Abstract
Male meiotic studies in eight Indian Cucumis species, including two newly included species, have been carried out. Gametic chromosome numbers of n = 7 and 12 were recorded in these taxa. Data collected on chromosome associations, chiasma frequency at diplotene/diakinesis/metaphase I revealed the predominant occurrence of bivalents in the majority of the pollen mother cells (PMCs) of taxa having n = 7, suggesting a high degree of homology among the pairing chromosomes. Conversely, species with n = 12 had chromosome associations resulting in a mixed of bivalents and univalents. One of the reasons for such heterogeneity exclusively found in these species may be attributed to chromosome structural alteration at DNA level (duplication/deletion/point mutation) rather than numerical alteration. Multivalents were not encountered in any of the PMCs, indicating the diploid nature of the taxa. A single nucleolus showing affinity towards varying numbers of bivalents (1–4) was the hallmark feature of the PMCs analyzed at diplotene.
Introduction
The genus Cucumis L., belonging to the family Cucurbitaceae, is one of the most economically important genera, and includes many vegetable crops such as cucumber, melon and West Indian gherkin. These cucurbitaceous vegetable crops are widely grown and are consumed in various forms, e.g. salad (cucumber, gherkins, long melon), pickles (gherkins), deserts (melons) and are also used for culinary purposes. Some of them are well known for their unique medicinal properties, e.g. seeds of C. callosus are being used as a herbal coolant in the hot Indian Thar desert (Kumar et al. 2006). It has been also reported that the alcoholic extract of C. trigonus is analgesic, anti-inflammatory, diuretic (Naik et al. Citation1980, Citation1981) and anabolic in nature (Mainkar et al. Citation1986). C. hystrix and C. trigonus are some of the neglected/underutilized cucurbits which are cultivated and consumed by tribal people of north-east India (Yadav et al. Citation2004). Despite having such utility as a horticultural crop, the total number of distinct and well-defined species, their origin and genomic circumscription as well as evolutionary pattern of the genus Cucumis is still debatable and an important point of discussion among the horticulturists worldwide (Schaefer Citation2007; Sebastian et al. Citation2010). Interestingly, the ambiguous number of species which ranged from 32 (Kirkbride Citation1993) to 52 (Schaefer Citation2007) reported so far, also results in a taxonomical dilemma (Ghebretinsae et al. Citation2007). In addition, recently Sebastian et al. (Citation2010) reported that Cucumis comprises some 25 Asian and Australian species in addition to its approximately 30 African species.
Among these species, cucumber (C. sativus) and muskmelon (C. melo) are extensively cultivated in the southeast Asian region, including India. The Indian subcontinent is considered to be the centre of origin for C. sativus and a centre of diversity for C. melo (Zeven and De Wet Citation1982). In the Indian subcontinent, the Himalayan Mountains are considered to be the centre of diversity of C. sativus (Křístková et al. Citation2003; Sebastian et al. Citation2010).
From a perusal of the literature it is clear that the genus Cucumis is very well characterized from morphological to genome level (Stepansky et al. Citation1999; Szabó et al. Citation2008; De wilde and Duyfjes 2007; Parvathaneni et al. Citation2011). Recently Huang et al. (Citation2009) have proposed the whole genome sequence of Cucumis sativus, which is one of the most important species and extensively cultivated for consumption. However, wild species and their cultigens did not receive due attention.
From a cytogenetic point of view, the data available so far are limited to few species, which might be due to the small size and poor stainability of chromosomes (Dane Citation1991). Perusal of the literature reveals that the somatic chromosome number of Indian representatives of the genus Cucumis ranges from 2n = 14 to 24, however the appropriate chromosome counts for many important species such as C. setosus, C. silentvalleyii, C. indicus, C. ritchiei, C. javanicus, C. leiospermus are yet to be ascertained. Male meiotic studies in the genus Cucumis is also restricted to few Indian species with few details on chromosome associations, recombinational frequencies, distributional pattern of bivalents/chromosome at anaphase I and II (Singh and Roy Citation1974, Citation1975; Dane and Tsuchiya Citation1979; Yadava et al. Citation1984; Qian et al. Citation2003; Cao et al. Citation2004). However, such information is totally lacking for C. setosus, C. callosus, C. silentvalleyii and C. maderaspatanus. Therefore, being the centre of origin and diversity, there is an urgent need to evaluate the cytogenetic mechanism underlying genome organization and speciation in the genus Cucumis and its Indian representative species. Hence, an attempt has been made to study chromosomal behavior during meiotic process in eight Indian species of Cucumis: C. sativus, C. sativus var. hardwickii, C. melo, C. callosus, C. trigonus, C. setosus, C. prophetarum, C. maderaspatanus and C. silentvalleyii.
Materials and methods
Flower buds of Cucumis species were collected from field grown plants at the National Bureau of Plant Genetic Resources (NBPGR), Thrissur and accession numbers have been obtained as well (Table ). Flower buds of appropriate size were collected from mature plants and fixed in freshly prepared Carnoy’s fluid for a minimum of 24 h at room temperature and later stored in 70% alcohol at 5–10°C for long-term storage. For meiotic studies, anthers were squashed in 2% acetocarmine. On average 25 pollen mother cells (PMCs) were analyzed at diplotene/diakinensis/metaphase I to estimate the range of chromosome associations and recombination frequencies through chiasma analysis. At anaphase I/II, 5–10 cells were analyzed for distributional pattern of chromosomes. The micro-photographs were taken from temporary preparations using a Jenoptik CCD camera (Jenoptik, Optical Systems GmbH, Jena, Germany) attached to a Labomed LX 400 trinocular research microscope (Labomed Inc., Mumbai, India).
Table 1. Mean number and range of associations, chiasmata at diplotene/diakinesis/metaphase I and terminalization coefficient in Cucumis species.
Results
The meiotic details of various accessions belonging to eight species of Cucumis, e.g. chromosome associations at diplotene/diakinensis/metaphase I, are summarized in Table . The data on total and mean number of chiasmata and its range along with number of chiasmata terminalized, and the terminalization coefficient are summarized in Table . The distribution patterns of chromosomes at anaphase I are summarized in Table and most of these observations are illustrated in Figures –. All the species presently investigated can be resolved into two distinct groups, i.e. I and II, on the basis of somatic chromosome number. Group I had 2n = 14 (C. sativus and C. sativus var. hardwickii), whereas group II revealed 2n = 24 (C. callosus, C. trigonus, C. setosus, C. melo, C. maderaspatanus, C. prophetarum and C. silentvalleyii).
Table 2. Anaphase I distribution in Cucumis species.
Figures 16–30. Male meiosis in Cucumis spp. (2n = 12). Diplotene: 16. C. maderaspatanus; 17. C. trigonus IC 550180; 18. C. melo IC 536705; 19. C. prophetarum W 54; 20. C. callosus IC 550172; 21. C. silentvalleyii JRN 01. Metaphase I: 22. C. callosus IC 550172; 23, 24. C. maderaspatanus; 25. C. silentvalleyii JRN 01; 26. C. melo IC 536705; 27. C. setosus. Anaphase I: 28–30. C. melo IC 536705.
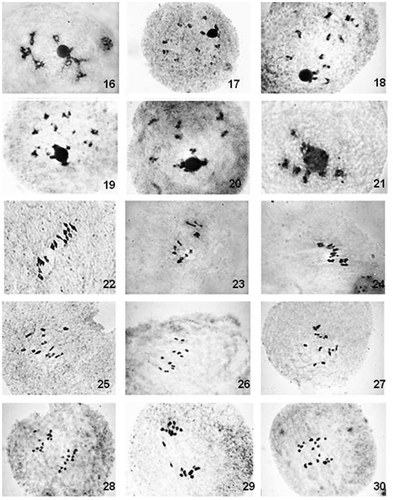
Figures 1–15. Male meiosis in Cucumis spp. (2n = 7). Diplotene/diakinensis: 1. C. sativus IC 550193; 2. C. sativus var. hardwickii IC 550197; 3. C. sativus IC 248283; 4. C. sativus SUC 6; 5. C. sativus var. hardwickii IC 256248; 6. C. sativus IC 469517. Metaphase I: 7. C. sativus SUC 6; 8. C. sativus var. hardwickii IC 550196; 9. C. sativus IC 550193; 10. C. sativus IC 550193. Anaphase I: 11. C. sativus var. hardwickii IC 550196; 12. C. sativus var. hardwickii IC 550197; 13. C. sativus var. hardwickii IC469591; 14. C. sativus IC 469517. Anaphase II: 15. C. sativus var. hardwickii IC469591.
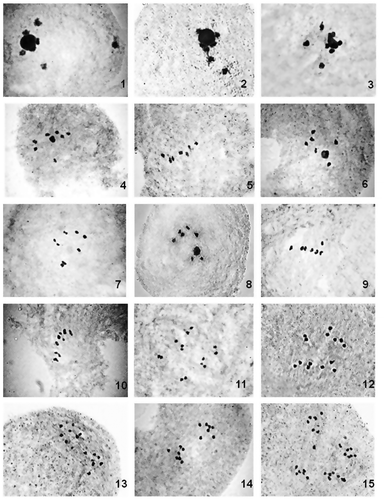
Chromosome associations
The percentage of presence of regular bivalents in both the groups I (2n = 14) and II (2n = 24) ranged from 75.92% to 94.87% and 3.70 to 100%, respectively. In group I, the mean value of total number of bivalents per PMC was observed to be highest (6.94) in C. sativus and lowest (6.57) in C. sativus var. hardwickii. On the other hand, it was highest (12.00) in C. maderaspatanus and lowest (10.00) in C. callosus.
In case of group I, among all the bivalents observed, the mean value of ring types was resolved as 4.45 and 3.94, whereas rod types were observed as 2.09 and 3.00 in C. sativus var. hardwickii and C. sativus, respectively. In group II, the mean value of ring type bivalent was observed as highest (9.42) and lowest (5.60) in C. setosus and C. melo, respectively. The mean value of rod type bivalents was resolved as highest (6.38) and lowest (2.53) in C. prophetarum and C. setosus, respectively.
The mean value of univalents ranged from 0.10 (C. sativus) to 0.96 (C. sativus var. hardwickii), where as in group II, it ranged from nil (C. maderaspatanus) to 4.00 (C. callosus).
Chiasma frequency and terminalization coefficient
In group I, the mean value of chiasmata per cell was found to be highest (11.14) and lowest (11.02) in C. sativus var. hardwickii and C. sativus respectively; of these 7.40 in C. sativus var. hardwickii and 7.74 in C. sativus were observed to be terminalized. In case of group II, chiasmata numbers were highest (21.53) in C. setosus and lowest (16.53) in C. melo. The terminalized chiasmata ranged from 9.63 (C. melo) to 12.72 (C. trigonus). The terminalization coefficient was observed as highest (0.85) in both C. prophetarum and C. silentvalleyii, and lowest (0.58) in C. melo.
Distribution pattern of chromosomes at anaphase I/II
The majority of the PMCs analyzed at anaphase I/II had shown equal distribution of chromosomes (7:7) and (12:12) in groups I and II, respectively.
Bivalents/univalents affinity towards nucleolus
A single, spherical, well-stained nucleolus was consistently observed in the majority of the PMCs of all the species analyzed, mostly at diplotene. In case of group I, 3–4 bivalents showed close association with nucleolus (Figures ). Conversely, the species of group II had only 1–3 bivalents with close affinity towards the nucleolus (Figures ). In case of C. trigonus, only 1 or 2 bivalents had association with the nucleolus (Figure ), whereas in the remaining species 2–3 bivalents were observed with the same tendency. Not a single univalent was observed in close affinity towards the nucleolus.
Discussion
According to Kirkbride (Citation1993), 32 species were recognized for the genus Cucumis, and later one more species was described by Thulin and Al-Gifri (Citation1994). Several workers (Ghebretinase et al. Citation2007; Schaefer Citation2007) opined that Cucumis in its current circumscription is paraphyletic, and to be monophyletic it must include five genera of the subtribe Cucumerinae, namely Cucumella, Dicoelospermum, Mukia, Myrmecosicyos and Oreosyce, which are morphologically related to various Cucumis species. The 19 species of the five genera are now formally transferred to the genus Cucumis, increasing the number of species from 33 to 52 (Ghebretinase et al. Citation2007; Schaefer Citation2007; Renner and Schaefer Citation2008). Recently Sebastian et al. (Citation2010) reported that the revised genus Cucumis now includes some 25 Asian and Australian species in addition to its approximately 30 African species.
In India the genus Cucumis is represented by six species, including five wild ones (Chakravarthy Citation1982), while a later treatment of the genus by Kirkbride (Citation1993) recognizes only four species, namely C. sativus, C. melo, C. prophetarum and C. hystrix; C. callosus and C. setosus are considered synonymous of C. melo and C. sativus, respectively. However, the exact number of Indian species still remains ambiguous and needs to be resolved. Among these, C. sativus and C. melo are the cultivated crop in India and it is presumed that the wild progenitor of C. sativus is C. sativus var. hardwiickii.
By combining the present data on male meiotic studies of eight Cucumis species with earlier published information (Dane and Tsuchiya Citation1979; Yadava et al. Citation1984; Qian et al. Citation2003; Cao et al. Citation2004) it is clear that the genus Cucumis is dibasic (x = 7 and 12). Two different hypotheses have been put forward to explain the relationship between these two unrelated numbers. The fragmentation hypothesis suggests that x = 12 has derived from x = 7 by fragmentation of particular chromosomes followed by de novo regeneration of centromeres (Bhaduri and Bose Citation1947; Ayyangar Citation1967). The fusion hypothesis, on the other hand, says that the basic number x = 7 might have arisen from x = 12, possibly by unequal translocation or fusion of non-homologous chromosomes (Trivedi and Roy Citation1970), which is the most reasonable hypothesis (Raamsdonk et al. Citation1989; Singh 1990). Huang et al. (Citation2009) established that five of the cucumber chromosomes arose from fusions of 10 ancestral chromosomes after divergence from C. melo.
Therefore, various taxa studied in the present investigations have been resolved into two groups, i.e. a group with n = 7 (C. sativus and C. sativus var. hardwickii) and a group with n = 12 (C. melo, C. callosus, C. setosus, C. trigonus, C. prophetarum, C. maderaspatanus and C. silentvalleyii). Occurrences of only bivalent or bivalent–univalent associations with no multivalent shows the diploid nature of all the species studied. In the species belonging to group I (n = 7), the mean value of bivalents ranges from 6.57 to 7, while the mean value of univalent range from 0 to 0.96. Among the bivalents, there are more ring bivalents than rod bivalents. The formation of higher numbers of bivalents; the occurrence of more ring than rod bivalents; and the lower numbers of univalents among the taxa belonging to the n = 7 group clearly indicate an extended homology in the chromosome complements, leading to relatively higher stability of the genome. These observations are in agreement with those of Yadava et al. (Citation1984) and are also supported by results from other plant species, e.g. Vicia (Kesavacharyulu Citation1988), Salvadora (Kumar et al. Citation2002), Cyamopsis (Purohit et al. Citation2011) and Senna (de Resende and Chamma Citation2013). On the other hand, different taxa belonging to group II (n = 12) were characterized in having comparatively fewer bivalents and more univalents. Our observations further drew support by the comparatively high number of rod bivalents in all the species belonging to group II. Such features are also indicative of possible structural heterogeneity in the genetic makeup of the bivalents. Structural heterozygosity in the bivalents as well as precocious separation of rod bivalents has been suggested as a reason for regular occurrence of univalents. Kumar and Singhal (Citation2013) has discussed the occurrence of structural heterozygosity, univalent chromosomes and their apparent effect on chiasma frequency and pollen fertility. Among the group II taxa, some wild species such as C. setosus, C. maderaspatanus, C. silentvalleyii, C. prophetarum and C. hystrix have fewer univalents, and an equal proportion of rod and ring bivalents, indicating the heterozygosity in the genetic makeup between the n = 7 and n = 12 groups Benhizia et al. (Citation2013). Further it may be recorded here that our observations on chromosome association of C. sativus and C. mello group are at variance with those of Yadava et al. (Citation1984) and Benhizia et al. (Citation2013).
Association of some bivalents (1–4) in both the groups might be indicative of the nucleolar nature of chromosomes in the complement. Our results are in agreement with Bhaduri and Bose (Citation1947) who also observed that four bivalents were attached with nucleoli during diakinesis. Chen et al. (Citation1999) reported that C. sativus possesses four pairs of rDNA loci and two pairs in C. melo and also hypothesized that the putative ribosomal loci are involved in nucleolus formation. The 45S rDNA was located on three pairs of C. sativus var. hardwickii chromosomes (Zhao et al. Citation2011). The identification of cucumber chromosomes was greatly improved by a combination of C-banding and FISH analysis with 45S and 5S rDNA probes, but the chromosomes could not be distinctly identified (Koo et al. Citation2002). The first report on molecular karyotyping of mitotic and meiotic spreads of cucumber was published by Koo et al. (Citation2005), dealing with C-banding, DAPI-stained pachytene analysis and RAPD-aided fluorescence in situ hybridization. Han et al. (Citation2008) analyzed repeat sequence composition in the genome of cucumber using whole genome shotgun reads and showed that satellite DNA sequences were the most dominant components. A karyotype showing the position and fluorescence intensity of several tandem repeat sequences was constructed and the establishment of such a FISH-based karyotype has led to the integration of molecular, genetic and cytogenetic maps in Cucumis sativus and culmination in genome sequencing project as well. By probing 45S rDNA and centromeric sequences to cucumber pachytene chromosomes, Koo et al. (Citation2010) demonstrated that cucumber chromosomes 1 and 2 might have evolved from fusions of ancestral karyotype with chromosome number n = 12. Sequential FISH with three sets of multi-probe cocktails (combined repetitive DNA with chromosome-specific fosmid clones as probes) provided the pattern of organization and distribution of highly repetitive DNA sequences among cultivar and wild cucumbers (Zhao et al. Citation2011).
The signals of 45S rDNA, which are usually associated with a secondary constriction, occupied the exclusive constriction region. Analyses of the distribution and organization of the nucleolar organizing region in rDNA-carrying chromosomes can allow better understanding of species evolution (Maluszynska and Heslop-Harrison Citation1993). Generally, secondary constrictions correlate well with the presence of active rRNA genes (Leitch and Heslop-Harrison Citation1992). The localization of rDNA loci will be useful in understanding the physical structure of the chromosome; in addition variation analysis at molecular level using various DNA marker systems (Sharma et al. Citation2010, Citation2011a, Citation2011b) may provide insight into the genome organization and its behavior, which needs to be investigated in more species of Cucumis.
The mean chiasma frequency has been observed to be optimal in most of the accessions studied here. Extreme small size of the bivalents has been the major obstacle in exact location and counting of chiasmata. However, most of the chiasmata are traced to distal position, leading to early terminalization of bivalents, even at the beginning of diakinesis itself, resulting in precocious separation of bivalents. Distally located chiasmata may have prevented some chromosome segments in the bivalents from undergoing genetic recombinations and enhancing linkage among the constituent genes (Chua and Roeder Citation1998; Moore Citation1998; Schnable et al. Citation1998; Sybenga Citation1996, Citation1999; Zickler and Kleckner Citation1999; Husband Citation2004). Such observations were earlier reported in large number of plants (Sybenga Citation1972, Singh Citation1993) including tropical hardwood tree species (Rao and Kumar Citation2004).
The equal distribution of chromosomes/chromatids at anaphase I is normal in the meiotic process in plants and is indicative of overall genomic stability and its manifestation. The few PMCs which showed lagging univalents/bivalents did not apparently influence the viability of the gametes.
Thus, our present observation of eight species of Cucumis indicates all the available Indian species did throw light on patterns of speciation vis-à-vis cytological events, which can be affectively utilized in future genetic improvement programs of important Indian wild cucurbits. From the forgoing discussion, it is clear that C. sativus and C. sativus var. hardwickii, both having n = 7, showed exclusive bivalent formation in most of the PMCs, thereby suggesting a high degree of homology among the pairing chromosomes. The high number of cells at anaphase I showing equal distribution of chromosomes also indicates the high degree of homology of bivalents of both these taxa, resulting in a balanced gametic formation. Conversely, as many as seven species with n = 12 had chromosome associations resulting in a mixed of bivalents and univalents in the majority of the PMCs, as recorded in C. callosus, i.e. 10 bivalents and four univalents per PMC on average. This indicates the possible heterogeneity in the genome, leading to few PMCs with unequal distribution in anaphase I, as observed for C. melo (Figures ). One of the reasons for the heterogeneity, exclusively found in species with n = 12, may be chromosome structural alteration at DNA level (duplication/point mutation) rather than numerical alterations.
The associations of the maximum number of four bivalents in C. sativus and C. sativus var. hardwickii are clear indications of the nuclear nature of these chromosomes. On the other hand, only three bivalents exhibit nucleolar nature in species with a gametic number of n = 12. Such interspecific differences are known to be common in cultivated plant species (Rao and Chandel Citation1991).
Acknowledgment
The present work is supported by a World Bank funded consortium research project of ICAR, New Delhi through the NAIP program. Sincere thanks are due to Prof. M.S. Bhist, Centre for Science Education, NEHU and Mr. Santosh Kumar Sharma and all other members of Plant Biotechnology Laboratories, Department of Botany as well as Department of Biotechnology & Bioinformatics, NEHU, Shillong for their constant encouragement and help.
References
- Ayyangar KR. 1967. Taxonomy of Cucurbitaceae. Bull Natl Inst Sci India. 34 : 380–396.
- Benhizia H, Benhizia Y, Ghernoub L, Siljak-Yakovlev S, Khalfallah N. 2013. Meiotic behaviour and karyotype features of endangered endemic fodder species Hedysarum perrauderianum (Fabaceae) in some populations from Algeria. Caryologia. doi:10.1080/00087114.2013.821838
- Bhaduri PN, Bose PC. 1947. Cytogenetical investigations in some cucurbits with special reference to fragmentation of chromosomes as a physical basis of speciation. J Genet. 48(2):237–256.
- Cao QH, Chen JF, Qian CT, Guo JY, 2004. Cytological studies on meiosis and male gametophyte development in cucumber. Acta Bot Boreal Occident. Sin. 24(9):1721–1726.
- Chakravarthy HL. 1982. Fascicles of flora of India – II Cucurbitaceae. Calcutta: Botanical Survey of India. p. 30–38.
- Chen JF, Staub JE, Adelberg JW, Jiang J. 1999. Physical mapping of 45S rRNA genes in Cucumis species by fluorescence in situ hybridization. Can J Bot. 77(3):389–393.
- Chua PR, Roeder GS. 1998. Zip2, a meiosis specific protein required for the initiation of chromosome synapsis. Cell. 93(3):349–359.
- Dane F. 1991. Cytogenetics in genus Cucumis. In: Tsuchiya T, Gupta PK, eds. Chromosome engineering in plants, part B: genetics, breeding, and evolution. Amsterdam: Elsevier, 201–204.
- Dane F, Tsuchiya T. 1979. Meiotic chromosome and pollen morphological studies of polyploid Cucumis species. Euphytica. 23(3):563–568.
- de Resende KFM, Chamma L. 2013. Chromosome number and meiosis in populations of Senna species (Caesalpinioideae – Fabaceae) from Southeast Brazil. Caryologia. 66(1):1–5.
- De wilde WJJO, BEE Duyfjes. 2007. The wild species of Cucumis L. (Cucurbitaceae) in South-East Asia. Adansonia. 29(2):239–248.
- Ghebretinsae AG, Thulin M, Barber JC. 2007. Relationships of cucumbers and melons unravelled: molecular phylogenetics of Cucumis and related genera (Benincaseae, Cucurbitaceae). Am J Bot. 94(7):1256–1266.
- Han YH, Zhang Z, Liu JH, Lu JY, Huang SW, Jin WW. 2008. Distribution of the tandem repeat sequences and karyotyping in cucumber (Cucumis sativus L.) by fluorescence in situ hybridization. Cytogenet. Genome Res. 12(1): 90–98.
- Huang S, Li R, Zhang Z, Li L, Gu X, Fan W, Lucas WJ, Wang X, Xie B, Ni P, Ren Y, Zhu H, Li J, Lin K, Jin W, Fei Z, Li G. 2009. The genome of the cucumber, Cucumis sativus L. Nat Genet. 41(12):1275–1281.
- Husband BC. 2004. Chromosomal variation in plant evolution. Am J Bot. 91(4):621–625.
- Kesavacharyulu K. 1988. Cytogenetics of Vicia [PhD thesis]. [Jodhpur (India)]: University of Jodhpur.
- Kirkbride Jh Jr. 1993. Biosystematic monograph of the genus Cucumis (Cucurbitaceae). Boone: Parkway Publications.
- Koo DH, Hur Y, Jin DC, Bang JW. 2002. Karyotype analysis of a Korean cucumber cultivar (Cucumis sativus L. cv. Winter Long) using C-banding and bicolor fluorescence in situ hybridization. Moll Cells 13(3):413–418.
- Koo DH, Choi HW, Cho J, Hur Y, Bang JW. 2005. A high resolution karyotype of cucumber (Cucumis sativus L. ‘Winter Long’) revealed by C-banding, pachytene analysis, and RAPD-aided fluorescence in situ hybridization. Genome. 48(3): 534–540.
- Koo DH, Nam YW, Choi D, Bang JW, de Jong H, Hur Y. 2010. Molecular cytogenetic mapping of Cucumis sativus and C. melo using highly repetitive DNA sequences. Chromosome Res. 18(3): 325–336.
- Křístková E, Lebeda A, Vinter V, Blahoušek O. 2003. Genetic resources of the genus Cucumis and their morphological description (English-Czech version). Hortic Sci. 30(1):14–42.
- Kumar A, Rao SR, Rathore TS. 2002. Cytological investigation in some important tree species of Rajasthan IV. Male meiosis studies in the genus Salvadora L. Cytologia. 67(2):105–115.
- Kumar P, Singhal VK. 2013. Reduction in chiasma frequency and pollen fertility due to multiple chromosomal associations and univalents in Saxifraga diversifolia from alpine regions of northwest Himalayas (India). Caryologia. 66(2):120–127.
- Leitch IJ, Heslop-Harrison JS. 1992. Physical mapping of the 18S-5.8S-26S rRNA genes in barley by in situ hybridization. Genome. 35(6):1013–1018.
- Mainkar AV, Naik VR, Dhume VG, Agshikar NV. 1986. Anabolic activity of alcoholic extract of Cucumis trigonus Roxburghii. Indian J Pharmacol. 18(4):261–262.
- Maluszynska J, Heslop-Harrison JS. 1993. Physical mapping of rDNA in Brassica species. Genome. 36(6):774–781.
- Moore G. 1998. To pair or not to pair: chromosome pairing and evolution. Curr Opin Plant Biol. 1:116–122.
- Naik VR, Agshikar NV, Abraham GJS. 1980. Analgesic and anti-inflammatory activity in alcoholic extracts, of Cucumis trigonous Roxb. – a preliminary communication. Pharmacology. 20(1):52–56.
- Naik VR, Agshikar NV, Abraham GJS. 1981. Cucumis trigonus Roxb. II. Diuretic activity. J Ethnopharmacol. 3(1):15–19.
- Parvathaneni RK, Natesan S, Devaraj AA, Muthuraja R, Venkatachalam R, Subramani AP, Laxmanan P. 2011. Fingerprinting in cucumber and melon (Cucumis spp.) genotypes using morphological and ISSR markers. J Crop Sci. Biotechnol. 14(1):39–43.
- Purohit J, Kumar A, Hynniewta M, Rao SR. 2011. Karymorphological studies in Guar (Cyamopsis tetragonoloba Linn. Taub) – an important gum yielding plant of Rajasthan. India. Cytologia. 76(2):163–169.
- Qian CT, Chen JF, Lou QF, Cao QH, Luo XD. 2003. Studies on meiotic behaviors of pollen mother cells in different ecotype cucumbers (Cucumis sativus L.). J Wuhan Bot Res. 21(3):193–197.
- Raamsdonk LWD, den Nijs APM, Jongerius MC. 1989. Meiotic analyses of Cucumis hybrids and an evolutionary evaluation of the genus Cucumis (Cucurbitaceae). Plant Syst Evol. 163(3): 133–146.
- Rao SR, Chandel KPS. 1991. Karyomorphological studies in the cultivated and wild Vigna species in Indian Gene Centre. Cytologia. 56(1):47–57.
- Rao SR, Kumar A. 2004. Chromosome studies in some important arid zone tree species of India. Indian J Biochem Cell Biol. 82(4):521.
- Renner SS, Schaefer H. 2008. Phylogenetics of Cucumis (Cucurbitaceae) as understood in 2008. In: Pitrat M, editor. Cucurbitaceae 2008, Proceedings of the IXth EUCARPIA meeting on genetics and breeding of Cucurbitaceae; 2008 May 21–24, Avignon (France): INRA.
- Thulin M, Al-Gifri AN. 1994. Cucumis canoxyi (Cucurbitaceae): a new species from Yemen. Nord J Bot. 14 (3): 315–317.
- Trivedi RN, Roy RP. 1970. Cytological studies in Cucumis and Citrullus. Cytologia. 35 (4): 561–569.
- Schaefer H. 2007. Cucumis (Cucurbitaceae) must include Cucumella, Dicoelospermum, Mukia, Myrmecosicyos, and Oreosyce: a recircumscription based on nuclear and plastid DNA data. Blumea. 52(1):165–177.
- Schnable PS, Hsia AP, Nikolav BJ. 1998. Genetic recombination in plants. Curr Opin Plant Biol. 1(2):123–129.
- Sebastian P, Schaefer H, Telford IRH, Renner SS. 2010. Cucumber (Cucumis sativus) and melon (C. melo) have numerous wild relatives in Asia and Australia, and the sister species of melon is from Australia. PNAS. 107(32):14269–14273.
- Sharma SK, Kumaria S, Tandon P, Rao SR. 2011a. Single primer amplification reaction (SPAR) reveals inter- and intra-specific natural genetic variation in five species of Cymbidium (Orchidaceae). Gene. 483(1-2):54–62.
- Sharma SK, Kumaria S, Tandon P, Rao SR. 2011b. Synaptic variation derived plausible cytogenetical basis of rarity and endangeredness of endemic Mantisia spathulata Schult. Nucleus. 54(2):85–93. doi:10.1007/s13237-011-0033-1
- Sharma SK, Rawat D, Kumar S, Kumar A, Kumaria S, Rao SR. 2010. Single primer amplification reaction (SPAR) reveals intra-specific natural variation in Prosopis cineraria (L.) Druce. Trees. 24(5):855–864.
- Singh AK, Roy RP. 1974. Karyological studies in Cucumis L. Caryologia. 27(2):153–160.
- Singh AK, Roy RP. 1975. Cytological studies in Cucumis hardwickii Royle. Indian J Bot Soc. 54(2):94.
- Singh RJ. 1993. Plant Cytogenetics. Boca Raton (FL): CRC Press.
- Stepansky A, Kovalski I, Perl-Treves R. 1999. Intraspecific classification of melons (Cucumis melo L.) in view of their phenotypic and molecular variation. Plant Syst Evol. 217(3–4):313–333.
- Sybenga J. 1972. General Cytogenetics. Amsterdam: North Holland Publishing Co.
- Sybenga J. 1996. Recombination and chiasmata: few but intriguing discrepancies. Genome. 39(3):473–484.
- Sybenga J. 1999. What makes homologous chromosomes find each other in meiosis. A review and hypothesis. Chromosoma. 108(4):209–219.
- Szabó Z, Gyulai G, Tóth Z, Heszky L. 2008. Morphological and molecular diversity of 47 melon (Cucumis melo) cultivars compared to an extinct landrace excavated from the 15th century. In: Pitrat M, editor. Cucurbitaceae 2008, Proceedings of the IXth EUCARPIA meeting on genetics and breeding of Cucurbitaceae, 2008 May 21–24, Avignon (France): INRA.
- Thulin M, Al-Gifri AN. 1994. Cucumis canoxyi (Cucurbitaceae): a new species from Yemen. Nord J Bot 14(3): 315 –317.
- Trivedi RN, Roy RP. 1970. Cytological studies in Cucumis and Citrullus. Cytologia. 35(4): 561–569.
- Yadav RK, Yadav DS, Sarma P. 2004. Diversity of Cucurbitaceous crops in north eastern region, Himalayan ecology. ENVIS Bulletin. 13(2):15-26.
- Yadava KS, Singh AK, Arya HC. 1984. Cytogenetic Investigation in Cucumis L. I. meiotic analysis in twenty four Cucumis species. Cytologia. 49(1):1–9.
- Zeven AC, De Wet JMJ. 1982. Dictionary of cultivated plants and their regions of diversity. Centre for Agricultural Publishing and Documentation: Wageningen. p. 263.
- Zhao X, Lu J, Zhang Z, Hu J, Huang S, Jin W. 2011. Comparison of the distribution of the repetitive DNA sequences in three variants of Cucumis sativus reveals their phylogenetic relationships. J Genet Genomics. 38(1):39–45.
- Zickler D, Kleckner N. 1999. Meiotic chromosomes: integrating structure and function. Ann Rev Genet. 33:603–754.