Abstract
The nuclear DNA content data available in “A genome size database in the Asteraceae” (GSAD: www.asteraceaegenomesize.com) have been analyzed, together with other parameters (i.e. ecological, karyological, cytogenetic), in order to establish hypotheses on the systematic, phylogenetic and evolutionary aspects of genome size in one of the largest angiosperm families. The novelty of this work is a comprehensive analysis of the whole family with the following aims: (1) to update the knowledge of genome size values in the Asteraceae; (2) to infer evolutionary trends of genome size, compared with other plant groups; and (3) to detect gaps in this field in the family and outline further research priorities. The analysis of this dataset shows that most Asteraceae genomes (57.23%) range from very small (1C ⩽ 1.4 pg) to small (1C ⩽ 3.5 pg). Gains and losses of DNA occur throughout the phylogeny of the family but although ancestral values for the basal nodes remain mostly equivocal, often small and very small ancestral genome sizes are reconstructed. Most genome size data (96.74%) are concentrated in five tribes, which broadly reflect their species richness. The relationships between genome size and other cytogenetic and ecological features have been analyzed and discussed, highlighting several general patterns. Further studies are needed to fill the gaps in genome size knowledge in the Asteraceae and more detailed research in some groups could provide information about mechanisms regulating genome expansions and contractions.
Introduction
Genome size, or C value, the amount of DNA of an unreplicated gametic nucleus (Swift Citation1950), is a key biodiversity character among angiosperms (Bennett and Leitch Citation2005b). The latest update of the angiosperm DNA C values database (release 6.0, Bennett and Leitch 2012) illustrates the increasing interest in knowledge of plant genome sizes over recent decades. The vast majority of angiosperms were shown to have small to very small genomes (1C ⩽ 1.4 pg and 1C ⩽ 3.5 pg, respectively), with a mode of just 1C = 0.7 pg and a mean of 1C = 5.79 pg (Bennett and Leitch 2012). Analyzing genome size evolution across the angiosperms in a phylogenetic context inferred a very small value for the angiosperm ancestor. It also revealed that genome size expansions leading to large and very large genomes are derived traits that have only occurred in a few specific lineages (Soltis et al. Citation2003).
Genome size diversity in plants relates, in part, to the huge range of chromosome numbers found, from n = 2 (there are two representatives of Asteraceae out of the five known species with this chromosome number, namely Brachyscome dichromosomatica and Haplopappus gracilis) to n = c. 300 for the palm Voanioala gerardii, and n = 320 in Sedum suaveolens (Uhl Citation1978; Bennett Citation1998; Heslop-Harrison and Schwarzacher Citation2011). Polyploidy, especially prevalent in plants, largely accounts for this trend. Indeed, up to 80% of land plants are believed to have a polyploid origin (Leitch and Leitch Citation2008), with evidence of genome duplications already reported within some of the early diverged angiosperm families (e.g. Nymphaeaceae; Cui et al. Citation2006). Even in a small genome such as Arabidopsis thaliana (1C = 0.16 pg), comparative genomic studies have identified numerous duplicate genes, suggesting it is actually a paleohexaploid (Vision et al. Citation2000; Bowers et al. Citation2003). The mechanisms responsible for genome size increase have been extensively studied, especially those referring to polyploidy and transposable element activity (Bennett and Leitch Citation2005c; Bennetzen et al. Citation2005; Hawkins et al. Citation2008). In addition, the amplification of repetitive DNAs (e.g. satellites and transposable elements) accounts for an important part of the genome sizes of eukaryotes (Kidwell Citation2002), as shown in plants with giant genomes (Ambrožová et al. Citation2011).
The Asteraceae are the largest angiosperm family, comprising c. 24,000 species described in 1,600–1,700 genera (Funk et al. Citation2009, and references therein). This fact, together with its cosmopolitan distribution (present across the world except in Antarctica; Funk et al. Citation2005) and the occurrence of many economically useful plants (Simpson Citation2009), has made this family the focus of a great deal of research in many fields of pure and applied plant science. Among these studies, a significant part has been devoted totally or partially to genome size assessments and analyses (Garnatje et al. Citation2007, and references therein), a field of work now covering a large range of biological aspects and dealing with most plant groups (Bennett and Leitch Citation2005a, Citation2005b, Citation2005c; Gregory Citation2005; Doležel et al. Citation2007, and references therein).
Starting from our own experience in this field and after a preliminary review of genome size studies in Asteraceae (Garnatje et al. Citation2007), we built a public database of Asteraceae genome sizes (accessible at www.asteraceaegenomesize.com; Garnatje et al. Citation2011). The database provides easy access to all the currently available information on nuclear DNA content in the family and it is regularly updated. Although the coverage of the family is still incomplete, the present study aims (1) to provide an overview of the current knowledge of nuclear DNA contents in Asteraceae; (2) to characterize evolutionary trends of genome size in this family, compared with other groups (families or genera) studied from this point of view; and (3) to identify key gaps in our knowledge and hence areas of focus for further research.
Materials and methods
The database “A genome size database in the Asteraceae” (GSAD, www.asteraceaegenomesize.com, release 2010 Garnatje et al. Citation2011), has been carefully combined with other ecological and systematic data (see database) available in the scientific literature to enable analysis of the current state of knowledge on nuclear DNA contents and to examine hypotheses relating to the systematic and evolutionary aspects of genome size variation in this botanical family. For systematic treatments and phylogenetic frameworks we have used the main reference studies in these fields for the family (Heywood et al. Citation1977; Hind et al. Citation1995; Caligari and Hind 1996; Hind and Beentje 1996; Kubitzki 2007; Funk et al. Citation2009).
To examine relationships between genome size and other parameters, the following statistical software was used: Excel 14.0 (Microsoft Office), XLSTAT (Addinsoft), Stata 10.0 (Stata Corporation). Regression analyses, and parametrical and non-parametrical statistical tests were carried out. In the genera with well-represented polyploid series in the database we investigated the relationship between genome size and chromosome number. Ancestral character states were generated with Mesquite v.2.75 (Maddison and Maddison 2011) using parsimony optimization for the genome size range per genus. To avoid the effect of polyploidy, this inference was done using monoploid genome sizes (1Cx), the quotient between genome size and ploidy level (where 1C is the abbreviated term for the holoploid genome size, i.e. the DNA amount of an unreplicated whole chromosome complement, Greilhuber et al. Citation2005). 1Cx mean values per genus were calculated by first determining the mean value for the species, with values for subspecies merged with those of the corresponding species and the data for hybrids considered separately as species. The tree used for the analyses was built using the phylogenies of Francisco-Ortega et al. (Citation2001), Garcia-Jacas et al. (Citation2001, Citation2006), Kimball and Crawford (Citation2004), Anderberg et al. (Citation2005), Hidalgo (2006), Hidalgo et al. (Citation2006), Susanna et al. (Citation2006), Oberprieler et al. (Citation2007), Pelser et al. (Citation2007) and Funk et al. (Citation2009) and irresolutions were used in order to avoid topology conflicts. Whether genome size might relate to life cycle was discussed comparing C values for annuals and their closest perennial relatives throughout Asteraceae lineages, using the phylogenies of Lee et al. (Citation2003), Samuel et al. (Citation2003, Citation2006), Susanna et al. (Citation2006), Pelser et al. (Citation2007), Enke (2008), Sanz et al. (Citation2008), Geleta et al. (Citation2010), Lo Presti et al. (Citation2010) and Sánchez-Jimenez et al. (2010).
Results and discussion
GSAD contains information on 1780 accessions, corresponding to genome size estimations for 110 genera and 820 species, and including 185 infraspecific taxa (Garnatje et al. Citation2011). It is clear from these data that the information on genome size in Asteraceae is still relatively scarce, as it covers approximately 6% of genera, 3% of species, 32.56% of tribes and 46.15% of subfamilies according to Funk et al.’s (Citation2009) classification. Figure presents the number of accessions and the percentage of each tribe with known genome size data. The data are biased to some degree, since the vast majority (96.74%) are concentrated in just five out of the 29 tribes recognized (Anthemideae, Cichorieae, Cardueae, Senecioneae, Heliantheae). However these five groups contribute around 50% to the species richness of the family (Funk et al. Citation2009).
Diversity and distribution of C values in Asteraceae
Holoploid nuclear DNA amount data range from 1C = 0.4 pg (Leontodon longirostris) to 1C = 28.3 pg (Coreopsis nuecensis), meaning an almost 70.75-fold variation within the family, whereas monoploid (1Cx) values vary from 0.18 (Pericallis appendiculata) to 10.45 (Chrysanthemum carinatum) pg. According to these data and using the classification for all angiosperms of Leitch et al. (1998), most Asteraceae genomes (57.23%) are considered to be very small or small (1C ⩽ 3.5 pg), with a mode of only 1.25 pg. Leitch et al. (1998) reported 51.70% of angiosperms as having 1C values equal or lower than 3.5 pg, which is within the range of the percentage currently found for the Asteraceae. In percentages, 20.30% of Asteraceae species have 1C values equal or lower than 1.4 pg, 36.93% between 1.4 and 3.5 pg (that is, 57.23% have 1C values very small or small, i.e. equal or lower than 3.5 pg) whereas only 1.78% have 1C values equal or larger than 14 pg. There is only one species (Chrysanthemum lacustre, a perennial high polyploid with 2n = 22x = 198) that has been reported with a 1C- value exceeding 35 pg (71 pg, Baetcke et al. Citation1967). However, although data concerning C. lacustre were included in our analyses, they could be considered unreliable because DNA amount was estimated using chemical extraction techniques including cells at all stages of the cell cycle. In addition, C. lacustre is now considered a synonymous of Leucanthemum vulgare for which genome size estimates (1C) range from 5 to 16 pg and the highest chromosome count for this taxon is 2n = 72 (IPCN, http://www.tropicos.org/Project/IPCN). Table provides genome size data for the ten most variable genera based on the maximum/minimum C value-ratio, and Table gives similar information for all tribes with genome size data. In Table S1 (Supplementary Material available via the article webpage), genome size information (maximum, minimum, mean, ratio maximum/minimum and number of accessions of 1C and 1Cx values) is listed for all the genera included in the database. The 1Cx values are higher than the 1C values in some genera due to the lack of chromosome counts for some accessions.
Figure 1 Diagram of the percentages of data included in GSAD (www.asteraceaegenomesize.com) grouped into tribes.
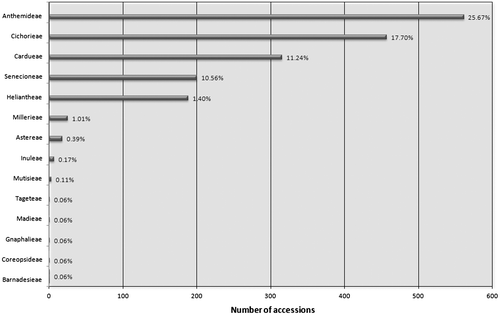
Table 1. Minimum, maximum, mean of 1C (in bold) and 1Cx values, and number of species with C values for 10 genera of Asteraceae with the highest maximum/minimum ratio (data obtained from www.asteraceaegenomesize.com, release 2010). For full details see Table S1 in the Supplementary Material available via the article webpage. Genera are arranged by decreasing range.
Table 2. Minimum, maximum, mean of 1C and 1Cx values, number of species measured for the tribes of Asteraceae included in www.asteraceaegenomesize.com, release 2010). The 1C values are in bold.
The analysis of genome size evolution in Asteraceae shows its dynamics at the 1Cx level, with gains and losses distributed throughout the phylogenetic tree (Figure ). This is likely to be even more accentuated at the 1C level as it is not adjusted for ploidy. Although ancestral values for basal nodes remain equivocal, inference analyses showed that most species with small 1Cx values and almost all with intermediate ones are probably derived from ancestors with very small 1Cx values (Figure ).
Figure 2 Ancestral genome sizes reconstructed using Mesquite v.2.75 (Maddison and Maddison 2011). Pie charts at nodes represent the probability of the ancestral state inferences, corresponding to the parsimony optimization for the 1Cx range per genus. Note that most small and intermediate 1Cx values are derived from nodes showing very small genome sizes (white).
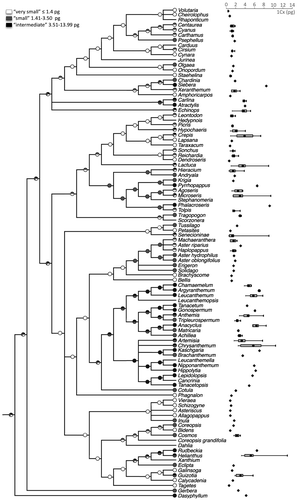
At the tribal level, the Anthemideae have the highest mean genome size value (1C = 5.97 pg), with the values ranging 14.19-fold (Table ). Despite the fact that more genome size records are available for tribe Anthemideae than for other tribes (Figure ), its range is considerably lower than those for tribes Senecioneae and Cichorieae (47.55-fold and 39.13-fold, respectively). This trend is also found at the generic level (Table ): for instance, in the genus Senecio (tribe Senecioneae), with ploidy levels reported up to 10x, a 1C genome size variation of 45.09-fold has been found, whereas the intensively sampled genus Artemisia in tribe Anthemideae, with a polyploid series up to 16x, shows an only 7.73-fold range in 1C values. These contrasting results between genera and tribes (i.e. Senecio showing a substantial range compared with Artemisia) might reflect different genomic responses to polyploidization. In Senecio the subsequent genomic reorganizations may be additive with little DNA loss (as detected, among many others, in the Asteraceae genus Carthamus; Garnatje et al. Citation2006), whilst in Artemisia changes following polyploidization may be more erosive, leading to a significant genome downsizing in its polyploids (already found in a reduced group of American endemic Artemisia; Garcia et al. Citation2008; Pellicer et al. 2010). Leitch et al. (Citation2008) related such differences in the direction of genome size change across polyploid series with their age, although without finding a discernible pattern. For all the genera with data available (Table S1), the ratio between maximum and minimum genome size values range from 1.00 (in many genera, often due to scarce – or a single – data) to 45.09 for 1C, and from 1.00 to 10.91 for 1Cx, Senecio in both cases showing the maximum ratio. Tribes Anthemideae, Cardueae, Cichorieae, Coreopsideae and Senecioneae show the largest genome size ranges (Table ), of which Cichorieae and Senecioneae present the highest dispersion of values. These are among the tribes in which genome sizes for more than 20 species have been reported to date.
Considering the angiosperms as a whole, Table summarizes genome size data compiled in the GSAD database (Garnatje et al. 2010, 2011) and the angiosperm C values database in four of the largest angiosperm families in terms of species number (Asteraceae, Leguminosae, Poaceae and Orchidaceae; Bennett and Leitch 2010). We have selected these four families because they represent monocot/eudicot lineages and the percentage of genome size estimations is comparable. At first sight we observe that Poaceae and Orchidaceae are more variable (103.88 and 167.87-fold in 1C values, respectively) than Asteraceae and Leguminosae (70.75 and 84.70-fold, respectively). However, this difference becomes less evident when we look at the 1Cx values (58.05, 56.15 and 62.72 for Asteraceae, Leguminosae and Poaceae, respectively), indicating that polyploidy actually accounts for most of their genome size variation.
Table 3. Genome size data in four of the main angiosperm families (Asteraceae, Leguminosae, Poaceae and Orchidaceae).
Correlations with chromosome numbers, ploidy levels and heterochromatin and/or ribosomal DNA loci
Figures present the distribution of 1C values and 2n chromosome numbers, respectively, for species with available genome size data. Figure clearly illustrates that most species in Asteraceae with available nuclear DNA amounts (> 90%) have 1C values lower than 10 pg, and a majority (57.23%) are equal or lower than 3.5 pg, as previously mentioned. The preponderance of x = 9 as the basic chromosome number in the family (Semple and Watanabe Citation2009) is reflected in Figure , as the chromosome numbers for most genome size estimates are multiples of nine (i.e. 18, 36, 54) with the diploid level (2n = 18) being by far the most frequent. It is noted that the abundant Artemisia information in the database, the most common basic chromosome number of which is also x = 9, contributes considerably to making accessions with a multiple of nine predominant.
Figure 3 Histogram showing the distribution of 1C values for species listed in the GSAD (www.asteraceaegenomesize.com).
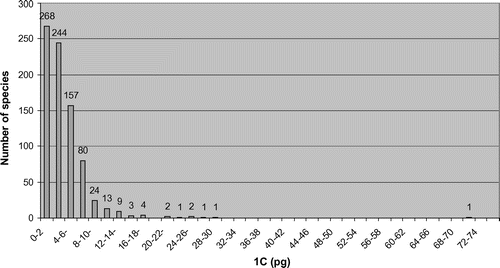
Figure 4 Number of accessions per chromosome number (2n) (indicated above the bars). Data taken from GSAD (www.asteraceaegenomesize.com).
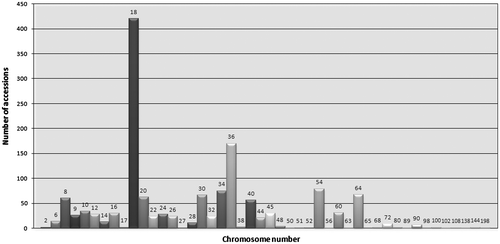
A highly significant positive correlation between chromosome number and 2C values (p < 0.0001) has been found in the family (Figure ), as noted by researchers for some other families (e.g. Orchidaceae; Jones et al. Citation1998). However, in other groups, chromosome number is not a predictor of genome size; indeed, previous studies found a negative correlation between chromosome number and genome size (in several angiosperm groups or in threatened plants; Vinogradov Citation2001, Citation2003) or no correlation at all (in bryophytes; Renzaglia et al. Citation1995).
Mean DNA amount per chromosome
The database holds genome size data for species which have a 49.5-fold variation in chromosome number, from 2n = 2x = 4 (Brachyscome dichromosomatica and Haplopappus gracilis) to 2n = 22x = 198 (Chrysanthemum lacustre). Several patterns in the evolution of mean DNA amount per chromosome (2C/2n) in the five best represented tribes of Asteraceae are observed (Figure ). The largest mean DNA amounts per chromosome are found in Anthemideae (Leucanthemum lineare, 2.25 pg per chromosome) and Cichorieae (Crepis grandiflora, 1.63 pg per chromosome) with all the other tribes being characterized by much smaller chromosomes (in terms of genome size, not in terms of length). Anthemideae and Cichorieae also present a similar range in 2C/2n values, but these show different patterns of distribution, suggesting that evolutionary processes driving chromosome DNA amount and genome size evolution behave differently in these tribes. Our results suggest that Cichorieae may undergo more relaxed chromosome genome size evolution than other Asteraceae tribes, as its mean range is wider and data are evenly distributed. On the other hand, Senecioneae and Heliantheae may have more severe constraints as regards chromosome genome size. Similar results were found by Leitch et al. (2010) in several subfamilies of Poaceae.
Figure 5 Linear regression between genome size (Y) and chromosome number (X) including 95% confidence intervals. The relationship between both variables is best fitted by the straight line: Y = –7.1591 + 0.4121X; R2 = 0.613 (p < 0.0001). Data taken from GSAD (www.asteraceaegenomesize.com).
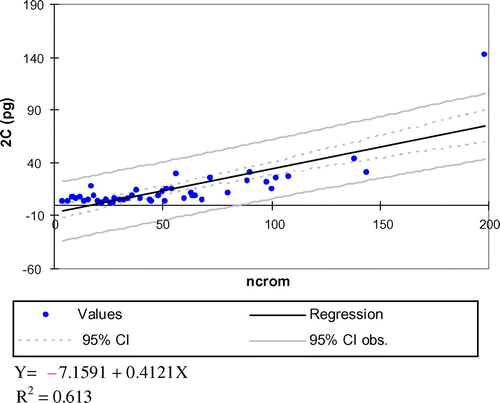
Figure 6 Scatter plot of mean DNA amount per chromosome (2C/2n) for five well-represented tribes in the database (www.asteraceaegenomesize.com). Horizontal grey stripes indicate the arithmetic mean of all 2C/2n values for each tribe.
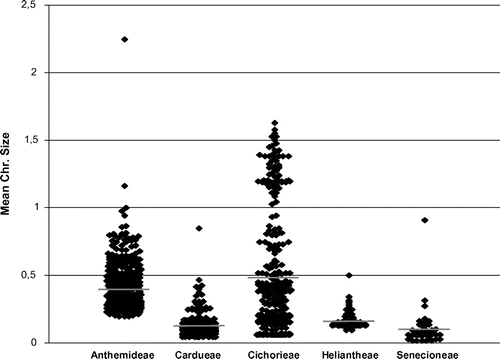
Polyploidy and dysploidy
From the data available in the database, 25.5% of the species belong to polyploid or presumed polyploid taxa. Polyploidy is a well-known and widespread phenomenon reported in many plant complexes (Leitch and Bennett Citation2004) including Asteraceae (e.g. Vallès et al. Citation2011, Citation2012; Meng et al. Citation2012). Due to the increasing number of genome size surveys published, new ploidy levels have been discovered in many plant groups (e.g. Hidalgo and Vallès Citation2012; Romo et al. 2013), therefore evidencing different patterns of genome size evolution following polyploidization. For example, an additive pattern was encountered in polyploid Carthamus species (Garnatje et al. Citation2006). Artemisia however generally shows a general downsizing (Pellicer et al. Citation2010, and references therein), although cases were found where polyploidy was not accompanied by DNA loss, such as in some members of the subgenus Tridentatae (Garcia et al. Citation2009) and some South American endemics (Pellicer et al. 2010). The absence of genome downsizing has been attributed in some taxa to the relatively young age of the polyploids, based on the assumption that nuclear DNA rearrangements accumulate through time and may be mostly accompanied by DNA loss (Leitch and Bennett Citation2004; Leitch et al. Citation2008; Pellicer et al. 2010). Nevertheless, Pires et al. (Citation2004) detected an additive pattern of the diploid parents in genome size of the recent tetraploid Tragopogon mirus and downsizing in some populations of tetraploid T. miscellus, in both cases without evidence of major genomic reshuffling. Finally, no cases of genome upsizing have been detected up to now in Asteraceae, although this has been described in ancient Nicotiana (Solanaceae) polyploids and a few other genera (Leitch et al. Citation2008).
At genus level, the function that best describes genome size variation with increasing ploidy level in Artemisia is the Michaelis–Menten function, with an asymptotic behavior leading one to speculate about the existence of an upper limit in the DNA content (Pellicer et al. Citation2010). The remaining genera with polyploid series and enough genome size data available do not show a constant pattern describing the relationship between both variables. Linearity can only be discarded in Carthamus (p = 0.000), Hieracium (p = 0.000) and Tripleurospermum (p = 0.000), and quadratic function is obtained for all of them, but these results are not compatible with any biological explanation. Further analyses are necessary to establish a putative genome size limit, if any, for different Asteraceae genera and to compare the patterns shown for them. Within the Asteraceae, some genera, such as Centaurea, which has several ploidy levels, are especially appropriate to test the existence of this upper limit and to compare their results with those of genera with only one ploidy level, such as Cheirolophus or Rhaponticum. However, for these studies it is necessary to fill the gaps in genome size measurements in the family.
Dysploidy, which leads to either a reduction or increase of the chromosome number usually through chromosomal rearrangements (fissions and fusions), tends to take place without causing considerable variations in the DNA amount (Lavia and Fernández Citation2008). In dysploid genera such as Dahlia or Reichardia, populations with a different chromosome number (of 64–65 and 14–16 chromosomes, respectively) have similar genome sizes (4.41–4.36 pg and 0.75–0.74 pg, for the species Dahlia coccinea and Reichardia picroides, respectively). Regardless, there are some cases in the Asteraceae that deserve attention, such as the reduction in genome size with strong descending dysploidy observed within the Rhaponticum group (Hidalgo et al. Citation2007, Citation2008).
rDNA loci number and ribosomal DNA structure
Ribosomal DNA, both the 5S and the 18S-5.8S-26S (onwards 35S) are one kind of tandemly repeated DNA (also called satellite DNA), and are a characteristic feature of eukaryotic genomes. Since satellite repeats can extensively expand and contract, this may cause genome size variation among related species. As with genome size, there is also considerable variation between plant species in the number of rRNA genes and loci (Rogers and Bendich Citation1987). Prokopowich et al. (Citation2003) reported that ribosomal DNA, as a highly repetitive gene family, was correlated to some degree with overall genome size; several authors have debated a possible relationship between the amount of nuclear DNA and ribosomal DNA (Maher and Fox Citation1973; Gall Citation1981; Lamppa et al. Citation1984; Garnatje et al. Citation2004; Garcia et al. Citation2009).
The Asteraceae has been particularly well studied regarding ribosomal DNA (Garcia et al. Citation2010), and this has enabled comparative studies to be made. We have analyzed statistically the relationship between rDNA loci number and genome size in the Asteraceae species with the available data. In total, 96 taxa (contained in the GSAD database) have been assessed, including data from several papers dealing with their molecular cytogenetics (Garcia et al. Citation2012).
Considering all taxa and all ploidy levels together, the total number of ribosomal DNA loci is positively and significantly correlated (p = 0.000) with genome size (2C), that is, species with larger genome sizes tend to present more rDNA loci numbers, although the increase is not directly proportional. Separately, 5S and 35S rDNA loci numbers are also significantly correlated with 2C (p < 0.0001 and p = 0.007, respectively). When only diploid taxa are considered, to overcome the effect of polyploidy, the results are the same (p < 0.01). This is consistent with previous studies stating that in general, species with smaller genomes tend to contain fewer copies of these genes (Lamppa et al. Citation1984) or a lower number of loci (Leitch and Bennett Citation2004; Garcia, Garnatje et al. Citation2009) than those with larger genomes, although these are usually not proportional to genome size.
This trend is also found when focusing on certain genera, e.g. Artemisia (p < 0.0001, 27 accessions) or Centaurea (p < 0.0001, 11 accessions). However, when just diploids or all ploidy levels were analyzed for these genera the positive relationship between genome size and overall number of rDNA loci was only significant for diploid Artemisia (p = 0.009). For the genera Cheirolophus (seven accessions, all diploids with 6–10 rDNA loci; Garnatje et al. Citation2012) and Hypochaeris (13 accessions) no relationship between genome size and number of rDNA loci was found. In genus Silene (Caryophyllaceae), Siroky et al. (Citation2001) detected a marked variation in the number and position of rDNA loci but no correlation between the number of rDNA sites and genome size of the studied species. Similarly, Cai et al. (Citation2006) were unable to find a significant correlation between rDNA loci number and genome size in species from the gymnosperm genus Pinus, but observed more rDNA loci in the subgenus Strobus, which is considered to have undergone an increase in genome size relative to other subgenera. Overall, our results point to a general positive correlation between the number of rDNA loci and genome size, but more studies are necessary in other plant groups to analyze the data within a phylogenetic context to avoid any effects of evolutionary history, not only to confirm or reject such an association but also for hypothesizing on its possible causes.
Two major types of organization of ribosomal DNA have been reported in Asteraceae (Garcia et al. Citation2010). The typically unlinked in which the 5S and 35S rDNA clusters are located separately (S-type) or when the 5S and 35S rDNA sites are linked (L-type) in a single operon (Garcia et al. 2009). This peculiar rDNA arrangement has been related to the activity of a Cassandra-type retrotransposon (Kalendar et al. Citation2008). Since plant genomes undergo genome size increases through bursts of retrotransposition (Vitte and Panaud Citation2005), we tested if there was a significant difference between genome sizes of L-type or S-type species. The results show that L-type species tend to have larger genome sizes (p < 0.0000). The analysis could be biased, however, because phylogenetic relationships between species were not taken into account (due to the scarcity of data). While other active transposable element families may be present both in L-type or S-type species, it is possible that the larger genomes observed in the L-type species bear some relationship with the activity of this particular Cassandra-type retroelement.
Genome size, reproductive system, life cycle and ecological conditions
Correlations between nuclear DNA amount and ecological aspects of plant life (in a broad sense, including for instance propagation types, life cycle and invasive potential) have been widely tested and discussed in Asteraceae. In a work focused on Microseris bigelovii, Price et al. (Citation1981) postulated that smaller genomes may have resulted from selection for low DNA content in stressed and/or time-limited environments. Bancheva and Greilhuber (Citation2006) analyzed the relationships between genome size and parameters such as altitude, precipitation and temperature in Bulgarian Centaurea s.l., finding that whereas altitude and precipitation were positively and significantly correlated with genome size, temperature showed a negative correlation. Suda et al. (Citation2003) found a positive correlation between altitude and genome size in Sonchus but negative in Argyranthemum and Microseris, although these findings were not confirmed in a larger sample (Suda et al. Citation2005).
Reproductive system
While there are few data available regarding this important plant characteristic, certain groups of Asteraceae seem to show an increased genome size in plants with vegetative propagation. For example, within the various Turkish tetraploid species of Tripleurospermum, the rhizomatous ones were found to have a significantly higher nuclear DNA amount than those without rhizomes (Garcia et al. Citation2005). In this context it is thus perhaps not surprising that the largest genome size found in Artemisia (Pellicer et al. Citation2010) – associated with the highest ploidy level in the genus, 16x – belongs to a population of a species (A. medioxima) showing evidence of vegetative multiplication by rhizomes. Rees and Jones (Citation1967) also observed a larger genome size in a species of annual Lolium (L. temulentum) with vegetative reproduction than in a perennial species (L. perenne) which undergoes sexual reproduction. However, results are not conclusive about a typical trend of genome size expansion linked to vegetative propagation, as other studies have reported that smaller rather than larger genome sizes are linked to vegetative reproduction: this is the case of a couple of species in the genus Veronica (Albach and Greilhuber Citation2004).
Life cycle
Nuclear DNA amounts in annual and perennial species have been compared in numerous studies concerning all groups of vascular plants, although no general conclusions have emerged. Besides the relevance of life cycle for adaptations to ecological parameters, its relationship with genome size is at least also partly determined by the duration of the cell cycle, as earlier stated by Bennett (Citation1972). After analyzing a set of both dicotyledons and monocotyledons, this author indicated that the mean nuclear DNA content of annual species was significantly lower than that of perennial species. Bennett (Citation1976) and Lawrence (Citation1985) found that the DNA amount in plants was related to both minimum generation time and life cycle type. Other classical studies [Van’t Hof and Sparrow (Citation1963) and Van’t Hof (1965)] which include an important group of Asteraceae from the genus Crepis, found in most groups studied smaller genome sizes in annual species, which have a much shorter life cycle and need a faster cell division rate. Indeed, large amounts of DNA are usually associated with large and slowly dividing cells (Gregory Citation2001); in this sense, plants with large genomes (1C >25 pg) are restricted to being obligate perennials – as is the case for all Asteraceae investigated here with large genomes – or to particular habitats (Leitch et al. Citation2007, and references therein). A lower genome size in annuals than in perennials is frequently found, agreeing with the constraints of large genomes at evolutionary, ecological and functional levels (Knight et al. Citation2005).
In an investigation centered in some Anthemideae genera, Nagl and Ehrendorfer (Citation1974) found that annuals may have either a lower or a much higher DNA content, and usually more heterochromatin than perennials, that is, annuals tend to go for “extreme” genome size values. Artemisia leucodes and Siebera pungens are two annual species that present very high nuclear DNA amounts as compared with other annuals (and even with perennials) of their groups (Garcia et al. Citation2004; Garnatje et al. Citation2004, respectively; see Figure S1 in Supplementary Material available via the article webpage), while other closely related annuals do have very low genome sizes (such as Artemisia annua; Torrell and Vallès Citation2001; Figure S1). Furthermore, analysis of genome size data currently available in GSAD indicates larger mean genomes in annuals than in perennials. Our present survey of Asteraceae genome size confirms the values for annuals to be from much lower to much higher than perennial relatives, and that opposite trends can be found within a same group of closely related annuals (Figure S1).
Continental and insular taxa
A relatively recent observation concerning genome size is its apparent decrease in some plant groups during the colonization of insular habitats for some taxa (Suda et al. Citation2005, Citation2007; Garnatje, Garcia, and Canela 2007). This fact has been verified in plant groups with both insular and continental representatives, in which the molecular phylogeny clearly shows the colonization events occurred from the continent to the islands. Particularly in the Asteraceae, some examples clearly illustrate this theory. In genus Cheirolophus (Garnatje, Garcia, and Canela 2007) a remarkable decrease in nuclear DNA content has been observed in the insular taxa with respect to their continental relatives. Given these observations, it is unclear whether this decrease is due to selection pressures exerted by the insular habitats on genome size or to the fact that the species that have colonized oceanic islands tend to be more phylogenetically derived, and may have undergone genome downsizing prior to colonization, perhaps making them pre-adapted to colonization (Garnatje, Garcia, and Canela 2007; Garnatje et al. Citation2012).
Ecological strategies
MacArthur and Wilson (Citation1967) proposed the classification of plants into K and r strategists to distinguish those slow growing and with a low seed production, occupying stable habitats (K), from fast growing plants, producing large amounts of seeds and capable of rapid colonization of disturbed habitats (r). Genome size data offer interesting arguments regarding this classification in some plant groups, such as the North American endemic subgenus Tridentatae of genus Artemisia (Garcia et al. Citation2008), in which species showing a more r strategist profile tend to have significantly lower genome sizes than those that would appear to be more K strategists. However, it is difficult to categorize the majority of species as there is a continuous spectrum between the two categories (i.e. most species are not clearly K or r). Although some annual Artemisia species, such as A. annua and A. scoparia, would also fit well within the category of the r strategy (short life cycles, profuse seed production, etc.) and have low genome sizes as compared to their counterparts, exceptions exist that prevent generalizations (i.e. the case of A. leucodes, an annual with a particularly large DNA amount of 1C = 7.7 pg; Garcia et al. Citation2004).
The r strategists are often considered weeds. Many weeds have a lower DNA amount than related species, as Bennett et al. (Citation1998) showed for a large set of taxa, including, among others, some representatives of the Asteraceae. These authors hypothesize that the possibility of a plant becoming a weed drastically diminishes as its genome size increases (with no weeds observed with genomes larger than 1C = c.25 pg), and that a small genome size would therefore favor a weedy behavior. Among the Asteraceae species listed as weeds in the Global Invasive Species Database (http://www.issg.org/database/welcome/), where genome size data are available (Table ), the mean 1C value of diploid weeds is 1.30 pg, and the mean of polyploids is 2.17 pg, the global mean being 1.65 pg. That is, even including polyploid weeds, genome sizes would still be considered small or very small, according to the Leitch et al. (1998) categories.
Table 4. Species listed at the Global Invasive Species Database (http://www.issg.org/database/welcome/) from Asteraceae with known genome sizes.
Genome size and systematics
Although a certain degree of genuine variation is accepted (Garcia et al. Citation2006; Garnatje et al. Citation2006, Citation2009; Pellicer et al. Citation2009), genome size in a given species is considered to be stable (Greilhuber Citation2005) and considerable infraspecific variation may indicate possible incipient speciation; thus, 1C values may in some cases be used as a systematics tool at the species level, capable of indicating taxonomic heterogeneity, and pointing to the need for a re-evaluation of the delimitation of the taxon in question (Murray Citation2005). Dimitrova and Greilhuber (Citation2000) used the variation in genome size in closely related taxa to justify the recognition of Crepis foetida subsp. commutata as a specific taxon, C. commutata. Its genome size was about 10% lower than those of the other two subspecies, which had similar genome sizes. On a similar basis, Garcia et al. (Citation2009) excluded from the core of subgenus Tridentatae three taxa, Artemisia bigelovii, A. pygmaea, and A. rigida, whose DNA content was clearly out of the range of the mentioned group, a decision which was later corroborated by a complete molecular phylogenetic analysis, including both nuclear and plastid markers (Garcia et al. Citation2011).
Needs for further research
The overview of genome size data available in GSAD highlights the uneven taxonomic coverage. Just five tribes are well represented, nine tribes have very scarce data (Figure ), and 29 tribes still have no data at all (although the majority of these have few species and thus do not represent a significant proportion of the family as a whole). One of the tribes needing further research effort in particular is the Barnadesieae, as it is the only one in the subfamily Barnadesioideae, and there are no genome size records for any of its nine genera and 91 species (Stuessy et al. Citation2009). In addition, if we consider that only three genome size estimates are available for the tribe Mutisieae, and there are none for the other two subtribes comprising the subfamily Mutisioideae or for the whole subfamily Wunderlichioideae, it is clear that for a better understanding of genome size evolution in the Asteraceae there is a need for more in-depth genome size studies in these early diverging clades of the family. Only the subfamily Carduoideae is relatively well known (but only through the tribe Cardueae) in this part of the phylogenetic tree. Finally, it is reiterated that for any study into genome size diversity and evolution in a family as diverse as Asteraceae in many aspects (e.g. karyology, taxonomy, etc.), it is essential that the data are viewed within a phylogenetic framework.
Concluding remarks
Although genome size data are still available for only a small part of the Asteraceae, and in a somewhat unrepresentative form, their analysis, made easy by GSAD (Garnatje et al. Citation2011), permits us to postulate some hypotheses about systematic, phylogenetic and evolutionary aspects of the genome size diversity encountered. Several patterns have been found across the plant phylogeny, a general pattern of genome downsizing from the gymnosperms to the angiosperms and from monocots to eudicots. In this sense, the genome sizes of the Asteraceae family, one of the most evolved angiosperm families, are considered to be from very small to small, thus agreeing with its phylogenetic position.
Karyological and cytogenetic characteristics, such as polyploidy and rDNA loci, are better correlated with the genome size than ecological characteristics. Several patterns have been found, however, for the reproductive systems, life cycles and ecological strategies.
The analysis of the above-mentioned database also helped us to detect some gaps in the knowledge of Asteraceae genome sizes, highlighting taxonomic groups in need of more research, in order to achieve a more representative phylogenetic coverage. This will enable us to confirm, suggest or discard the present conclusions and allow a better understanding of the role that genome size plays in the biology of one of the largest angiosperm families.
tcar_a_829690_sm0508.doc
Download MS Word (246 KB)tcar_a_829690_sm9415.tif
Download TIFF Image (1.2 MB)Acknowledgments
The authors thank researchers who contributed Asteraceae genome size assessments and studies, Dr. Ilia Leitch, Samuel Pyke and two anonymous reviewers for improving the manuscript, and Alba Anadon and Francisco Gálvez for technical support. This work has been supported by DGICYT (Spanish Government; projects CGL2007-64839-C02-01/BOS, CGL2007-64839-C02-02/BOS, CGL2010-22234-C02-01/BOS and CGL2010-22234-C02-02/BOS) and the Generalitat de Catalunya (“Ajuts a grups de recerca consolidats” 2005SGR00344 and 2009SGR0439). I.S-J. and D.V. benefited from a FPU predoctoral grants. O.H. benefitted from a Juan de la Cierva postdoctoral contract of the Ministry of Science and Innovation of the Spanish government, and S.G. benefitted from a Beatriu de Pinós postdoctoral contract of the Comissionat per a Universitats i Recerca (CUR), Departament d’Economia i Coneixement de la Generalitat de Catalunya (Catalonian government).
References
- Albach DC, Greilhuber J. 2004. Genome size variation and evolution in Veronica. Ann Bot. 94 (6): 897–911.
- Ambrožová K, Mandáková T, Bureš P, Neumann P, Leitch IJ, Koblížková A, Macas J, Lysak MA. 2011. Diverse retrotransposon families and an AT-rich satellite DNA revealed in giant genomes of Fritillaria lilies. Ann Bot. 107 (2): 255–258.
- Anderberg AA, Eldenäs P, Bayer RJ, Englund M. 2005. Evolutionary relationships in the Asteraceae tribe Inuleae (incl. Plucheeae) evidenced by DNA sequences of ndhF; with notes on the systematic positions of some aberrant genera. Org Divers Evol. 5 (2): 135–146.
- Baetcke KP, Sparrow AH, Nauman CH, Schwemmer SS. 1967. The relationship of DNA content to nuclear and chromosome volumes and to radiosensitivity (LD50). Botany. 58 (2): 533–540.
- Bancheva S, Greilhuber J. 2006. Genome size in Bulgarian Centaurea s.l. (Asteraceae). Plant Syst Evol. 257 : 95–117.
- Bennett MD. 1972. Nuclear DNA content and minimum generation time in herbaceous plants. Proc R Soc B. 181 (1–2): 109–135.
- Bennett MD. 1976. DNA amount, latitude and crop plant distribution. Environ Exp Bot. 16 (2–3): 93–108.
- Bennett MD. 1998. Plant genome values: how much do we know? Proc Natl Acad Sci USA. 95 (5): 2011–2016.
- Bennett MD, Leitch IJ. 2005a. Plant genome size research: a field in focus. Ann Bot. 95 (1): 1–6.
- Bennett MD, Leitch IJ. 2005b. Nuclear DNA amounts in angiosperms: progress, problems and prospects. Ann Bot. 95 (1): 45–90.
- Bennett MD, Leitch IJ. 2005c. Genome size evolution in plants. In: Gregory TR, ed. The evolution of the genome. San Diego (CA): Elsevier, 89–162.
- Bennett MD, Leitch IJ. 2010, Dec. Plant DNA C-values database [database release 5.0]. http://data.kew.org/cvalues/.
- Bennett MD, Leitch IJ. 2012, Dec. Plant DNA C-values database [database release 6.0]. http://data.kew.org/cvalues/.
- Bennett MD, Leitch IJ, Hanson L. 1998. DNA amounts in two samples of angiosperms weeds. Ann Bot. 82 (Suppl. 1): 121–134.
- Bennetzen JL, Ma JX, Devos KM. 2005. Mechanism of recent genome size variation in flowering plants. Ann Bot. 95 (1): 127–132.
- Bowers JE, Chapman BA, Rong J, Paterson AH. 2003. Unravelling angiosperm genome evolution by phylogenetic analysis of chromosomal duplication events. Nature. 422 (6930): 433–438.
- Cai Q, Zhang D, Liu ZL, Wang XR. 2006. Chromosomal localization of 5S and 18S rDNA in five species of subgenus Strobus and their implications for genome evolution of Pinus. Ann Bot. 97 (1): 715–722.
- Caligari PDS, Hind DJN, D.J.N. Hind (Editor-in-Chief). 1996. Compositae: Biology & utilization. In: Proceedings of the International Compositae Conference, Kew, 1994, vol. 2. Royal Botanic Gardens, Kew.
- Cui L, Wall PK, Leebens-Mack JH, Lindsay BG, Soltis DE, Doyle JJ, Soltis PS, Carlson JE, Arumuganathan K, Barakat A, Albert V, Ma H, dePamphilis CW. 2006. Widespread genome duplications throughout the history of flowering plants. Genome Res. 16 (6): 738–749.
- Dimitrova D, Greilhuber J. 2000. Karyotype and DNA-content evolution in ten species of Crepis (Asteraceae) distributed in Bulgaria. Bot J Linn Soc. 132 (3): 281–297.
- Doležel J, Greilhuber J, Suda J. 2007. Flow cytometry with plant cells: analysis of genes, chromosomes and genomes. London: Wiley-VCH.
- Enke N. 2008. Phylogeny and character evolution in the genus Crepis L. (Cichorieae, Compositae) [dissertation zur Erlangung des akademischen Grades des Doktors der Naturwissenschaften (Dr. rer. nat.) eingereicht im Fachbereich Biologie, Chemie, Pharmazie]. [Berlin]: Freien Universität. 129 p.
- Francisco-Ortega J, Park SJ, Santos-Guerra A, Benabid A, Jansen K. 2001. Origin and evolution of the endemic Macaronesian Inuleae (Asteraceae): evidence from the internal transcribed spacers of nuclear ribosomal DNA. Bot J Linn Soc. 72 (1): 77–97.
- Funk VA, Bayer RJ, Keeley S, Chan R, Watson L, Gemeinholzer B, Schilling E, Panero JL, Baldwin BG, Garcia-Jacas N, Susanna A, Jansen RK. 2005. Everywhere but Antarctica: using a supertree to understand the diversity and distribution of the Compositae. In: Friis I and Balslev H, editors. Biologiske Skrifter. 55 : 343–373.
- Funk VA, Susanna A, Stuessy TF, Bayer RJ, editors. 2009. Systematics, evolution and biogeography of Compositae. Vienna: International Association for Plant Taxonomy.
- Gall JG. 1981. Chromosome structure and the C-value paradox. J Cell Biol. 91 (3): 3–14.
- Garcia S, Canela MÁ, Garnatje T, McArthur ED, Sanderson SC, Vallès J. 2008. Evolutionary and ecological implications of genome size in the North American endemic sagebrushes and allies (Artemisia, Asteraceae). Biol J Linn Soc. 94 (3): 631–649.
- Garcia S, Garnatje T, Kovarik A. 2012. Plant rDNA database: ribosomal DNA loci information goes online. Chromosoma. doi:10.1007/s00412-012-0368-7. 121(4):389–394.
- Garcia S, Garnatje T, Pellicer J, McArthur ED, Siljak-Yakovlev S, Vallès J. 2009. Ribosomal DNA, heterochromatin and correlation with genome size in diploid and polyploid North American endemic sagebrushes (Artemisia, Asteraceae). Genome. 52 (12): 1012–1024.
- Garcia S, Garnatje T, Twibell JD, Vallès J. 2006. Genome size variation in the Artemisia arborescens complex (Asteraceae, Anthemideae) and its cultivars. Genome. 49 (3): 244–253.
- Garcia S, Inceer H, Garnatje T, Vallès J. 2005. Genome size variation in some representatives of the genus Tripleurospermum. Biol Plantarum. 49 (3): 381–387.
- Garcia S, Lim KY, Chester M, Garnatje T, Pellicer J, Vallès J, Leitch AR, Kovarik A. 2009. Linkage of 35S and 5S rRNA genes in Artemisia (family Asteraceae): first evidence from angiosperms. Chromosoma. 118 (1): 85–97.
- Garcia S, McArthur ED, Pellicer J, Sanderson SC, Vallès J, Garnatje T. 2011. A molecular phylogenetic approach to Western North America endemic Artemisia and allies (Asteraceae): untangling the sagebrushes. Am J Bot. 98 (4): 638–653.
- Garcia S, Panero JL, Siroky J, Kovarik A. 2010. Repeated reunions and splits feature the highly dynamic evolution of 5S and 35S ribosomal RNA genes (rDNA) in the Asteraceae family. BMC Plant Biol. 10 : 176.
- Garcia S, Sanz M, Garnatje T, Kreitschitz A, McArthur ED, Vallès J. 2004. Variation of DNA amount in 47 populations of the subtribe Artemisiinae and related taxa (Asteraceae, Anthemideae): karyological, ecological, and systematic implications. Genome. 47 (6): 1004–1014.
- Garcia-Jacas N, Susanna A, Garnatje T, Vilatersana R. 2001. Generic delimitation and phylogeny of the subtribe Centaureinae (Asteraceae): a combined nuclear and chloroplast DNA analysis. Ann Bot. 87 (4): 503–515.
- Garcia-Jacas N, Uysal T, Romaschenko K, Suárez-Santiago VN, Ertuğrul K, Susanna A. 2006. Centaurea revisited: a molecular survey of the Jacea group. Ann Bot. 98 (4): 741–753.
- Garnatje T, Canela MÁ, Garcia S, Hidalgo O, Pellicer J, Sánchez-Jiménez I, Siljak-Yakovlev S, Vitales D, Vallès J. 2010. GSAD: a database on genome size of the family Asteraceae [database]; [cited 2010 Sep 30]. Available from http://www.etnobiofic.cat/gsad/.
- Garnatje T, Canela MÁ, Garcia S, Hidalgo O, Pellicer J, Sánchez-Jiménez I, Siljak-Yakovlev S, Vitales D, Vallès J. 2011. GSAD: a genome size in the Asteraceae database. Cytom Part A. 79A (6): 401–404.
- Garnatje T, Garcia S, Canela MA. 2007. Genome size variation from a phylogenetic perspective in the genus Cheirolophus Cass. (Asteraceae): biogeographic implications. Plant Syst Evol. 264 (1–2): 117–134.
- Garnatje T, Garcia S, Hidalgo O, Pellicer J, Sánchez-Jiménez I, Vallès J. 2009. Cheirolophus intybaceus (Asteraceae, Centaureinae) o la constància del valor 2C. Collect Bot (Barcelona). 28 : 1–11.
- Garnatje T, Garcia S, Hidalgo O, Pellicer J, Vallès J. 2007. Genome size in the Asteraceae: state-of-art and perspectives. Nucleus. 50 (3): 427–438.
- Garnatje T, Garcia S, Vilatersana R, Vallès J. 2006. Genome size variation in the genus Carthamus L. (Asteraceae, Cardueae): systematic implications and additive changes during allopolyploidization in hybrid taxa. Ann Bot. 97 (3): 461–467.
- Garnatje T, Hidalgo O, Vitales D, Pellicer J, Vallès J, Robin O, Garcia S, Siljak-Yakovlev S. 2012. Swarm of terminal 35S in Cheirolophus (Asteraceae, Centaureinae). Genome. 55 (7): 1–7.
- Garnatje T, Vallès J, Garcia S, Hidalgo O, Sanz M, Canela MA, Siljak-Yakovlev S. 2004. Genome size in Echinops L. and related genera (Asteraceae, Cardueae): karyological, ecological and phylogenetic implications. Biol Cell. 96 (2): 117–124.
- Geleta M, Bekele E, Dagne K, Bryngelsson T. 2010. Phylogenetics and taxonomic delimitation of the genus Guizotia (Asteraceae) based on sequences derived from various chloroplast DNA regions. Plant Syst Evol. 289 (1–2): 77–89.
- Gregory TR. 2001. Coincidence, coevolution, or causation? DNA content, cell size, and the C-value enigma. Biol Rev. 76 (1): 65–101.
- Gregory TR, editor, 2005. The evolution of the genome. Amsterdam: Elsevier
- Greilhuber J. 2005. Intraspecific variation in genome size in angiosperms: Identifying its existence. Ann Bot. 95 (1): 91–98.
- Greilhuber J, Doležel J, Lysák MA, Bennett MD. 2005. The origin, evolution and proposed stabilization of the terms 'genome size' and 'C-value' to describe nuclear DNA contents. Ann Bot. 95 (1): 255–260.
- Hawkins JS, Grover CE, Wendel JF. 2008. Repeated big bangs and the expanding universe: Directionality in plant genome size evolution. Plant Sci. 174 (6): 557–562.
- Heslop-Harrison JS, Schwarzacher T. 2011. Organization of the plant genome in chromosomes. Plant J. 66 (1): 18–33.
- The biology and chemistry of the Compositae, 2 vols Heywood VH Harborne JB Turner BL Academic Press London 1977
- Hidalgo O. 2006. El grupo Rhaponticum (Asteracae, Cardueae, Centaureinae): delimitación y filogenia [PhD thesis]. [Catalonia (Spain)]: University of Barcelona.
- Hidalgo O, Garcia-Jacas N, Garnatje T, Romashchenko K, Susanna A, Siljak-Yakovlev S. 2008. Extreme environmental conditions and phylogenetic inheritance: systematics of Myopordon and Oligochaeta (Asteraceae, Cardueae-Centaureineae). Taxon. 57 (3): 769–778.
- Hidalgo O, Garcia-Jacas N, Garnatje T, Susanna A. 2006. Phylogeny of Rhaponticum (Asteraceae, Cardueae-Centaureinae) and related genera inferred from nuclear and chloroplast DNA sequence data: taxonomic and biogeographic implications. Ann Bot. 97 (5): 705–714.
- Hidalgo O, Garcia-Jacas N, Garnatje T, Susanna A, Siljak-Yakovlev S. 2007. Karyological evolution in Rhaponticum Vaill. (Asteraceae, Cardueae) and related genera. Bot J Linn Soc. 153 (2): 193–201.
- Hidalgo O, Vallès J. 2012. First record of a natural hexaploid population for Valeriana officinalis: genome size is confirmed to be a suitable indicator of ploidy level in the species. Caryologia. 3 (3): 243–245.
- Hind DJN, Beentje HJ, D.J.N. Hind (Editor-in-Chief). 1996. Compositae: Systematics. In: Proceedings of the International Compositae Conference, Kew, 1994, vol. 1. Royal Botanic Gardens, Kew.
- Hind DJN, Jeffrey C, Pope GV, editors. 1995. Advances in Compositae systematics. Kew: Royal Botanic Gardens.
- Jones WE, Kuehnle AR, Arumuganathan K. 1998. Nuclear DNA content of 26 Orchid (Orchidaceae) genera with emphasis on Dendrobium. Ann Bot. 82 (2): 189–194.
- Kalendar R, Tanskanen J, Chang W, Antonius K, Sela H, Peleg O, Schulman AH. 2008. Cassandra retrotransposons carry independently transcribed 5S RNA. Proc Natl Acad Sci USA. 105 (15): 5833–5838.
- Kidwell MG. 2002. Transposable elements and the evolution of genome size in eukaryotes. Genetica. 115 (1): 49–63.
- Kimball RT, Crawford DJ. 2004. Phylogeny of Coreopsideae (Asteraceae) using ITS sequences suggests lability in reproductive characters. Mol Phylogenet Evol. 33 (1): 127–139.
- Knight CA, Molinari NA, Petrov DA. 2005. The large genome constraint hypothesis: evolution, ecology, and phenotype. Ann Bot. 95 (1): 177–190.
- Kubitzki K. 2007. The families and genera of vascular plants. In: Kadereit JW, Jeffrey C, editors. Flowering plants volume VIII. Eudicots. Asterales. Berlin, Heidelberg: Springer-Verlag Press. p. 639.
- Lamppa GK, Honda S, Bendich AJ. 1984. The relationship between ribosomal repeat length and genome size in Vicia. Chromosoma. 89 (1): 1–7.
- Lavia GI, Fernández A. 2008. Genome size in wild and cultivated peanut germplasm. Plant Syst Evol. 272 (1–4): 1–10.
- Lawrence ME. 1985. Senecio L. (Asteraceae) in Australia: nuclear DNA amounts. Aust J Bot. 33 (2): 221–232.
- Lee J, Baldwin BG, Gottlieb LD. 2003. Phylogenetic relationships among the primarily North American genera of Cichorieae (Compositae) based on analysis of 18S–26S nuclear rDNA ITS and ETS sequences. Syst Bot. 28 (3): 616–626.
- Leitch IJ, Beaulieu JM, Chase MW, Leitch AR, Fay MF. 2010. Genome size dynamics and evolution in monocots. J Bot. vol. 2010, article ID 862516, 18 pages, doi:10.1155/2010/862516. http://www.hindawi.com/journals/jb/2010/862516/
- Leitch IJ, Beaulieu JM, Cheung K, Hanson L, Lysak M, Fay MF. 2007. Punctuated genome size evolution in Liliaceae. J Evol Biol. 20 (6): 2296–2308.
- Leitch IJ, Bennett MD. 2004. Genome downsizing in polyploid plants. Biol J Linn Soc. 82 (4): 651–663.
- Leitch I, Chase MW, Bennett MD. 1998. Phylogenetic analysis of DNA C-values provides evidence for a small ancestral genome size in flowering plants. Ann Bot. 82(Suppl. A):85–94.
- Leitch IJ, Hanson L, Lim KY, Kovařík A, Chase MW, Clarkson JJ, Leitch AR. 2008. The ups and downs of genome size evolution in polyploid species of Nicotiana (Solanaceae). Ann Bot. 101 (6): 805–814.
- Leitch AR, Leitch IJ. 2008. Genomic plasticity and the diversity of polyploid plants. Science. 320 : 481–483.
- Lo Presti RM, Oppolzer S, Oberprieler C. 2010. A molecular phylogeny and a revised classification of the Mediterranean genus Anthemis s.l. (Compositae, Anthemideae) based on three molecular markers and micromorphological characters. Taxon. 59 (5): 1441–1456.
- MacArthur RH, Wilson EO. 1967. The theory of island biogeography. Princeton (NJ): Princeton University Press.
- Maddison WP, Maddison DR [Internet]. 2011. Mesquite: a modular system for evolutionary analysis. Version 2.75. Available from: http://mesquiteproject.org. 30 September 2011, CIPRES Project, USA.
- Maher EP, Fox DP. 1973. Multiplicity of ribosomal RNA genes in Vicia species with different nuclear DNA contents. Nature-New Biol. 245 (145): 170–172.
- Meng Y, Nie Z-L, Sun H, Yang Y-P. 2012. Chromosome numbers and polyploidy in Leontopodium (Asteraceae: Gnaphalieae) from the Qinghai-Tibet Plateau of S.W. China. Caryologia. 65 (2): 87–93.
- Murray BG. 2005. When does intraspecific C-value variation become taxonomically significant? Ann Bot. 95 (1): 119–125.
- Nagl W, Ehrendorfer F. 1974. DNA content, heterochromatin, mitotic index, and growth in perennial and annual Anthemideae (Asteraceae). Plant Syst Evol. 123 (1): 35–54.
- Oberprieler C, Himmelreich S, Vogt R. 2007. A new subtribal classification of the tribe Anthemideae (Compositae). Willdenowia. 37 : 89–114.
- Pellicer J, Garcia S, Canela MÁ, Garnatje T, Korobkov AA, Twibell JD, Vallès J. 2010. Genome size dynamics in Artemisia L. (Asteraceae): following the track of polyploidy. Plant Biol. 12 (5): 820–830.
- Pellicer J, Garcia S, Garnatje T, Vallès J. 2009. Changes in genome size in a fragmented distribution area: the case of Artemisia crithmifolia L. (Asteraceae, Anthemideae). Caryologia. 62 (2): 152–160.
- Pellicer J, Garnatje T, Molero J, Pustahija F, Siljak-Yakovlev S, Vallès J. 2010. Origin and evolution of the South American endemic Artemisia species (Asteraceae): Evidence from molecular phylogeny, ribosomal DNA and genome size data. Aust J Bot. 58 (7): 605–616.
- Pelser PB, Nordenstam B, Kadereit JW, Watson LE. 2007. An ITS phylogeny of tribe Senecioneae (Asteraceae) and a new delimitation of Senecio. Taxon. 56 (4): 1077–1104.
- Pires JC, Lim KY, Kovařík A, Matyásek R, Boyd A, Leitch AR, Leitch IJ, Bennett MD, Soltis PS, Soltis DE. 2004. Molecular cytogenetic analysis of recently evolved Tragopogon (Asteraceae) allopolyploids reveal a karyotype that is additive of the diploid progenitors. Am J Bot. 91 (7): 1022–1035.
- Price HJ, Chambers KL, Bachmann K. 1981. Genome size variation in diploid Microseris bigelovii (Asteraceae). Bot Gaz. 142 (1): 156–159.
- Prokopowich C, Gregory T, Crease T. 2003. The correlation between rDNA copy number and genome size in eukaryotes. Genome. 46 (1): 48–50.
- Rees H, Jones GH. 1967. Chromosome evolution in Lolium. Heredity. 22 (1): 1–18.
- Renzaglia KS, Rasch EM, Pike LM. 1995. Estimates of nuclear DNA content in bryophyte sperm cells: phylogenetic considerations. Am J Bot. 82 (1): 18–25.
- Rogers SO, Bendich AJ. 1987. Ribosomal RNA genes in plants: variability in copy number and in the intergenic spacer. Plant Mol Biol. 9 (5): 509–520.
- Romo A, Hidalgo O, Boratyński A, Sobierajska K, Jasińska AK, Vallès J, Garnatje T. 2013. Genome size and ploidy levels in highly fragmented habitats: the case of western Mediterranean Juniperus (Cupressaceae) with special emphasis on J. Juniperus L. Tree Gen Genomes. doi:10.1007/s11295-012-0581-9. 9(2):587–599
- Samuel R, Gutermann W, Stuessy TF, Ruas CF, Lack HW, Tremetsberger K, Talavera S, Hermanowski B, Ehrendorfer F. 2006. Molecular phylogenetics reveals Leontodon (Asteraceae, Lactuceae) to be diphyletic. Am J Bot. 93 (8): 1193–1205.
- Samuel R, Stuessy TF, Tremetsberger K, Baeza CM, Siljak-Yakovlev S. 2003. Phylogenetic relationships among species of Hypochaeris (Asteraceae, Cichorieae) based on ITS, plastid trnL intron, trnL-F spacer, and matK sequences. Am J Bot. 90 : 496–507.
- Sánchez-Jiménez I, Lazkov GA, Hidalgo O, Garnatje T. 2010. Molecular systematics of Echinops L. (Asteraceae, Cynareae): A phylogeny based on ITS and trnL-trnF sequences with emphasis on sectional delimitation. Taxon. 59 (3): 698–708.
- Sanz M, Vilatersana R, Hidalgo O, Garcia-Jacas N, Susanna A, Schneeweiss GM, Vallès J. 2008. Molecular phylogeny and evolution of floral characters of Artemisia and allies (Anthemideae, Asteraceae): Evidence from nrDNA ETS and ITS sequences. Taxon. 57 (1): 66–78.
- Semple JC, Watanabe K. 2009. A review of chromosome numbers in Asteraceae with hypotheses on chromosomal base number evolution. In: Funk VA, Susanna A, Stuessy TF, Bayer RJ, eds. Systematics, evolution and biogeography of Compositae. Vienna: International Association for Plant Taxonomy, 61–72.
- Simpson BB. 2009. Economic importance of Compositae. In: Funk VA, Susanna A, Stuessy TF, Bayer RJ, eds. Systematics, evolution and biogeography of Compositae. Vienna: International Association for Plant Taxonomy, 45–58.
- Siroky J, Lysák M, Doležel J, Kejnovský E, Vyskot B. 2001. Heterogeneity of rDNA distribution and genome size in Silene spp. Chromosome Res. 9 (5): 387–393.
- Soltis DE, Soltis PS, Bennett MD, Leitch IJ. 2003. Evolution of genome size in the angiosperms. Am J Bot. 90 (11): 1596–1603.
- Stuessy TF, Urtubey E, Gruendstaeudl M. 2009. Barnadesieae (Barnadesioideae). In: Funk VA, Susanna A, Stuessy TF, Bayer RJ, eds. Systematics, evolution and biogeography of Compositae. Vienna: International Association for Plant Taxonomy, 215–228.
- Suda J, Krahulcová A, Trávníček P, Rosenbaumová R, Peckert T, Krahulec F. 2007. Genome size variation and species relationships in Hieracium sub-genus Pilosella (Asteraceae) as inferred by flow cytometry. Ann Bot. 100 (6): 1323–1335.
- Suda J, Kyncl T, Freiová R. 2003. Nuclear DNA amount in Macaronesian angiosperms. Ann Bot. 92 (1): 153–164.
- Suda J, Kyncl T, Jarolímová V. 2005. Genome size variation in Macaronesian angiosperms: forty percent of the Canarian endemic flora completed. Plant Syst Evol. 252 (3–4): 215–238.
- Susanna A, Garcia-Jacas N, Hidalgo O, Vilatersana R, Garnatje T. 2006. The Cardueae (Compositae) revisited: insights from ITS, trnL-trnF and matK nuclear and chloroplast DNA analysis. Ann Mo Bot Gard. 93 (1): 150–171.
- Swift H. 1950. The constancy of deoxiribose nucleic acid in plant nuclei. Proc Natl Acad Sci USA. 36 (11): 643–654.
- Uhl CH. 1978. Chromosomes of Mexican Sedum, II. Section Pachysedum. Rhodora. 80 (824): 491–512.
- Torrell M, Vallès J. 2001. Genome size in 21 Artemisia L. species (Asteraceae, Anthemideae): Systematic, evolutionary, and ecological implications. Genome. 44 (2): 231–238.
- Vallès J, Garcia S, Hidalgo O, Martín J, Pellicer J, Sanz M, Garnatje T. 2011. Biology, genome evolution, biotechnological issues and research including applied perspectives in Artemisia (Asteraceae). In: Kader JC, Delseny M, eds. Advances in Botanical Research. Burlington, VT: Academic Press, 349–419.
- Vallès J, Pellicer J, Sánchez-Jiménez I, Hidalgo O, Vitales D, Garcia S, Martín J, Garnatje T. 2012. Polyploidy and other changes at chromosomal level and in genome size. Its role in systematics and evolution exemplified by some genera of Anthemideae and Cardueae (Asteraceae). Taxon. 61 (4): 841–851.
- Van’T Hof J. 1965. Relationships between mitotic cycle duration, S period duration and the average rate of DNA synthesis in the root meristem cells of several plants. Exp Cell Res. 39 (1): 48–58.
- Van’T Hof J, Sparrow AH. 1963. A relationship between DNA content, nuclear volume, and minimum mitotic cycle time. Proc Natl Acad Sci USA. 49 (6): 897–902.
- Vinogradov AE. 2001. Mirrored genome size distributions in monocot and dicot plants. Acta Biotheor. 49 (1): 43–51.
- Vinogradov AE. 2003. Selfish DNA is maladaptive: evidence from the plant red list. Trends Genet. 19 (11): 609–614.
- Vision TJ, Brown DG, Tanksley SD. 2000. The origins of genomic duplications in Arabidopsis. Science. 290 (5499): 2114–2117.
- Vitte C, Panaud O. 2005. LTR retrotransposons and flowering plant genome size: emergence of the increase/decrease model. Cytogenet Genome Res. 110 (1–4): 91–107.