Abstract
The aim of this study was to compare seed germination percentage, seedling growth, total chlorophyll and protein content, sugar content, and root and sugar yields of sugar beet (Beta vulgaris L.) genotypes at different ploidy levels. Sugar beet seeds from cultivars “Agnessa” and “Felicita” (diploids), and lines “AD 440” and “CBM 315” (tetraploids) were obtained from the Sugar Research Institute, Etimesgut, Ankara. Seed germination percentage was recorded after 4 days, whereas seedling and root lengths were noted 4 and 14 days after the study initiation. The size of epidermal cells in length and width were determined on the upper leaf surface of 6-week-old plants. Six and 10 weeks after seed sowing, plant and root lengths, leaf length and width, plant fresh and dry weights, and total chlorophyll and protein content were measured. Data related to yield components such as sugar content, root and sugar yields were obtained from trials conducted by the Sugar Research Institute under field conditions 6 months after study initiation. In the current study, diploid genotypes showed superior responses regarding generative characteristics while tetraploids passed diploid ones vegetatively in the further stages of the development. Ten weeks after study initiation, the highest results regarding vegetative characters such as plant height, root and leaf lengths, leaf width, approximate leaf area, plant fresh and dry weights, and tissue water content were recorded from tetraploid genotypes as 35.00 cm, 43.85 cm, 18.40 cm, 9.25 cm, 170.35 cm2, 369.10 g, 77.45 g and 291.65 g, respectively while the highest total chlorophyll content and protein percentage was obtained from diploid genotypes as 1552.65 μg g–1 fresh tissue and 21.11%, respectively. The highest values of sugar content, root and sugar yields were obtained from diploid genotypes as 15.55%, 71.47 t ha–1 and 11.09 t ha–1, respectively.
Introduction
Differences between diploid and polyploid plants include morphological, physiological, cellular and biochemical aspects. Polyploid plants have bigger cells and stomatas than diploid plants, resulting in thicker and larger leaves, larger flowers and fruits. In general, autotetraploids have greater vegetative volume and larger seed weight but lower reproductive fertility than diploids, and flowering and fruit formation are often later in tetraploids than in diploids as reported by Stebbins (Citation1947). Shoots of polyploid genotypes are thicker with short internodes and wider crotch angles. As the chromosome number increases, DNA content per cell, enzyme activity per cell and cell volume all increases (Molin et al. Citation1982; Warner et al. Citation1987). In addition, polyploids are used as sources of variability and new genotypes for plant improvement (Jan Citation1988; Hussain et al. Citation1997).
Polyploid genotypes have shown resistance to biotic (pests and pathogens) and abiotic (drought and cold, etc) stress factors in some cases and this resistance enables them to have greater adaptability to wider ecological regions. This could be attributed to higher chromosome number and gene expression causing an increase in the concentration of secondary metabolites and chemicals that are responsible for defense mechanisms. This increase in the concentration of secondary metabolites and chemicals enables polyploid genotypes to resist biotic and abiotic stress factors, and consequently to grow in a wide range of environments.
However, the effects of increased ploidy level cannot be anticipated all the time. Polyploid individuals may not always be superior to diploid ones: in some cases polyploid plants have slower growth rates (Ranney Citation2006) which could be attributed to difficulties in the cell cycle and slow cell division (Comai Citation2005), causing lower cell numbers and smaller organs. For example, it was reported that the overall chlorophyll content in polyploid plants is higher than diploid ones with lower chromosome numbers (Molin et al. Citation1982; Warner et al. Citation1987; Mathura et al. Citation2006), while total chlorophyll content of tetraploid sugar beet genotypes (“AD 440” and “CBM 315”) in our study were found to be lower than that of diploid ones (“Agnessa” and “Felicita”).
The aim of this study was to compare sugar beet (Beta vulgaris L.) genotypes at different ploidy levels with respect to seed germination, seedling growth, total chlorophyll and protein content, sugar content, root and sugar yields.
Materials and methods
Seeds of genotypes “Agnessa” and “Felicita” (diploid, high root yield)), and “AD 440” and “CBM 315” (tetraploid, high root yield) were obtained from the Sugar Research Institute, Etimesgut, Ankara.
Seeds were placed in bottles containing 200 ml tap water and shook for 24 hours to increase permeability of true seed coat for rapid germination. After that, seeds were sown in wooden boxes under greenhouse conditions at 25°C with a 14 h light/10 h dark photoperiod. All boxes were irrigated at 2-day intervals. Seed germination percentage was recorded after 4 days, whereas seedling and root lengths were noted 4 and 14 days after the study initiation. Data related to yield components such as sugar content, root and sugar yields were obtained from trials conducted by the Sugar Research Institute under field conditions.
The sizes of epidermal cells in length and width in a field of view area (105,938 μm2) of a microscope on the upper leaf surface of 6-week-old plants were determined using clear fingernail polish, clear tape, a glass slide, and a microscope at 60× magnification.
After 6 and 10 weeks, plant and root lengths, leaf length and width, plant fresh and dry weights, and total chlorophyll and protein content were measured. Plants were weighed to determine the fresh weight while the dry weight was noted after drying plants at 70°C for 4 days. All measurements were made using an analytical scale, with precision of 0.01 g.
Total chlorophyll content was determined in 6- and 10-week-old plants according to the protocol of Curtis and Shetty (1996). Fresh tissue (50 mg) from the leaf was put in 3 ml methanol and kept in total darkness at 23°C for 2 hours. In this way, chlorophyll in the fresh tissue passed through into methanol. After 2 hours, absorbencies were determined at 650 and 665 nm. Total chlorophyll content was calculated as μg chlorophyll/g fresh tissue.
Three replicates were tested. The number of plants per replication for seed germination and seedling establishment was 40 and for other parameters examined was 15. Data were statistically analyzed by Duncan’s multiple range test using the SPSS for Windows computer program. Data presented in percentages were subjected to arcsine () transformation before statistical analysis (Snedecor and Cochran 1967).
Results
In the current study conducted in sugar beet, which is an important sucrose-producing crop worldwide in temperate regions and supplies about 21% of the world sugar consumption, two diploid (“Agnessa” and “Felicita”) and two tetraploid (“AD 440” and “CBM 315”) sugar beet genotypes were compared with respect to seed germination, seedling growth, total chlorophyll and protein content, sugar content, and root and sugar yields. The sizes of epidermal cells in a field of view area on the upper leaf surface of sterile seedlings were counted. The number of cells in a field of view area was higher in diploid genotypes than in tetraploids due to small cell sizes. The mean number of cells was recorded as 160.22 in diploid genotypes while it was 75.50 in tetraploids (Table , Figure ). On the other hand, approximate cell area was found to be higher in tetraploids than in diploids. Mean approximate cell area was calculated as 2713.32 μm2 in diploids while it was 6531.02 μm2 in tetraploid genotypes (Table , Figure ).
Table 1. Cell sizes in the upper leaf surface of 6-week-old sugar beet genotypes at different ploidy levels.
Figure 1 Cells and stomatas from the upper leaf surface of 6-week-old sugar beet seedlings. (a) Diploid genotype “Felicita” and (b) Tetraploid genotype “AD 440”.
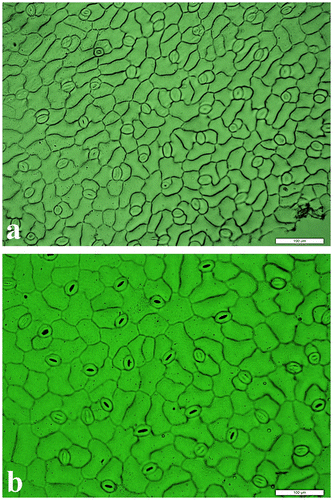
Diploid genotypes gave higher results than tetraploids in seed germination percentage, root length and seedling height at the fourth day, and root length and seedling height at the 14th day. In diploids, root length was recorded as 7.93 cm at the fourth day and it was noted as 13.32 cm at the 14th day. This indicated a 68.00% increase in root length. However, in the case of tetraploid genotypes, root length was recorded as 5.46 cm at the fourth day while it was 7.51 cm at the 14th day, which meant an increase of 37.55%. On the other hand, seedling height in diploids was recorded as 6.67 cm at the fourth day while it reached 9.29 cm at the 14th day. In tetraploids, seedling height was measured as 5.62 cm at the fourth day whereas it reached 6.80 cm at the 14th day. This lower increase in root length and seedling height in tetraploid genotypes could be attributed to lower metabolic activity and slower cell cycle (Table ).
Table 2. Germination and seedling growth in diploid and tetraploid sugar beet genotypes.
In the first six weeks, diploid genotypes gave rise to the highest results with respect to plant height, root length, leaf length and width, plant fresh and dry weights, total chlorophyll content and protein percentage. Mean plant height reached 19.00 cm in diploid genotypes while it was 15.07 cm in tetraploids six weeks after study initiation. Mean leaf area was recorded as 17.28 cm2 in diploids and 12.02 cm2 in tetraploids. Mean plant fresh and dry weights were calculated as 10.45 g and 2.64 g in diploid genotypes while they were 5.44 g and 1.06 g in tetraploids. Tissue water content was 7.82 g in diploids and 4.38 g in tetraploids. Total chlorophyll content was noted as 964.77 μg g–1 fresh tissue in diploids while it was 747.90 μg g–1 fresh tissue in tetraploids. Protein was calculated 19.76% in diploids and 4.61% in tetraploids (Figure , Table ). However, diploids were passed by tetraploid genotypes in the further stages of development in the characters of plant height, root length, leaf length and width, and plant fresh and dry weights. Mean plant height in diploid genotypes was 23.90 cm while it was 35.0 cm in tetraploids 10 weeks after study initiation. Mean leaf area was calculated as 100.30 cm2 in diploids and 170.35 cm2 in tetraploids. Mean plant fresh and dry weights were recorded as 296.35 g and 47.25 g in diploid genotypes while they were 369.10 g and 77.45 g in tetraploids. Tissue water content was recorded as 249.10 g in diploids and 291.65 g in tetraploids. Total chlorophyll content and protein percentage, which could be accepted as generative characters, were both found higher in diploids than in tetraploids. Total chlorophyll content was recorded as 1552.65 μg g–1 fresh tissue in diploids and 1243.55 μg g–1 fresh tissue in tetraploids. Protein was detected as 21.11% in diploid genotypes and 16.74% in tetraploids (Figure , Table ). It was thought that this could be due to the fact that photosynthetic capacity of the tissue in diploids was higher because of higher chlorophyll content, water and nutrient uptake from the soil with their roots. Higher photosynthetic capacity resulted in higher protein content in diploids.
Figure 2 Seedlings developed from seeds of (a) diploid (“Felicita”) and (b) tetraploid (“AD 440”) genotypes 6 weeks after study initiation (bar = 3 cm).
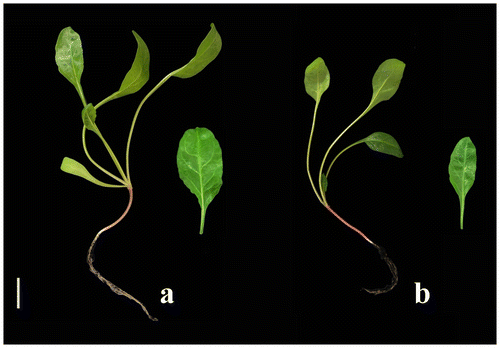
Table 3. Development of seedlings from seeds of diploid and tetraploid genotypes 6 weeks after study initiation.
Figure 3 Seedlings developed from seeds of (a) diploid (“Felicita”) and (b) tetraploid (“AD 440”) genotypes 10 weeks after study initiation (bar = 5 cm)
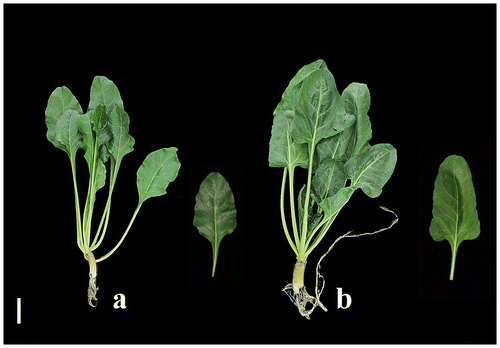
Sugar content, root and sugar yields were recorded as 15.55%, 71.47 t ha–1 and 11.09 t ha–1 in diploid genotypes while they were 12.46%, 56.53 t ha–1 and 7.04 t ha–1 in tetraploids, respectively ().
Table 4. Development of seedlings from seeds of diploid and tetraploid genotypes 10 weeks after study initiation.
Table 5. Sugar content, root and sugar yields in diploid and tetraploid genotypes.
Discussion
There are differences between diploid and polyploid plants from morphological, physiological, cellular and biochemical aspects (Berkov and Philipov Citation2002).
The highest values of cell size were recorded in tetraploid genotypes, as reported by Smith et al. (Citation2003) who noted that polyploidy increased the cell size and volume. Decreased cell number in polyploid genotypes was compensated by increased cell size as reported by Doonan (Citation2000) and Inze and De Veylder (Citation2006). Our findings verified these studies that lower cell number (75.50) in tetraploid genotypes was compensated by increased cell size (Table ).
Plants developed from diploid seeds were more vital and well-grown in the first stages of the development. This could be due to the fact that polyploid seeds had lower germination and emergence percentages than diploid ones due to their thicker seed coat and seedling emergence strength (Sung and Chui Citation1995; Kihara Citation1951). Plant height and root length scores, which were higher in diploid genotypes in the first 6 weeks, were good indicators for vitality and growth. High ploidy level does not result in increased shoot growth all the time. Leaf area, which plays an important role in the photosynthetic activity, was found to be higher in diploid genotypes in the first 6 weeks. However, at the 10th week, although leaf area of tetraploid genotypes was greater than in diploids, total chlorophyll content was determined to be higher in diploids than in tetraploids. High ploidy level does not result in increased shoot growth every time (Pegtel Citation1999). Polyploid plants may show high-ploidy syndrome, with a costly cell cycle and slow cell division at higher ploidy levels. Lower results in morphological characters in the first developmental stages of tetraploid genotypes could be attributed to slow cell division, as reported by Comai (Citation2005).
Chlorophyll content, which is accepted as an indicator of photosynthetic capacity of tissues (Pal and Laloraya Citation1972; Wright et al. Citation1994; Nageswara et al. Citation2001), was again found to be higher in diploid plants. The number of photosynthetic cells per unit leaf area decreases with increasing ploidy level (Warner and Edwards Citation1989). Although chloroplasts (Beck, Dunlop et al. Citation2003; Beck, Fossey et al. 2003) and chlorophyll content (Molin et al. Citation1982) are higher in polyploid genotypes, increased chlorophyll content with increasing ploidy level is not always apparent. For instance, chlorophyll content remained constant in different ploidy levels of Atriplex confertifolia (Warner and Edwards Citation1989).
It was reported that the fresh weight increase was mainly due to cell enlargement by water absorption (Dale Citation1988) and increase in dry weight was closely related to cell division and new material synthesis via photosynthesis (Sunderland Citation1960). Dry weight increase in diploids was due to an increase in photosynthetic activity and carbohydrate metabolism resulting from increased water uptake by longer roots. Reduced fresh weight in tetraploids in the beginning of the development could be attributed to decreased water absorption as reported by Prado et al. (Citation1995). Sullivan and Pfahler (Citation1986) reported that seedling growth was affected by genotypic differences more than ploidy in diploid and autotetraploid Secale cereale.
Cells with high ploidy level have bigger vacuoles (Jibiki et. al. Citation1993) and the vacuole plays an important role in regulating osmotic pressure of the cell (Guertin and Sabatini Citation2005). Higher cell osmotic pressure in polyploid plants as reported by Tal and Gardi (2006) could cause high tissue metabolic activity by increasing water and nutrition uptake. Thus, increase in the fresh and dry weights of tetraploid genotypes in our study at the end of 10th week were chiefly due to an increase in the absorption of water and other components via high cell osmotic pressure. Conversely, lower osmotic pressure of diploid genotypes caused a decline in fresh and dry weights by decreasing the absorption of water and other components via roots. The results of tissue water content clearly showed that tetraploid genotype had higher osmotic pressure, causing higher absorption of water and other components from the soil. In general, tetraploids have higher vegetative growth but lower reproductive fertility than diploids. Polyploids flower and fruit later than diploids, as reported by Stebbins (Citation1947). Thus, in our study, tetraploid genotypes overtook diploid genotypes 10 weeks after study initiation, in vegetative characters such as plant height, root and leaf lengths, leaf width, approximate leaf area, plant fresh and dry weights. However, in generative characters (total chlorophyll content, protein percentage, sugar content, root and sugar yields), diploid genotypes showed the highest performance.
From the results of the present study, the lower levels of parameters recorded in tetraploid genotypes confirmed that the effects of increased ploidy level could not be anticipated all the time. Diploid genotypes were found to be superior to tetraploids in generative characteristics such as total chlorophyll and protein content, sugar content, root and sugar yields. Thus, it should be considered that responses of polyploid genotypes may differ in morphological, physiological, cellular and biochemical aspects. That is why, in a research study, responses of both diploid and polyploid genotypes should be evaluated carefully for successful results.
References
- Beck , SL , Dunlop , RW and Fossey , A . 2003 . Evaluation of induced polyploidy in Acacia mearnsii through stomatal counts and guard cell measurements . S Afr J Bot , 69 : 563 – 567 .
- Beck , SL , Fossey , A and Mathura , S . 2003 . Ploidy determination of black waffle (Acacia mearnsii) using stomatal chloroplast counts . S Afr Forest J , 192 : 79 – 92 .
- Berkov , S and Philipov , S . 2002 . Alkoloid production in diploid and autotetraploid plants of Datura stramonium . Pharm Biol , 40 : 617 – 621 .
- Comai , L . 2005 . The advantages and disadvantages of being polyploid . Nat Genet , 6 : 836 – 846 .
- Curtis , OF and Shetty , K . 1996 . Growth medium effects on vitrification, total phenolics, chlorophyll, and water content of in vitro propagated oregano clones . Acta Hortic , 426 : 498 – 503 .
- Dale , JE . 1988 . The control of leaf expansion . Annu Rev Plant Physiol , 39 : 267 – 295 .
- Doonan , J . 2000 . Social control on cell proliferation in plants . Curr Opin Plant Biol , 3 : 482 – 487 .
- Guertin DA, Sabatini DM. 2005. Encyclopedia of life sciences. Chichester: John Wiley & Sons. Cell size control; p. 1–10.
- Hussain , SW , Williams , WM , Woodfield , DR and Hampton , JG . 1997 . Development of a ploidy series from a single interspecific Trifolium repens L&A macr: nigrescens Viv. F1 hybrid . Theor Appl Genet , 94 : 821 – 831 .
- Inze , D and De Veylder , L . 2006 . Cell cycle regulation in plant development . Annu Rev Genet , 40 : 77 – 105 .
- Jan , CC . 1988 . Induced tetraploidy and trisomic production of Heliantus annus L . Genome , 30 : 647 – 651 .
- Jibiki , M , Kuno , Y , Shinoyama , H and Fujii , T . 1993 . Isolation and properties of large cell strains from a methanol-utilizing yeast, Candida sp. N-16 by colchicine treatment . J Gen Appl Microbiol , 39 : 439 – 442 .
- Kihara , H . 1951 . Triploid watermelons . Proc Am Soc Hortic Sci , 58 : 217 – 230 .
- Mathura , S , Fossey , A and Beck , S . 2006 . Comparative study of chlorophyll content in diploid and tetraploid black Wattle (Acacia mearnsii) . Forestry , 79 ( 4 ) : 381 – 388 .
- Molin , WT , Meyers , SP , Baer , GR and Schrader , LE . 1982 . Ploidy effects of isogenic populations of alfalfa II. Photosynthesis, chloroplast number, ribulose-1,5-bisphosphate carboxylase, chlorophyll, and DNA in protoplasts . Plant Physiol , 70 : 1710 – 1714 .
- Nageswara , RRC , Talwar , HS and Wright , GC . 2001 . Rapid assessment of specific leaf area and leaf nitrogen in peanut (Arachis hypogaea L.) using chlorophyll meter . J Agron Crop Sci , 189 : 175 – 182 .
- Pal , RN and Laloraya , MM . 1972 . Effect of calcium levels on chlorophyll synthesis in peanut and linseed plants . Biochem Physiol Pflan , 163 : 443 – 449 .
- Pegtel , DM . 1999 . Effect of ploidy level on fruit morphology, seed germination and juvenile growth in scurvy grass (Cochlearia officinalis L. s.l., Brassicaceae) . Plant Species Biol , 14 : 201 – 215 .
- Prado , FE , Gonzalez , JA , Gallardo , M , Moris , M , Boero , C and Kortsarz , A . 1995 . Changes in soluble carbonhydrates and invertase activity in Chenopodium quinoa (“quinoa”) developed for saline stress during germination . Curr Topic Phytochem , 14 : 1 – 5 .
- Ranney , TG . 2006 . Polyploidy: From evolution to new plant development . Proc Int Plant Propagator Soc , 56 : 604 – 607 .
- Smith , KF , McFarlane , NM , Croft , VM , Trigg , PJ and Kearney , GA . 2003 . The effects of ploidy and seed mass on the emergence and early vigour of perennial ryegrass (Lolium perenne L.) cultivars . Aust J Exp Agric , 43 : 481 – 486 .
- Snedecor GW, Cochran WG. 1967. Statistical Methods. 6th ed. Iowa State University Press, Ames, IA.
- Stebbins , GL . 1947 . Types of polyploids: their classification and significance . Adv Genet , 1 : 403 – 429 .
- Sullivan , BP and Pfahler , PL . 1986 . Diploid-tetraploid comparison in rye. III. Temperature effects on seedling development . Crop Sci , 26 : 795 – 799 .
- Sung , JM and Chiu , KY . 1995 . Hydration effect on seedling emergence strength of watermelon seeds differing in ploidy . Plant Sci , 110 : 21 – 26 .
- Sunderland , N . 1960 . Cell division and expansion in the growth of the leaf . J Exp Bot , 11 : 68 – 80 .
- Tal , M and Gardi , I . 1976 . Physiology of polyploid plants: Water balance in autotetraploid and diploid tomato under low and high salinity . Physiol Plant , 38 ( 4 ) : 257 – 261 .
- Warner , DA and Edwards , GE . 1989 . Effects of polyploidy on photosynthetic rates, photosynthetic enzymes, contents of DNA, chlorophyll, and sizes and numbers of photosynthetic cells in the C4 dicot Atriplex confertifolia . Plant Physiol , 91 : 1143 – 1151 .
- Warner , DA , Ku , MSB and Edwards , GE . 1987 . Photosynthesis, leaf anatomy, and cellular constituents in the polyploid C4 grass Panicum virgatum . Plant Physiol , 84 : 461 – 466 .
- Wright , GC , Nageswara , RRC and Farquhar , GD . 1994 . Water use efficiency and carbon isotope discrimination in peanut under water deficit conditions . Crop Sci , 34 : 92 – 97 .