Abstract
Clematis heracleifolia is a beautiful garden plant, and both the whole plant and its root are important in traditional Chinese medicine. Polyploids are usually more valuable because they exhibit increased biomass and content of effective compounds for medicinal plants. This research aimed to induce tetraploidy in C. heracleifolia by the apical meristem method using 0.2% colchicine. Apical buds were induced under three treatments (24, 48 and 72 h) to explore the best treatment time. Cytology identification was accomplished for the obtained mutants and diploid controls. It showed that the highest mutation rate of up to 80% was obtained with the 48 h treatment. The leaves of mutants were much broader, thicker, and showed lower leaf index compared with controls by morphology examination. There were significant differences between tetraploids and controls for stoma size and stoma frequency at the 0.01 or 0.05 levels. The chromosome number of most mutants was 32 (2n = 4x), while the chromosome number of diploid controls was 16 (2n = 2x) by cytology observation of root tip cells. Mutants were further confirmed by the meiosis behavior of pollen mother cells. Compared with regular meiosis of diploid controls, induced tetraploids were cytogenetically distinguished from diploids by the occurrence of a quadrivalent configuration at diakinesis, asynchronous chromosome segregation at meiosis II and finally generating polyads with various numbers of unbalanced microspores. Other abnormalities were observed as laggard chromosomes and some chromosome bridges at anaphase I and II in induced tetraploids. As a consequence tetraploid mutants of C. heracleifolia were successfully developed by colchicine induction and identified in this study. This has implications for further breeding programs.
Introduction
The genus Clematis contains ∼300 species native to many regions of the globe, including the well-known large-flowered cultivars, as well as the lesser-known nonvining species (Dale Citation2007). Clematis heracleifolia is one of a type of clematis that is often described as “herbaceous erect”. The whole plant and its root are important in traditional Chinese medicine (Mimaki et al. Citation2004).
Since it bears both male and female blossoms on the same plant (as most clematis do), C. heracleifolia is self-fertile, but it readily interbreeds with related species. As a consequence there is often a confusing tangle of hybrids and select forms. Intersimple sequence repeat (ISSR) primers were used to fingerprint the genus Clematis for assessing genetic relationships and cultivar identification (Gardner and Hokanson Citation2005). A comparative study was also performed to identify and classify the genus Clematis on the basis of morphological and phytochemical characters (Ishtiaq et al. Citation2010), but few polyploidy breeding reports for C. heracleifolia have been available until now.
Although polyploids frequently encounter low seed-setting rates or complete sterility (Lewis Citation1980), they usually show larger organ size and superior cold tolerance (Kato and Birchler Citation2006). As they are often larger polyploids have increased commercial interest, and are attractive for agricultural production (Elrad and Unal Citation2010). For this reason, the polyploidy procedure is used for root crops, fruit trees and ornamental flowers (Kato and Birchler Citation2006). For medicinal plants, polyploids are usually more valuable because they exhibit increased biomass and content of effective compounds (Gao et al. Citation1996). So polyploidy breeding is an effective approach of germplasm development for C. heracleifolia.
The objectives of our study were to induce tetraploidy in C. heracleifolia by colchicine treatment, and to identify tetraploid mutants by chromosome counting, stoma measurement and meiosis behavior of pollen mother cells (PMCs).
Materials and methods
Plant material
Seeds of C. heracleifolia came from the Natural Medicinal Plant Garden in Shanxi province. The first generation, M1, acted as material for meiosis examination, while M2 was suitable for mitosis examination in this study.
Induction of polyploidy
Approximately three seeds per pots were planted with sunshine potting soil. When seedlings were at the cotyledon stage, 45 pots were treated and 15 pots were left as controls. Treatment pots were divided into three groups: 15 pots for 24 h (one application), 15 pots for 48 h (two applications) and 15 pots for 72 h (three applications). The suitable plant (subsample) in each pot was treated by semi-solid 1% agar with 0.2% colchicine. A single drop of the warm semi-solid was applied on the apical meristem of seedlings between the first pair of true leaves. That was one application and two or three applications were conducted in every consecutive 24 h. The treated pots were then covered by a plastic cup under a high humidity growth chamber at 25°C. After each treatment, the plastic cup was uncovered and the residue was carefully removed with tweezers. Sprinkling water and nutritive solution (0.3 g CON2H4 and 0.5 g KH2PO4 in 1 L distilled water) were applied by turns (water in the first day, nutritive solution in the second day and so on) to help the seedling growth for two weeks and then seedlings were transplanted in the field.
Morphological examination
Morphological characteristics of three treatments were examined during the seedling growth period. Abnormal plants with crimpled and dark green leaves were measured as mutant preliminary indicators and mutation rates of different treatments were statistically estimated.
Cytological identification
The ploidy level of C. heracleifolia was estimated by chromosome counting of root tips from putative mutants earlier determined by morphological characteristics. Chromosome spreads were made according to the method of Wu et al. (Citation2011). Microscope slides were examined with an Olympus BX51 (Japan) using a 40× objective. The chromosome number of all treatments in root tips was counted in at least 10 cells. A plant with all root tip cells showing 16 chromosomes was classified as diploid, and with all cells showing 32 chromosomes was determined as tetraploid.
Meiotic studies
Flower buds for meiotic studies were collected from each individual plant in the field between 8.00 and 11.00 am and fixed in freshly prepared Carnoy’s solution (ethanol: acetic acid = 3:1) for 24 h, then transferred to 70% alcohol, and stored under refrigeration for use. Meiotic analysis was carried out on flower buds of a suitable size; after washing the fixed buds in distilled water, anthers were squashed on slides in carbol fuchsin solution. Photographs were taken from freshly prepared slides using an Olympus BX60 microscope with automatic camera. Meiosis was studied using a minimum of 30 PMCs.
Stoma measurement
The ploidy level of C. heracleifolia was estimated by stoma measurement. The lower epidermis of each leaf sample was peeled off from obtained tetraploids and diploid controls in the same internode (the 12th) and placed on glass slides. Another drop of 1% I-KI solution was added to stain the stoma and covered with cover slip. Slides were examined with an Olympus BX51 using a 40× objective. The frequency and size of stoma under the same magnified scope were measured. At least 10 scopes were chosen and mean numbers were statistically calculated.
Data analysis
The experimental design was completely randomized and data analyses were performed using SAS software (SAS Institute, Cary, NC, 1995).
Results
Estimation of mutation rates
Morphology characters provide early evidence for estimation of ploidy level. The seedling growth and development of mutants was considerably changed by colchicine treatment and mutants were morphologically distinguished by vegetative growth, showing broader and thicker leaf and lower leaf index. The mutation rates for the 24, 48 and 72 h treatments were 53.3%, 80% and 40% respectively (Table ). This showed that a 48 h colchicine treatment was the most efficient for mutagenesis, producing 80% of mutants. Increasing the colchicine treatment time also increased the number of deaths.
Table 1. Inducing effect of colchicine on C. heracleifolia at different treatment times.
Chromosome counting
Chromosome counting in root-tip cells is direct evidence for testing ploidy level in plants. All obtained mutants were examined by chromosome counting and most of them revealed the presence of 2n = 4x = 32 chromosomes and they were confirmed as tetraploids. The chromosome number of all diploid controls was 16 at metaphase plates in mitosis of root tips by microscope observation (Figure ).
Stoma measurement
Lower epidermis from two strictly comparable leaves in the same internode (the 12th) showed an increase in the size of stoma between obtained tetraploids and diploid controls. By microscope measurement, the length and width of stoma ranged from 38.78 and 23.46 μm in controls to 46.56 and 32.76 μm in tetraploids (Figure ). However, stoma frequency decreased from controls (36.88/10 μm2) to tetraploids (28.86/10 μm2) (Table ). Statistical analysis revealed that there were significant differences for stoma width and stoma frequency at the 0.05 level, and significant differences for stoma length at 0.01 or 0.05 levels, between tetraploids and controls. This study suggested that polyploid mutants could be identified by stoma size, stoma frequency, and that these are useful indicators in primary screening for new ploidy level.
Figure 2 Comparisons for stoma measurement of C. heracleifolia. (A) Diploid control; (B) induced tetraploid (10 × 40).
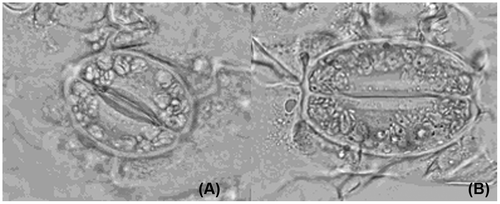
Table 2. Comparison of stoma size and frequency between mutants and controls.
Abnormal meiotic behavior of induced tetraploids
Meiotic behavior in diploid controls was quite normal in the first division and completely normal in the second division, generating 100% of normal tetrads. Observations were also made on chromosome morphology and behavior during meiosis for induced tetraploids. In the majority of cells, the chromosomes were dispersed in the cytoplasm as either bivalents or univalents, and quadrivalent configuration without forming a metaphase plate (Figure A, B). The other common meiotic abnormalities observed in tetraploids were laggard chromosomes in anaphase I (Figure C, D), and some irregular chromosome bridges in telophase I and II (Figure E, F). At meiosis II, the activation of chromosome segregation was asynchronous. Some activated earlier, while others later (Figure G, H). After the occurrence of an irregular first cytokinesis, the meiocytes progressed normally to the second division, generating polyads with various numbers of unbalanced microspores mainly because anaphase chromosome movements were blocked. As a consequence of this abnormality in chromosome orientation, a few or several micronuclei of various sizes were observed inside the cells (Figure I, J). A similar meiotic abnormality was reported in maize (Taschetto and Pagliarini 1993) and in Poaceae (Mendes-Bonato et al. Citation2009), where the meiotic mutant lacked spindle organization in both divisions, generating polyads as the meiotic product.
Figure 3 Abnormal meiosis behavior of PMCs in induced tetraploid of C. heracleifolia. (A) Diplotene (showing bivalent);(B) diakinesis (showing quadrivalent); (C, D) telophase I (showing laggard chromosomes); (E, F) telophase I and II (showing chromosome bridge); (G, H) asynchronous chromosome segregation at telophase I; (I) triad; (J) heptad.
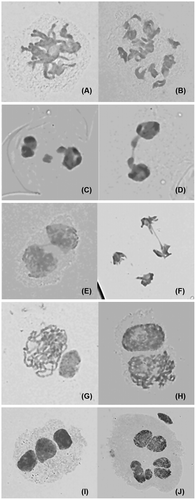
Discussion
Polyploidy
Polyploidy is defined as an increase in chromosome number by an exact multiple of the haploid chromosome number, and is a typical characteristic of plant species (Baatout Citation1999). Polyploids may have superior levels of adaptability and higher probabilities of survival (Soltis and Soltis Citation2000). The success of polyploid species in plants has been attributed to their ability to colonize a wider range of habitats and to survive better in unstable climates compared with their diploid progenitors, presumably due to increased heterozygosity and flexibility provided by the presence of additional alleles (Baatout Citation1999). There are several ways that autopolyploids and allopolyploids can be formed by the union of two non-reduced gametes, e.g. spontaneous somatic genome doubling or the merger of a reduced gamete with a non-reduced one, which would then give rise to triploids (Wendel and Doyle Citation2005). In the late 1930s it was discovered that colchicine inhibited the formation of spindle fibers and temporarily arrests mitosis at the anaphase stage. At this point, the chromosomes have replicated, but cell division has not yet taken place, resulting in polyploid cells (Blakeslee and Avery Citation1937).
Ovary, seed, shoots and axillary or sub-axillary meristems have been induced for polyploidy mutagenesis. But one of the easiest and most effective methods is to work with a large number of seedlings with small, actively growing meristems. Seedlings can be soaked or the apical meristems can be submerged with different concentrations, durations, or frequencies of a given doubling agent. In our study, an improved colchicine method was used on apical buds; the chemical is transported to other parts of plant by vascular bundle. If those parts include secondary meristem, induction can be produced the second time and cause far effect. Direct part of colchicine treatment can cause original effect. Besides, near effect can be produced by such plasmodesmata, pinocytosis and osmotic pressure (Wu et al. Citation2011). The potential role of various cyclins and cyclin dependent kinases in polyploidization is being investigated in several laboratories and will lead in the near future to a better understanding of the key elements responsible for polyploidization (Baatout et al. 1999).
Identification of polyploidy level
The direct evidence to test ploidy level is chromosome counting in mitosis and chromosome quadrivalent configuration in meiosis. Chromosome counting in root-tip cells is an accurate procedure to determine the ploidy level, but it is time-consuming and requires much experience. It is also helpful to confirm the ploidy level by studying the meiosis of PMCs. Meiosis is a premise of sexual reproduction and the behaviors in the meiosis are correlation with the chromosomes and the genes (Swanson et al. Citation1981). In all sexually reproducing organisms, meiosis is a complex process that helps to maintain a constant chromosomal number from generation to generation and ensures the operation of Mendel’s laws of heredity (Singh Citation1993; Wendel Citation2000). Extensive studies on the inactivation of the sex chromosomes during male meiosis will allow a better understanding of some male infertilities (Guichaoua et al. Citation2009).
Recently leaf index value, pollen grain diameter and other changes in plant morphology were found to be useful indicators in the primary screening for new ploidy levels. Another indirect identification method for ploidy level is stoma size, stoma frequency and the number of chloroplast in guard cells. Zhang et al. (Citation2010) confirmed that the number of chloroplasts in guard cells of stomata was a stable and reliable marker in discriminating crape myrtle plants of different ploidy levels. In this study we also found that stoma size of mutants is much bigger than that of controls, but stoma frequency is much lower than controls. However, when tetraploidy was induced in vitro in mat rush, there was a significant reduction of chlorophyll content that might be the result of structural modification in the lamellar membranes of chloroplasts (Xu et al. Citation2010). In many plant species, there are correlations between ploidy level and cytogenetic characteristics such as chloroplast number in guard cells, size of stomata cells, stomata density and pollen grain diameter (Sakhanokho et al. 2009; Zlesak 2009). Another method, flow cytometry (FCM) is the most efficient method for detecting ploidy level (Omidbaigi et al. Citation2010) and would appear to offer advantages for plant breeding (Costich et al. 1993).
Meiosis behavior
Meiosis consists of two consecutive nuclear divisions, a reductional division (meiosis I) and an equational division (meiosis II), without an intervening S phase between them. While meiosis II is essentially similar to a mitotic division, meiosis I is a specialized division, whose aim is to reduce the number of chromosomes in the nucleus and allow exchange of genetic material between maternal and paternal chromosomes (Ronceret et al. Citation2008). The key to proper segregation of chromosomes in meiosis consists of processes that take place in early meiotic prophase I and homologous chromosome pairing. However, in contrast with most other meiotic processes, little is known about the mechanisms controlling chromosome pairing in plants (Ronceret et al. Citation2007).
During meiosis, the duplicated chromosome segments recombine with each other, and a dicentric chromosome is formed. In the second division of meiosis, one centromere is pulled to one pole, while the second centromere is pulled to the opposite pole. As a result, the chromosome breaks in the region between the two centromeres. Then, two broken chromosome ends can fuse, which leads to a bridge (Han et al. Citation2007). The formation of bridges also seems to be the result of chromatin stickiness and late disjunction of bivalents (Savita et al. Citation2011). In this study, some irregular chromosome bridges and laggard chromosomes were observed in anaphase I and telophase I and II of tetraploids. Segregation in meiosis I and II also requires spindle formation and chromosome attachment to spindle microtubules. A large group of proteins, kinesins, is thought to be involved in spindle morphogenesis (Ronceret et al. Citation2008). All these abnormalities lead to the abnormal microsporogenesis which results in the formation of monads, diads, triads and polyads accompanied by micronuclei and it indicates the existence of intraspecifc genetic diversities (Savita et al. Citation2011).
Acknowledgments
This research was financially supported by National Natural Science Foundation of China (No. 31171599) and Research Project Supported by Shanxi Scholarship Council of China (2012-052) and Scientific and Technological Innovation Programs of Higher Education Institutions in Shanxi (No. 20081010).
References
- Baatout S. 1999. Molecular basis to understand polyploidy. Hematol Cell Ther. 41 (4): 169–170.
- Blakeslee AF, Avery AG. 1937. Methods of inducing doubling of chromosomes in plants by treatment with colchicine. J Hered. 28 (12): 393–411.
- Costich DE, Ortiz R, Mcagher TR, Bruederte LP, Vona N. 1993. Determination of ploidy level and nuclear DNA content in blueberry flow cytometry. Theor. Appl. Genet. 86 (1): 1001–1006.
- Elrad T, Unal M. 2010. Production of colchicine induced tetraploids in Vicia villosa roth. Caryologia. 63 (3): 292–303.
- Gao SL, Zhu DN, Cai ZH, Xu DR. 1996. Autotetraploid plants from colchicine-treated bud culture of Salvia miltior-rhiza Bge. Plant Cell, Tiss Org Cult. 47 (1): 73–77.
- Gardner N, Hokanson SC. 2005. Intersimple sequence repeat fingerprinting and genetic variation in a collection of Clematis cultivars in commercial germplasm. Hortscience. 40 (7): 1982–1987.
- Guichaoua MR, Geoffroy-Siraudin C, Tassistro V, Ghalamoun-Slaimi R, Perrin J, Metzler-Guillemain C. 2009. Sex chromosomes and meiosis. Gynécol obstét fertil. 37 (4): 895–900.
- Han F, Gao Z, Yu W, Birchler JA. 2007. Minichromosome analysis of chromosome pairing, disjunction, and sister chromatid cohesion in maize. Plant Cell. 19 (12): 3853–3863.
- Ishtiaq M, Qing H, Wang Y, Cheng Y. 2010. A comparative study using chemometric and numerical taxonomic approaches in the identification and classification of traditional Chinese medicines of the genus Clematis. Plant Biosyst. 144 (2): 288–297.
- Kato A, Birchler JA. 2006. Induction of tetraploid derivatives of maize inbred lines by nitrous oxide gas treatment. J Hered. 97 (1): 39–44.
- Lewis WH. 1980. Ploidy: Biological Relevance. NewYork: Plenum.
- Mendes-Bonato AB, Felismino MF, Souza Kaneshima AM, Pessim C, Calisto V, Pagliarini MS, Borges do Valle. C. 2009. Abnormal meiosis in tetraploid genotypes of Brachiaria brizantha (Poaceae) induced by colchicine: its implications for breeding. J Appl Genet. 50 (2): 83–87.
- Mimaki Y, Yokosuka A, Hamanaka M, Sakuma C, Yamori T, Sashida Y. 2004. Triterpene saponins from the roots of Clematis chinensis. J Nat Prod. 67 (9): 1511–1516.
- Ronceret A, Christopher GB, Pawlowski WP. 2007. Naughty behavior of maize minichromosomes in meiosis. Plant Cell. 19 (12): 3835–3837.
- Ronceret A, Sheehan MJ, Pawlowski WP. 2008. Chromosome dynamics in meiosis. Plant Cell Monog. 9 (4): 103–124.
- Sakhanokho HF, Rajasekaran K, Kelley RY, Islam-Faridi N. 2009. Induced Polyploidy in Diploid Ornamental Ginger (Hedychium muluense R. M. Smith) Using Colchicine and Oryzalin. HortScience. 44 (7): 1809–1814.
- Savita R, Sanjeev K, Mudassir JS, Santosh K, Chand GR. 2011. Cytological studies in some members of family Ranunculaceae from Western Himalayas (India). Caryologia. 64 (4): 405–418.
- Singh RJ. 1993. Plant cytogenetics. Boca Raton (FL): CRC Press p. 391.
- Soltis DE, Soltis PS. 2000. The role of genetic and genomic attributes in the success of polyploids. Proc Natl Acad Sci USA. 97 (13): 7051–7057.
- Wendel JF. 2000. Genome evolution in polyploids. Plant Mol Biol. 42 (1): 225–249.
- Wendel J, Doyle J. 2005. Polyploidy and evolution in plants. In: Henry RJ, ed. Plant diversity and evolution: genotypic and phenotypic variation in higher plants. Wallingford: CABI, 97–117.
- Wu YX, Yang FH, Zhao XM, Yang WD. 2011. Identification of tetraploid mutants of Platycodon grandiflorus by colchicine induction. Caryologia. 64 (3): 343–349.
- Xu L, Najeeb U, Naeem M, Daud M, Cao J, Gong H, Shen W, Zhou W. 2010. Induction of tetraploidy in Juncus effusus by colchicine. Biol Plantarum. 54 (4): 659–663.
- Zlesak DC. 2009. Pollen diameter and guard cell length as predictors of ploidy in diverse rose cultivars, species, and breeding lines. Floriculture and ornamental biotechnology. 3 (1): 53–70.