 (5): 473–481. https://doi.org/10.1080/0307945031000154062.
- Maxxperformance. 2023. “The Power of Stabilized Enzymes in Poultry Feed.” Retrieved on 27 July 2023 from https://www.maxxperformance.com/stories/animal-feed/the-powerof-stabilized-enzymes-in-poultryfeed/.
- Medvecky, M., D. Cejkova, O. Polansky, D. Karasova, T. Kubasova, A. Cizek, and I. Rychlik. 2018. “Whole Genome Sequencing and Function Prediction of 133 Gut Anaerobes Isolated from Chicken Caecum in Pure Cultures.” BMC Genomics 19:561. https://doi.org/10.1186/s12864-018-4959-4.
- Morishita, Y., and T. Mitsuoka. 1976. “Microorganisms Responsible for Controlling the Populations of Escherichia coli and Enterococcus and the Consistency of Cecal Contents in the Chicken.” Japanese Journal of Microbiology 20 (3): 197–202. https://doi.org/10.1111/j.1348-0421.1976.tb00975.x.
- Morrow, J. L., M. Frommer, D. C. A. Shearman, and M. Riegler. 2015. “The Microbiome of Field-Caught and Laboratory-Adapted Australian Tephritid Fruit Fly Species with Different Host Plant Use and Specialisation.” Microbial Ecology 70 (2): 498–508. https://doi.org/10.1007/s00248-015-0571-1.
- Mor, S. K., T. A. Sharafeldin, R. E. Porter, A. Ziegler, D. P. Patnayak, and S. M. Goyal. 2013. “Isolation and Characterization of a Turkey Arthritis Reovirus.” Avian Disease 57 (1): 97–103. https://doi.org/10.1637/10353-090712-Reg.1.
- Moussavi, A. R. H., R. O. Gilbert, T. R. Overton, D. E. Bauman, and W. R. Butler. 2007. “Effects of Feeding Fish Meal and N-3 Fatty Acids on Milk Yield and Metabolic Responses in Early Lactating Dairy Cows.” Journal of Dairy Science 90 (1): 136–144. https://doi.org/10.3168/jds.S0022-0302(07)72615-2.
- Mughini-gras, L., G. di Martino, L. Moscati, F. Buniolo, V. Cibin, and L. Bonfanti. 2020. “Natural Immunity in Conventionally and Organically Reared Turkeys and its Relation with Antimicrobial Resistance.” Poultry Science 99 (2): 763–771. https://doi.org/10.1016/j.psj.2019.10.027.
- Muramatsu, T., S. Nakajima, and J. Okumura. 1994. “Modification of Energy Metabolism by the Presence of the Gut Microflora in the Chicken.” British Journal of Nutrition 71 (5): 709–717. https://doi.org/10.1079/BJN19940178.
- Mwangi, W. N., R. K. Beal, C. Powers, X. Wu, T. Humphrey, M. Watson, M. Bailey, et al. 2010. “Regional and Global Changes in TCRαβ T Cell Repertoires in the Gut are Dependent Upon the Complexity of the Enteric Microflora.” Developmental and Comparative Immunology 34 (4): 406–417. https://doi.org/10.1016/j.dci.2009.11.009.
- Mwato, M. 2023. “Nutritional and Metabolic Diseases in Poultry.” Accessed August 11, 2023 from https://bivatec.com/blog/nutritional-and-metabolic-diseases-in-poultry.
- Nemati, Z., H. Janmohammadi, A. Taghizadeh, G. H. Moghaddam, and H. Maleki Nejad. 2014. “Effect of Bentonite Supplementation to the Contaminated Diets with Aflatoxin B1 on Broiler Performance.” In The 6th Iranian Congress on Animal Science, 27–28. Tabriz, Iran.
- Newell, D. G., K. T. Elvers, D. Dopfer, I. Hansson, P. Jones, S. James, J. Gittins, et al. 2011. “Biosecurity-Based Interventions and Strategies to Reduce Campylobacter Spp. on Poultry Farms.” Applied and Environmental Microbiology 77 (24): 8605–8614. https://doi.org/10.1128/AEM.01090-10.
- Ngunjiri, J. M., K. J. M. Taylor, M. C. Abundo, H. Jang, M. Elaish, K. C. Mahesh, A. Ghorbani, et al. 2019. ““Farm Stage, Bird Age and Body Site Dominantly Affect the Quantity, Taxonomic Composition and Dynamics of Respiratory and Gut Microbiota of Commercial Layer Chickens.” Applied Environmental Microbiology 85 (9): e03137–18. https://doi.org/10.1128/AEM.03137-18.
- Nicholson, J. K., E. Holmes, J. Kinross, R. Burcelin, G. Gibson, W. Jia, and S. Pettersson. 2012. “Host-Gut Microbiota Metabolic Interactions.” Science 336 (6086): 1262–1267. https://doi.org/10.1126/science.1223813.
- Niewold, T. A. 2007. “The Nonantibiotic Anti-Inflammatory Effect of Antimicrobial Growth Promoters, the Real Mode of Action? A Hypothesis.” Poultry Science 86 (4): 605–609. https://doi.org/10.1093/ps/86.4.605.
- The Nutrition Source. 2023. “The Microbiome.” Retrieved on 12 July 2023 from https://www.hsph.harvard.edu/nutritionsource/microbiome/.
- Oakley, B. B., and M. H. Kogut. 2016. “Spatial and Temporal Changes in the Broiler Chicken Cecal and Fecal Microbiomes and Correlations of Bacterial Taxa with Cytokine Gene Expression.” Frontiers in Veterinary Science 3:11. https://doi.org/10.3389/fvets.2016.00011.
- Oakley, B. B., H. S. Lillehoj, M. H. Kogut, W. K. Kim, J. J. Maurer, A. Pedroso, M. D. Lee, et al. 2014. “The Chicken Gastrointestinal Microbiome.” FEMS Microbiology Letters 360 (2): 100–112. https://doi.org/10.1111/1574-6968.12608.
- Oakley, B. B., C. A. Morales, J. Line, M. E. Berrang, R. J. Meinersmann, G. E. Tillman, M. G. Wise, et al. 2013. “The Poultry-Associated Microbiome: Network Analysis and Farm-To-Fork Characterizations.” PLOS One 8 (2): e57190. https://doi.org/10.1371/journal.pone.0057190.
- Oakley, B. B., E. J. R. Vasconcelos, P. P. V. P. Diniz, K. N. Calloway, E. Richardson, R. J. Meinersmann, N. A. Cox, and M. E. Berrang. 2018. “The Cecal Microbiome of Commercial Broiler Chickens Varies Significantly by Season.” Poultry Science 10 (10): 3635–3644. https://doi.org/10.3382/ps/pey214.
- O’hara, A. M., and F. Shanahan. 2006. “The Gut Flora as a Forgotten Organ.” EMBO Report 7 (7): 688–693. https://doi.org/10.1038/sj.embor.7400731.
- Organization for Economic Co-operation and Development/Food and Agricultural Organization. 2017. OECD-FAO Agricultural Outlook 2017-2026, OECD. Paris, France.
- Pantin-jackwood, M. J. 2013. “Multicausal Enteric Diseases.” In Diseases of Poultry, edited by D. E. Swayne, 1322–1325. New Jersey: Wiley-Blackwell.
- Pantin-jackwood, M., J. M. Day, M. W. Jackwood, and E. Spackman. 2008. “Enteric Viruses Detected by Molecular Methods in Commercial Chicken and Turkey Flocks in the United States Between 2005 and 2006.” Avian Disease 52 (2): 235–244. https://doi.org/10.1637/8174-111507-Reg.1.
- Pan, D., and Z. Yu. 2014. “Intestinal Microbiome of Poultry and Its Interaction with Host and Diet.” Gut Microbes 5 (1): 108–119. https://doi.org/10.4161/gmic.26945.
- Patel, J., and M. Skłodowska. 2023. “The Delicate Balance.” Retrieved on 26 September 2023 from https://www.teagasc.ie/news–events/daily/pigs–poultry/a-delicate-balance.php.
- Pedroso, A. A., A. L. Hurley-bacon, A. S. Zedek, T. W. Kwan, A. P. Jordan, G. Avellaneda, C. L. Hofacre, et al. 2013. “Can Probiotics Improve the Environmental Microbiome and Resistome of Commercial Poultry Production?” International Journal of Environmental Research and Public Health 10 (10): 4534–4559. https://doi.org/10.3390/ijerph10104534.
- Pender, C. M., S. Kim, T. D. Potter, M. M. Ritszi, M. Young, and R. A. Dalloul. 2016. “In Ovo Supplementation of Probiotics and Its Effects on Performance and Immune-Related Gene Expression in Broiler Chicks.” Poultry Science 96 (5): 1052–1062. https://doi.org/10.3382/ps/pew381.
- Petersen, C., and J. L. Round. 2014. “Defining Dysbiosis and Its Influence on Host Immunity and Disease.” Cellular Microbiology 16 (7): 1024–1033. https://doi.org/10.1111/cmi.12308.
- Pin Viso, N., E. Redondo, J. M. Dı´az Carrasco, L. Redondo, J. S. Garcia, M. F. Miyakawa, M. D. Farber, and L. D. Alcaraz. 2021. “Geography as Non-Genetic Modulation Factor of Chicken Cecal Microbiota.” Public Library of Science ONE 16 (1): e0244724. https://doi.org/10.1371/journal.pone.0244724.
- Polansky, O., Z. Sekelova, M. Faldynova, A. Sbkova, F. Sisak, and F. Rychik. 2016. “Important Metabolic Pathways and Biological Processes Expressed by Chicken Cecal Microbiota.” Applied environmental microbiology 82 (5): 1568–1576. https://doi.org/10.1128/AEM.03473-15.
- Poloni, V., A. Magnoli, A. Fochesato, A. Cristofolini, M. Caverzan, C. Merkis, M. Montenegro, et al. 2020. “A Saccharomyces cerevisiae RC016-Based Feed Additive Reduces Liver Toxicity, Residual Aflatoxin B1 Levels and Positively Influences Intestinal Morphology in Broiler Chickens Fed Chronic Aflatoxin B1-Contaminated Diets.” Animal Nutrition 6 (1): 31–38. https://doi.org/10.1016/j.aninu.2019.11.006.
- Qureshi, M. A., M. Yu, and Y. M. Saif. 2000. “A Novel ‘Small Round virus’ Inducing Poult Enteritis and Mortality Syndrome and Associated Immune Alterations.” Avian Disease 44 (2): 275–283. https://doi.org/10.2307/1592540.
- Rehman, H., P. Hellweg, D. Taras, and J. Zentek. 2008. “Effects of Dietary Inuin on the Intestinal Short Chain Fatty Acids and Microbial Ecology in Broiler Chickens As Revealed by Denaturing Gradient Gel Electrophoresis.” Poultry Science 87 (4): 783–789. https://doi.org/10.3382/ps.2007-00271.
- Rehman, H. U., W. Vahjen, W. A. Awad, and J. Zentek. 2007. “Indigenous Bacteria and Bacterial Metabolic Products in the Gastrointestinal Tract of Broiler Chickens.” Archives of Animal Nutrition 61:319–335. https://doi.org/10.1080/17450390701556817.
- Richards, P. J., G. M. Flaujac Lafontaine, P. L. Connerton, L. Liang, K. Asiani, N. M. Fish, and I. F. Connerton. 2020. “Galacto-Oligosaccharides Modulate the Juvenile Gut Microbiome and Innate Immunity to Improve Broiler Chicken Performance.” mSystems [Internet] 5 (1): e00827–19. https://doi.org/10.1128/mSystems.00827-19.
- Ricke, S. C. 2003. “Perspectives on the Use of Organic Acids and Short Chain Fatty Acids as Antimicrobials.” Poultry Science 82 (4): 632–639. https://doi.org/10.1093/ps/82.4.632.
- Ricke, S. C. 2015. “Potential of Fructooligosaccharide Prebiotics in Alternative and Nonconventional Poultry Production Systems.” Poultry Science 94 (6): 1411–1418. https://doi.org/10.3382/ps/pev049.
- Rinttilä, T., and J. Apajalahti. 2013. “Intestinal Microbiota and Metabolites—Implications for Broiler Chicken Health and Performance.” Journal of Applied Poultry Research 22 (3): 647–658. https://doi.org/10.3382/japr.2013-00742.
- Rittenberry, R. 2011. “Farm-To-Fork Safety. The New Federal Food Safety Law Aims to Prevent Contamination rather than Simply Respond to it.” Occupational Health and Safety 80 (6): 66–68.
- Roberts, T., J. Wilson, A. Guthrie, K. Cookson, D. Vancraenest, J. Schaeffer, R. Moody, and S. Clark. 2015. “New Issues and Science in Broiler Chicken Intestinal Health: Emerging Technology and Alternative Interventions.” Journal of Applied Poultry Research 24 (2): 257–266. https://doi.org/10.3382/japr/pfv023.
- Roussan, D. A., I. A. Shaheen, G. Y. Khawaldeh, W. S. Totanji, and R. H. al-rifai. 2012. “Simultaneous Detection of Astrovirus, Rotavirus, Reovirus Adenovirus Type 1 in Broiler Chicken Flocks.” Polish Journal of Veterinary Science 15 (2): 337–334. https://doi.org/10.2478/v10181-012-0052-0.
- Rubio, L. A. 2019. “Possibilities of Early Life Programming in Broiler Chickens via Intestinal Microbiota Modulation.” Poultry Science 98 (2): 695–706. https://doi.org/10.3382/ps/pey416.
- Schokker, D., B. de Klerk, R. Borg, A. Bossers, and J. M. J. Rebel. 2021. “Factors Influencing the Succession of the Fecal Microbiome in Broilers.” Livestock Science 247:104486. https://doi.org/10.1016/j.livsci.2021.104486.
- Sekirov, I., S. L. Russell, L. C. Antunes, and B. B. Finlay. 2010. “Gut Microbiota in Health and Disease.” Physical Review 90 (3): 859–904. https://doi.org/10.1152/physrev.00045.2009.
- Sergeant, M. J., C. Constantinidou, T. A. Cogan, M. R. Bedford, C. W. Penn, and M. J. Pallen. 2014. “Extensive Microbial and Functional Diversity within the Chicken Caecal Microbiome.” PLoS One 9 (3): e91941. https://doi.org/10.1371/journal.pone.0091941.
- Shang, Y., S. Kumar, B. Oakley, and K. K. Kim. 2018. “Chicken Gut Microbiota: Importance and Detection Technology.” Frontiers in Veterinary Science 5:254. https://doi.org/10.3389/fvets.2018.00254.
- Shirley, M. W., A. L. Smith, and F. M. Tomley. 2005. “The Biology of Avian Eimeria with an Emphasis on their Control by Vaccination.” Advances in Parasitology 60:285–330. https://doi.org/10.1016/S0065-308X(05)60005-X.
- Sizova, Е., S. Miroshnikov, S. Lebedev, B. Usha, and S. Shabunin. 2020. “Use of Nanoscale Metals in Poultry Diet as a Mineral Feed Additive.” Animal Nutrition 6 (2): 185–191. https://doi.org/10.1016/j.aninu.2019.11.007.
- Sommer, F., and F. Bäckhed. 2013. “The Gut Microbiota — Masters of Host Development and Physiology.” Nature Reviews in Microbiology 11 (4): 227–238. https://doi.org/10.1038/nrmicro2974.
- Songserm, T., J. M. Pol, D. J. van Roozelaar, G. L. Kok, F. Wagenaar, and A. A. Ter Huurne. 2000. “A Comparative Study of the Pathogenesis of Malabsorption Syndrome in Broilers.” Avian Disease 44 (3): 556–567. https://doi.org/10.2307/1593095.
- Soundararajan, S., J. Selvakumar, Z. M. M. Joseph, Y. Gopinath, V. Saravanan, and R. Santhanam. 2023. “Investigating the Modulatory Effects of Moringa Oleifera on the Gut Microbiota of Chicken Model Through Metagenomic Approach.” Frontiers in Veterinary Science 10:1153769. https://doi.org/10.3389/fvets.2023.1153769.
- Stanley, D., R. J. Hughes, and R. J. Moore. 2014. “Microbiota of the Chicken Gastrointestinal Tract: Influence on Health, Productivity and Disease.” Applied Microbiology and Biotechnology 98 (10): 4301–4310. https://doi.org/10.1007/s00253-014-5646-2.
- Stecher, B., R. Robbiani, A. W. Walker, A. M. Westendorf, M. Barthel, M. Kremer, A. J. Macperson, et al. 2007. “Salmonella enterica Serovar Typhimurium Exploits Inflammation to Compete with the Intestinal Microbiota.” PLOS Biology 5 (10): e244. https://doi.org/10.1371/journal.pbio.0050244.
- Sugiharto, S. 2019. “A Review of Filamentous Fungi in Broiler Production.” Annals of Agricultural Sciences 64 (1): 1–8. https://doi.org/10.1016/j.aoas.2019.05.005.
- Sun, B., L. Hou, and Y. Yang. 2021. “The Development of the Gut Microbiota and Short-Chain Fatty Acids of Layer Chickens in Different Growth Periods.” Frontiers in Veterinary Science 8:666535. https://doi.org/10.3389/fvets.2021.666535.
- Tan, Z., L. Luo, X. Wang, Q. Wen, L. Zhou, and K. Wu. 2019. “Characterization of the Caecal Microbiome Composition of Wenchang Chickens Before and After Fattening.” PLOS One 14 (12): e0225692. https://doi.org/10.1371/journal.pone.0225692.
- Tolin, S. A., and H. Domermuth. 1975. “Enteritis of Turkeys: Electron Microscopy of the Causal Virus.” Avian Disease 19 (1): 118–125. https://doi.org/10.2307/1588962.
- Torok, V. A., R. J. Hughes, L. L. Mikkelsen, R. Perez-maldonado, K. Balding, R. MacAlpine, N. J. Percy, and K. Ophela-keller. 2011. “Identification and Characterization of Potential Performance-Related Gut Microbiotas in Broiler Chickens Across Various Feeding Trials.” Applied Environmental Microbiology 77 (17): 5868–5878. https://doi.org/10.1128/AEM.00165-11.
- Turnbaugh, P. J., M. Hamady, T. Yatsunenko, B. L. Cantarel, A. Duncan, R. E. Ley, M. L. Sogin, et al. 2008. “A Core Gut Microbiome in Obese and Lean Twins.” Nature 457 (7228): 480–484. https://doi.org/10.1038/nature07540.
- Velthof, G. L., Y. Hou, and O. Oenema. 2015. “Nitrogen Excretion Factors of Livestock in the European Union: A Review.” Journal of the Science of Food and Agriculture 95 (15): 3004–3014. https://doi.org/10.1002/jsfa.7248.
- Vera, R., L. Arosemena, and M. L. Calvo-torras. 2016. “Incidence of Filamentous Fungi with Toxigenic Potential on Samples of Feed and Raw Materials for their Manufacture.” Journal of Microbiology, Biotechnology and Food Sciences 5 (6): 599–601. https://doi.org/10.15414/jmbfs.2016.5.6.599-601.
- Waite, D. W., and M. Taylor. 2015. “Exploring the Avian Gut Microbiota: Current Trends and Future Directions.” Frontiers in Microbiology 6:673. https://doi.org/10.3389/fmicb.2015.00673.
- Walugembe, M., J. C. F. Hsieh, N. J. Koszewski, S. J. Lamont, M. E. Persia, and M. F. Rothschild. 2015. “Effects of Dietary Fibre on Caecal Short-Chain Fatty Acid and Caecal Microbiota of Broiler and Laying-Hen Chicks.” Poultry Science 94 (10): 2351–2359. https://doi.org/10.3382/ps/pev242.
- Wang, S., L. Chen, M. He, J. Shen, G. Li, Z. Tao, R. Wu, and L. Lu. 2018. “Different Rearing Conditions Alter Gut Microbiota Composition and Host Physiology in Shaoxing Ducks.” Scientific Reports 8 (1): 7387. https://doi.org/10.1038/s41598-018-25760-7.
- Weber, W. H. 2017. “Overview of Gastrointestinal Regulatory Peptides.” Current Opinion in Endocrinology, Diabetes and Obesity 23 (1): 1–2. https://doi.org/10.1097/MED.0000000000000222.
- Wen, H., J. P. Y. Ting, and A. J. o’neill. 2012. “A Role for the NLRP3 Inflammasome in Metabolic Diseases—Did Warburg Miss Inflammation?” Nature Immunology 13 (4): 352–357. https://doi.org/10.1038/ni.2228.
- Wickramasuriya, S. S., I. Park, Y. Lee, L. M. Richer, C. Przybyszewski, C. G. Gay, J. G. van Oosterwijk, and H. S. Lillehoj. 2023. “Orally Delivered Bacillus subtilis Expressing Chicken NK-2 Peptide Stabilizes Gut Microbiota and Enhances Intestinal Health and Local Immunity in Coccidiosis-Infected Broiler Chickens.” Poultry Science 102:102590. https://doi.org/10.1016/j.psj.2023.102590.
- Wideman, R. F., K. R. Hamal, J. M. Stark, J. Blankenship, H. Lester, K. N. Mitchell, G. Lorenzoni, and I. Pevzner. 2012. “A Wire-Flooring Model for Inducing Lameness in Broilers: Evaluation of Probiotics as a Prophylactic Treatment.” Poultry Science 91 (4): 870–883. https://doi.org/10.3382/ps.2011-01907.
- Willing, B. P., and A. G. van Kessel. 2010. “Host Pathways for Recognition: Establishing Gastrointestinal Microbiota as Relevant in Animal Health and Nutrition.” Livestock Science 133 (1–3): 82–91. https://doi.org/10.1016/j.livsci.2010.06.031.
- Witter, R., and K. Schat. 2003. “Marek’s Disease.” In Disease of Poultry. Agricultural Research Service U.S, edited by Y. M. Saif, J. Barnes, A. Fadly, J. Glisson, L. Mcdougal, and D. Swayne, 407–465. Urbana, Illinois: Department of Agriculture.
- Xu, Z. R., C. H. Hu, M. S. Xia, X. A. Zhan, and M. Q. Wang. 2003. “Effects of Dietary Fructooligosaccharide on Digestive Enzyme Activities, Intestinal Microflora and Morphology of Male Broilers.” Poultry Science 82 (6): 1030–1036. https://doi.org/10.1093/ps/82.6.1030.
- Yausheva, E. V., S. A. Miroshnikov, D. B. Kosyan, and E. A. Sizova. 2016. “Nanoparticles in Combination with Amino Acids Change Productive and Immunological Indicators of Broiler Chicken.” Agricultural Biology 51 (6): 912–920. https://doi.org/10.15389/agrobiology.2016.6.912eng.
- Yegani, M., and D. R. Korver. 2008. “Factors Affecting Intestinal Health in Poultry.” Poultry Science 87 (10): 2052–2063. https://doi.org/10.3382/ps.2008-00091.
- Yeoman, C. J., N. Chia, P. Jeraldo, M. Sipos, N. D. Goldenfeld, and B. A. White. 2012. “The Microbiome of the Chicken Gastrointestinal Tract.” Animal Health Research Reviews 13 (1): 89–99. https://doi.org/10.1017/S1466252312000138.
- Yu, H., Q. Wang, J. Tang, L. Dong, G. Dai, T. Zhang, G. Zhang, et al. 2023. “Comprehensive Analysis of Gut Microbiome and Host Transcriptome in Chickens after Eimeria Tenella Infection.” Frontiers in Cellular and Infection Microbiology 13:1191939. https://doi.org/10.3389/fcimb.2023.1191939.
- Yu, L. C. H., J. T. Wong, S. C. Wei, and Y. H. Ni. 2012. “Host Microbial Interactions and Regulation of Intestinal Epithelial Barrier Functions: From Physiology to Pathology.” World Journal of Gastrointestinal Pathophysiology 3 (1): 27–43. https://doi.org/10.4291/wjgp.v3.i1.27.
- Zain, M. E. 2011. “Impact of Mycotoxins on Humans and Animals.” Journal of Saudi Chemical Society 15 (2): 129–144. https://doi.org/10.1016/j.jscs.2010.06.006.
- Zaneveld, J. R., R. McMinds, and V. R. Thurber. 2017. “Stress and Stability: Applying the Anna Karenina Principle to Animal Microbiomes.” Nature Microbiology 2 (9): 1–8. https://doi.org/10.1038/nmicrobiol.2017.121.
- Zhang, S., T. Wei, H. Tianv, J. Cheng, J. Xiao, M. Wang, and Y. Hu. 2015. “Small Intestinal Injury in Mice Infected with Respiratory Influenza a Virus: Evidence for Virus Induced Gastroenteritis.” Biotechnology Letters 37 (8): 1585–1592. https://doi.org/10.1007/s10529-015-1847-8.
- Zhou, H., J. Gong, J. T. Brisbin, H. Yu, B. Sanei, P. Sabour, and S. Sharif. 2007. “Appropriate Chicken Sample Size for Identifying the Composition of Broiler Intestinal Microbiota Affected by Dietary Antibiotics, Using the Polymerase Chain Reaction Denaturing Gradient Gel Electrophoresis Technique.” Poultry Science 86 (12): 2541–2549. https://doi.org/10.3382/ps.2007-00267.
- Zsak, L., K. O. Strother, and J. Kisary. 2008. “Partial Genome Sequence Analysis of Parvoviruses Associated with Enteric Disease in Poultry.” Avian Pathology 37 (4): 435–441. https://doi.org/10.1080/03079450802210648.