Abstract
The enzyme 3-hydroxy-3-methylglutaryl-CoA reductase (HMGR, EC 1.1.1.34) catalyzes the conversion of 3-hydroxy-3-methylglutaryl-CoA to mevalonate, which is the first committed step in the pathway for isoprenoid biosynthesis in plants. In this study we performed bioinformatics analyses on Rosaceae; 23 HMGR protein sequences in plants and three animal samples including human, mouse and fruit fly were aligned and analyzed by computational tools to predict the protein properties, such as molecular mass, pI, signal peptide, transmembrane and conserved domains, and secondary and spatial structures. Sequence comparison analysis revealed there is a high identity between plants and animals. The structural modeling showed that HMGR has a typical spatial structure with catalytic regions including L, N and S domains. The tertiary structure model of Malus domestica HMGR (accession number: ABQ52378) was further checked by the PROCHECK algorithm, which showed that 90.3% of the amino acid residues were located in the most favored regions in a Ramachandran plot, indicating that the simulated three-dimensional structure was reliable. Phylogenetic analysis showed that there is a close relationship among HMGR proteins in Rosaceae species; they are also similar to HMGRs of other organisms. According to these results, HMGRs are probably derived from a common ancestor.
Introduction
The enormous variety of metabolites in plants can be classified into two basic groups according to their functions: primary metabolites and secondary metabolites (Hartmann Citation1996). Primary metabolites include nucleic acids, proteins, fatty acids and others that participate in plant basic functions. Secondary metabolites influence ecological interactions between plants and their environment (Croteau et al. Citation2000). The structurally and functionally diverse isoprenoids (also called terpenoids) are among the largest and the most structurally varied groups of natural products, with over 30,000 known compounds, many of which have essential biological functions in plants, e.g. sterols as the essential components of bio-membranes, carotenoids and chlorophyllins as photosynthesis pigments, plant hormones as the regulators of plant growth, development and defense isoprenoids. Additionally, some isoprenoids are economically important chemicals, including flavors, pigments, waxes, rubbers, vitamins, taxol (Wani et al. Citation1971), artemisinin (Chen and Xu Citation1996) and ginkgolides (Cieza et al. Citation2003). There are two distinct pathways for isoprenoid biosynthesis in the plant kingdom: the well-studied mevalonate (MVA) pathway and the 2-C-methyl-D-erythritol 4-phosphate/1-deoxy-D-xylulose 5-phosphate pathway (MEP/DOXP pathway), which is mevalonate-independent. These two distinct pathways are localized in different subcellular compartments: the MVA pathway predominates in the cytosol and the MEP pathway in the plastid (Bick and Lange Citation2003). As shown in Figure (Denbow et al. Citation1996), 3-hydroxy-3-methylglutaryl-CoA reductase (HMGR, EC 1.1.1.34) catalyzes the conversion of 3-hydroxy-3-methylglutaryl-CoA (HMG-CoA) to mevalonate, which is the first step in the pathway for isoprenoid biosynthesis in plants.
Figure 1. Plant isoprenoid biosynthetic pathway, highlighting important end products. PP, pyrophosphate; PGR, plant growth regulator (data taken from Denbow et al. Citation1996).
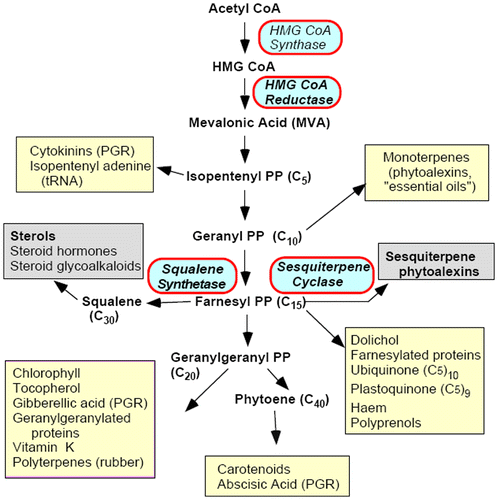
HMGR is a rate-limiting enzyme that can be inhibited specifically by lovastatin. It catalyzes the NADP-dependent synthesis of mevalonate from HMG-CoA, which is the most important step of the MVA biosynthetic pathway (Caelles et al. Citation1989). HMGR is well studied in archeobacteria, bacteria, fungi, plants and animals. In plants, overexpression of HMGR in the isoprenoid pathway has been attempted to increase triterpenoid productivity (Chappell et al. Citation1995; Schaller et al. Citation1995; Harker et al. Citation2003; Hey et al. Citation2006; Muñoz-Bertomeu et al. Citation2007). Despite improved productivities per unit weight, the transgenic plants sometimes showed growth inhibition, probably caused by metabolic imbalances. In fact, co-overexpression of the HMGR catalytic domain alleviated the growth inhibition caused by the individual overexpression of the HMGR catalytic domain (Manzano et al. Citation2004).
Structurally, HMGR consists of three regions: the N-terminal region that contains a variable number of transmembrane segments (usually seven in mammals, insects and fungi; two in plants), a linker, and a C-terminal catalytic region of approximately 400 amino acid residues. Although little sequence similarity is found among the transmembrane domains of HMG-CoA reductases from different species, the C-terminal catalytic region is highly conserved. The structure of this region is predicted to consist of amphipathic helices flanking an extended-pleated sheet (Istvan and Deisenhofer Citation2000).
The catalytic regions of plant HMGR consist of three domains: the small helical amino-terminal N-domain; the large, central L-domain harboring two HMG-CoA-binding motifs (EMPIGYVQIP and TTEGCLVA); and a NADP(H)-binding motif (GTVGGGT). Its architecture resembles a prism with an alpha helix forming the central structural element; the small helical S-domain harboring a NADP(H)-binding motif characterized by the sequence DAMGMNM (Ruiz-Albert et al. Citation2001). The nucleotides correlated to these motifs are well conserved and can be used for designing degenerate primers for isolating the members of hmgr gene family. In this work, we applied bioinformatics analysis to this key gene in Rosaceae for elucidation of structural, functional and phylogenetic relationships.
Materials and methods
Collection of HMGR sequences
All the HMGR protein sequences (FASTA format) belonging to the Rosaceae and three animal samples including human, mouse and fruit fly were downloaded from NCBI (http://www.ncbi.nlm.nih.gov; accessed December 2012) as listed in Table .
Table 1. The six species analyzed in this study.
Bioinformatics analyses
Several online web services and software packages were used for analyses of HMGRs in Rosaceae and other organisms. Comparative and bioinformatics analyses of HMGRs were carried out online at http://www.ncbi.nih.gov and http://expasy.org/tools. The phylogenetic analysis of HMGRs was performed with the DNASTAR package (MegAlign) using CLUSTAL-W with default parameters. The motifs of protein sequences were found using the Multiple Em for Motif Elicitation program (MEME; version 4.9) (Bailey et al. Citation2006) and Motif Alignment and Search Tool (MAST; version 4.9) (Bailey and Gribskov Citation1998) at http://meme.nbcr.net/meme. The parameters of MEME and MAST analyses were applied as follows: minimum width for each motif, six; maximum width for each motif, 20; maximum number of motifs to find, four; and number of repetitions, zero or one per sequence. A phylogenetic tree was constructed using MEGA 5 (Tamura et al. Citation2011) from aligned sequences. The maximum parsimony (MP) method was used to construct the phylogenetic tree as follows: MP search method, subtree-pruning-regrafting (SRR) on random trees; number of initial trees (random addition), 10; and MP search level, 1. The homology-based 3D structural modeling of Malus domestica HMGR (accession number: ABQ52378) was done by ESyPred3D Web Server 1.0 (automated homology modeling program using neural networks). WebLab ViewerLite 4 was used for 3D structure visualization. In order to assess the stereochemical qualities of 3D model, PROCHECK analysis (http://www.ebi.ac.uk/pdbsum/procheck) (Laskowski et al. Citation1993) was performed and a Ramachandran plot was drawn.
Results and discussion
In this study, 23 HMGR protein sequences belonging to Rosaceae and three samples of other organisms, including human (Homo sapiens), mouse (Mus musculus) and fruit fly (Drosophila melanogaster) were aligned by CLUSTAL-W. The results of the sequence alignment showed that HMGRs in Rosaceae and other organisms have a high homology with one another. HMGRs contained two HMG-CoA-binding motifs (ENVVGYVPIP and TTEGCLVA) and two NADP(H)-binding motifs (DAMGMNM and GTVGGGT) in Rosaceae.
The highest homology in HMGR protein sequences between human and Rosaceae belonged to the Malus domestica (AAQ54556) sequence with 60% identity; the lowest homology was observed between sequences of Homo sapiens (NP_001124468) and Fragaria ananassa (BAF79618), with 22.9% identity. As shown in Figure , motifs 2 (TTEGCLVA), 3 (DAMGMNM) and 4 (GTVGGGT) were more conserved than motif 1 (ENVVGYVPIP) in Rosaceae and three animal samples. However, two Rosaceae proteins, FaHMGR and MdHMGR, did not have one or more of the motifs, because of their short sequences. Figure shows some of the percentage identities among proteins in the multiple alignments of HMGR.
Figure 3. The percentage of sequence identity of residues across the Rosaceae and the three animal samples protein sequences that are shown in Figure .
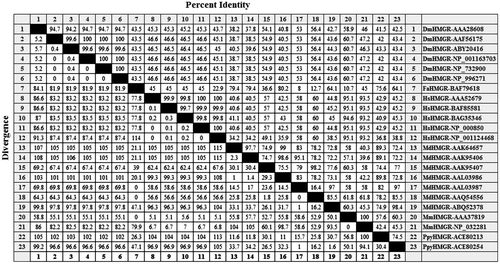
Figure 2. (Color online) Multiple alignment of amino acid sequences in Rosaceae and other HMGRs. Residues with yellow shading match the consensus; black shading indicates they differ from the consensus; and green shading indicates residues differ from the consensus by three distance units. The putative HMG-CoA-binding sites (1, 2, 3 and 4) were indicated in the shape.
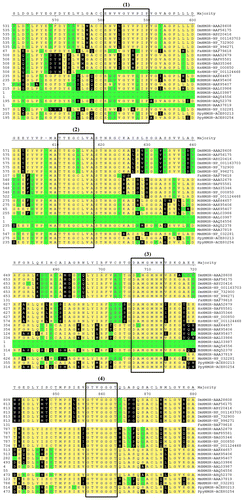
We have performed motif analyses of these proteins to find patterns of conserved motifs, and ran the MEME program to detect sequence motifs in Rosaceae and three animal samples (Figures , ).
Figure 5. (Color online) Motifs for HMGR proteins. The MAST motifs are shown as different-colored boxes.
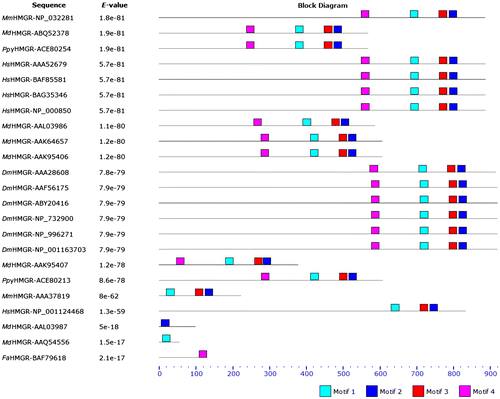
Figure 4. (Color online) Sequence-specific MEME motifs for HMGR proteins. Motif 1: width: 20, GN[YF]C[STC]DKKPAA[IV]NWI[EK]GRGK; motif 2: width: 20, DL[YH][IVM][ST][CV]TMPS[IL]E[VI]GTVGGGT; motif 3: width: 20, A[TC]GQD[PA]AQNV[EGT]SS[NH]C[IS]T[LAM]ME; motif 4: width: 20, [YF][YQS]VPMATTEG[CA]LVASTNRG[CF].
![Figure 4. (Color online) Sequence-specific MEME motifs for HMGR proteins. Motif 1: width: 20, GN[YF]C[STC]DKKPAA[IV]NWI[EK]GRGK; motif 2: width: 20, DL[YH][IVM][ST][CV]TMPS[IL]E[VI]GTVGGGT; motif 3: width: 20, A[TC]GQD[PA]AQNV[EGT]SS[NH]C[IS]T[LAM]ME; motif 4: width: 20, [YF][YQS]VPMATTEG[CA]LVASTNRG[CF].](/cms/asset/13434b6e-d399-453d-bfab-7b420b5077f8/tcar_a_856089_f0004_oc.gif)
To investigate the protein structures of HMGR in Rosaceae, Malus domestica HMGR (GenBank accession number: ABQ52378) was selected and analyzed by bioinformatics tools. The MdHMGR protein (length: 569 aa) had a molecular mass of 60.34 KDa and a theoretical pI (isoelectric point) value of 7.51 (http://www.expasy.org/tools/protparam) (Gasteiger et al. Citation2005). On the amino acid level, MdHMGR had high homology with many other plant HMGRs including those from Pyrus pyrifolia HMGR-ACE80254 (with 98.4% identity), Gossypium barbadense HMGR-ABC71313 (with 92.8% identity), Glycine max HMGR-AAD09278 (with 91.7% identity), and Bixa orellana HMGR-AAG43469 (with 90.4% identity). SignalP 4.1 analysis (http://www.cbs.dtu.dk/services/SignalP/) (Petersen et al. Citation2011) showed MdHMGR and other HMGRs in Rosaceae had no signal peptide that synthesized in the cytoplasm. MdHMGR contained two HMG-CoA-binding motifs (EMPVGYVQIP and TTEGCLVA) and two NADP(H)-binding motifs (DAMGMNM and GTVGGGT), which is similar to other HMGRs.
Analyzed by TMHMM 2.0 (http://www.cbs.dtu.dk/services/TMHMM-2.0/) (Moller et al. Citation2001), MdHMGR was found to contain two transmembrane domains (one located between A31 (P) and A53 (L) and the other located between A74 (L) and A96 (V) along the polypeptide chain) (Figures , ). The secondary structure of MdHMGR was analyzed by SOPMA (http://npsa-pbil.ibcp.fr/cgi-bin/npsa_automat.pl?page=npsa_sopma.html) (Geourjon and Deléage Citation1995) and the result showed that the putative MdHMGR peptide contains 43.94% of alpha helices, 15.82% of extended strands, 5.62% of beta turns and 34.62% of random coils. Penetrating through most parts of the secondary structure, alpha helices and random coils are the most abundant structural elements of MdHMGR and other plants of Rosaceae, while extended strands and beta turns were intermittently distributed in protein.
Figure 7. Prediction of transmembrane helices in Malus domestica HMGR (GenBank accession number: ABQ52378) by TMHMM server (CBS; Denmark).
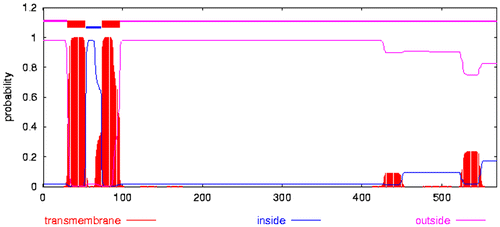
Figure 6. (Color online) Transmembrane and conserved domains in the protein sequence of Malus domestica HMGR (GenBank accession number: ABQ52378).
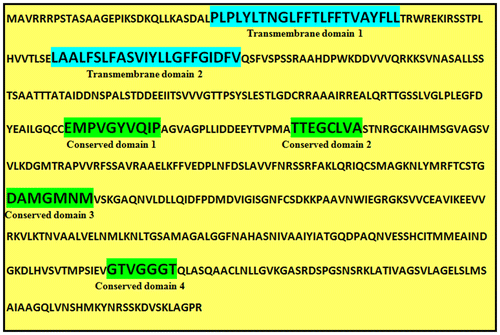
An important indicator of the stereochemical quality of the model is distribution of the main chain torsion angles phi and psi in the Ramachandran plot (Figure ). The Ramachandran plot shows the phi–psi torsion angles for all residues in the structure (except those at the chain termini). Glycine residues are separately identified by triangles. The coloring/shading on the plot represents the different regions described in Morris et al. (Citation1992): the darkest areas (here shown in red) correspond to the “core” regions representing the most favorable combinations of phi–psi values. The Ramachandran plot showed that all the main chain conformational angles fell into core and allowed regions. Therefore, the structure of MdHMGR has a good chain stereochemistry.
Figure 8. (Color online) Ramachandran plot of MdHMGR (Malus domestica HMGR; ABQ52378) (PDB accession code: 1DQ8). The regions are labeled as follows: A, core alpha; L, core left-handed alpha; a, allowed alpha; l, allowed left-handed alpha; ~a, generous alpha; ~l, generous left-handed alpha; B, core beta; p, allowed epsilon; b, allowed beta; ~p, generous epsilon; ~b, generous beta.
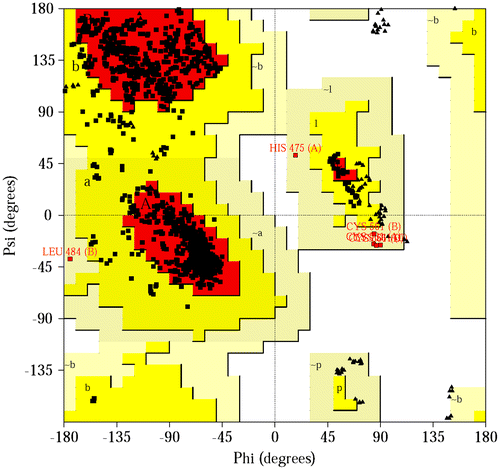
The 3D structure of MdHMGR was predicted by ESyPred3D Web Server 1.0 (http://www.unamur.be/sciences/biologie/urbm/bioinfo/esypred/) (Lambert et al. Citation2002). Molecular modeling results revealed that MdHMGR has an intricate spatial architecture which is very similar to human HMGR, whose catalytic portion and activity has been determined (Istvan and Deisenhofer Citation2000). The catalytic domain of MdHMGR consists of three domains: L-domain, N-domain and S-domain (Figure ).
Figure 9. (Color online) The 3D structure of MdHMGR (Malus domestica HMGR; ABQ52378), established by homology-based modeling (PDB accession code: 1DQ8). The α-helix is shown as helix-shaped, the sheet as wide ribbon-shaped and the random coil as line-shaped. The substrate-binding motifs are marked with letters. The 3D structure was shown by the solid ribbon model of WebLab ViewerLite 4.
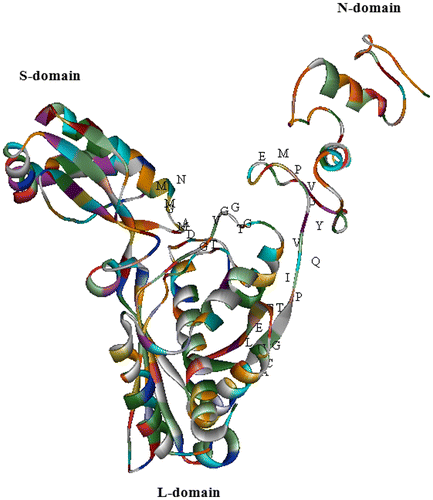
A phylogenetic tree was constructed based on the amino acid sequences of HMGRs in Rosaceae and other HMGRs from different organisms including human, mouse and fruit fly to investigate the evolutionary relationships among them. The result revealed that HMGRs were derived from an ancestor gene and evolved into different groups. According to the phylogenetic tree, HMGRs have a relationship with one another (Figure ).
Figure 10. (Color online) Phylogenetic tree of HMGRs from plants and animals using the CLUSTAL-W (MEGA 5) program. The maximum parsimony (MP) method was used to construct the tree. The percentage of 1000 bootstrap replicates is given at each node. Based on the phylogenetic tree result, 23 protein sequences of HMGR were defined, approximating four major groups (yellow: human; blue: mouse; red: fruit fly and green: plant).
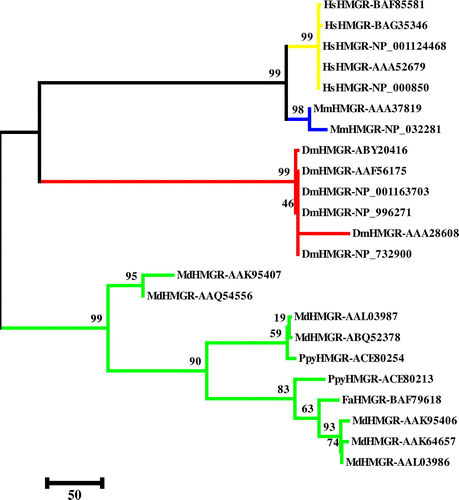
The biosynthesis pathways of isoprenoids in plants have been discovered and investigated. The genes encoding enzymes involved in both the MVA and MEP pathways have been identified. This has potential for bioengineering the isoprenoid biosynthetic pathways in plants. Various novel strategies are likely to further accelerate the progress of isoprenoid metabolic engineering (Mann et al. Citation2000; Romer et al. Citation2000; Schmidt-Dannert et al. Citation2000; Ye et al. Citation2000). Recently, bioinformatics and genomics tools have revolutionized the study of organisms’ metabolisms (Papini et al. Citation2010; Darabi et al. Citation2012; Umeton et al. Citation2012; Carapezza et al. Citation2013; Darabi and Seddigh Citation2013a, Citation2013b). Novel technologies include engineering isoprenoid-based plant defenses, improving the quality of crops, such as “Golden Rice” (Ye et al. Citation2000), breaking the isoprenoid biosynthetic bottleneck in peppermint to produce a higher amount of essential oils (Lange et al. Citation2001), rebuilding a pathway in bacteria to produce pharmaceuticals such as artemisinin (Martin et al. Citation2003), altering the metabolic flux to the desirable direction to yield high-content pharmaceutical agents such as terpenoid indole alkaloids (Fits and Memelink Citation2000), and blocking the checkpoint along the pathways to develop new herbicides (Lange and Croteau Citation1999) and anti-parasite drugs (Jomaa et al. Citation1999). Furthermore, isoprenoid biosynthesis is also important for plant growth and biomass properties (Eriksson et al. Citation2000). Although good progress has been made in the metabolic engineering of isoprenoids, it is still a largely undiscovered field (Soriente et al. Citation1999; Matthews and Wurtzel Citation2000).
The results of this study confirmed the findings of Istvan and Deisenhofer (Citation2000) about structures and transmembrane domains of HMGR, and showed that the HMGR gene family has a very conserved domain among plants and other organisms, therefore this family is a key biological elements during development. With the study of genes such as HMGR, it is expected that metabolic engineering of isoprenoid will be translated into industrial and pharmaceutical reality.
References
- Bailey TL, Gribskov M. 1998. Combining evidence using p-values: application to sequence homology searches. Bioinformatics. 14(1):48–54.
- Bailey TL, Williams N, Misleh C, Li WW. 2006. MEME: discovering and analyzing DNA and protein sequence motifs. Nucleic Acids Res. 34:369–373.
- Bick JA, Lange BM. 2003. Metabolic cross talk between cytosolic and plastidial pathways of isoprenoid biosynthesis: Unidirectional transport of intermediates across the chloroplast envelope membrane. Arch Biochem Biophys. 415:146–154.
- Caelles C, Ferrer A, Balcells L, Hegardt FG, Boronat A. 1989. Isolation and structural characterization of a cDNA encoding Arabidopsis thaliana 3-hydroxy-3-methylglutaryl coenzyme A reductase. Plant Mol Biol. 13:627–638.
- Carapezza G, Umeton R, Costanza J, Angione C, Stracquadanio G, Papini A, Lio P, Nicosia G. 2013. Efficient behavior of photosynthetic organelles via pareto optimality, identifiability and sensitivity analysis. ACS Synthetic Biology Publication. 2(5):274–288.
- Chappell J, Wolf F, Proulx J, Cuellar R, Saunders C. 1995. Is the reaction catalyzed by 3-hydroxy-3-methylglutaryl coenzyme A reductase a rate-limiting step for isoprenoid biosynthesis in plants? Plant Physiol. 109:1337–1343.
- Chen XY, Xu ZH. 1996. Recent progress in biotechnology and genetic engineering of medicinal plants in China. Med Chem Res. 6:215–224.
- Cieza A, Maier P, Poppel E. 2003. The effect of Ginkgo biloba on healthy elderly subjects. Fortschritte Der Medzin. 1:10–15.
- Croteau R, Kutchan TM, Lewis NG. 2000. Natural products (secondary metabolites). In Buchanan B, Gruissem W, Jones R, editors. Biochemistry and molecular biology of plants. Rockville, MD, USA: American Society of Plant Biologists. Chapter. 24:1255–1318.
- Darabi M, Masoudi-Nejad A, Nemat-Zadeh G. 2012. Bioinformatics study of the 3-hydroxy-3-methylglotaryl-coenzyme A reductase (HMGR) gene in Gramineae. Mol Biol Rep. 39:8925–8935.
- Darabi M, Seddigh S. 2013a. Conserved motifs identification of 3-hydroxy-3-methylglotaryl-coenzyme A reductase (HMGR) protein in some different species of drosophilidae by bioinformatics tools. Ann Biol Res. 4(4):158–163.
- Darabi M, Seddigh S. 2013b. Phylogenetic study of the 3-hydroxy-3-methylglotaryl-Coenzyme A reductase (HMGR) protein in six different family. Eur J Exp Biol. 3(4):158–164.
- Denbow CJ, Lang S, Cramer CL. 1996. The N-terminal domain of tomato 3-hydroxy-3-methylglutaryl-CoA reductases. Sequence, microsomal targeting, and glycosylation. J Biol Chem. 271:9710–9715.
- Eriksson ME, Israelsson M, Olsson O, Mortiz T. 2000. Increased gibberellins biosynthesis in transgenic trees promotes growth, biomass production and xylem fiber length. Nat Biotechnol. 18:784–788.
- Fits LVD, Memelink J. 2000. ORCA3, a jasmonate-responsive transcriptional regulator of plant primary and secondary metabolism. Science. 289:295–297.
- Gasteiger E, Hoogland C, Gattiker A, Duvaud S, Wilkins MR, Appel RD, Bairoch A. 2005. Protein identification and analysis tools on the ExPASy Server. In: Walker JM, editor. The proteomics protocols handbook. Totowa(NJ):Humana Press. p. 571–607.
- Geourjon C, Deléage G. 1995. SOPMA: significant improvement in protein secondary structure prediction by consensus prediction from multiple alignments. CABIOS. 11:681–684.
- Harker M, Holmberg N, Clayton JC, Gibbard CL, Wallace AD, Rawlins S, Hellyer SA, Lanot A, Safford R. 2003. Enhancement of seed phytosterol levels by expression of an N-terminal truncated Hevea brasiliensis (rubber tree) 3-hydroxy-3-methylglutaryl-CoA reductase. Plant Biotechnol J. 1:113–121.
- Hartmann T. 1996. Diversity and variability of plant secondary metabolism: a mechanistic view. Entomol Gen Application. 80:177–188.
- Hey SJ, Powers SJ, Beale MH, Hawkins ND, Ward JL, Halford NG. 2006. Enhanced seed phytosterol accumulation through expression of a modified HMG-CoA reductase. Plant Biotechnol J. 4:219–229.
- Istvan ES, Deisenhofer J. 2000. The structure of the catalytic portion of human HMG-CoA reductase. Biochem Biophys Acta. 1529:9–18.
- Jomaa H, Wiesner J, Sanderbrand S, Altincicek B, Weidemeyer K, Hintz M, Turbachova I, Eberl M, Zeidler J, Lichtenthaler HK, et al. 1999. Inhibitors of the nonmevalonate pathway of isoprenoid biosynthesis as antimaterial drugs. Science. 285:1573–1576.
- Lambert C, Leonard N, De Bolle X, Depiereux E. 2002. ESyPred3D: Prediction of proteins 3D structures. Bioinformatics. 18(9):1250–1256.
- Lange BM, Croteau R. 1999. Genetic engineering of essential oil production in mint. Curr Opin Plant Biol. 2:139–144.
- Lange BM, Ketchum REB, Croteau R. 2001. Isoprenoid biosynthesis: metabolite profiling of peppermint oil gland secretory cells and application to herbicide target analysis. Plant Physiol. 127:305–314.
- Laskowski RA, MacArthur MW, Moss DS, Thornton JM. 1993. PROCHECK: a program to check the stereochemical quality of protein structures. J Appl Cryst. 26:283–291.
- Mann V, Harker M, Pecker I, Hirschberg J. 2000. Metabolic engineering of astaxanthin production in tobacco flowers. Nat Biotechnol. 18:888–892.
- Manzano D, Fernández-Busquets X, Schaller H, González V, Boronat A, Arró M, Ferrer A. 2004. The metabolic imbalance underlying lesion formation in Arabidopsis thaliana overexpressing farnesyl diphosphate synthase (isoform 1S) leads to oxidative stress and is triggered by the developmental decline of endogenous HMGR activity. Planta. 219:982–989.
- Martin VJJ, Pitera DJ, Withers ST, Newman JD, Keasling JD. 2003. Engineering a mevalonate pathway in Escherichia coli for production of terpenoids. Nat Biotech. 21:796–802.
- Matthews PD, Wurtzel ET. 2000. Metabolic engineering of carotenoid accumulation in Escherichia coli by modulation of the isoprenoid precursor pool with expression of deoxyxylulose phosphate synthase. Appl Microbiol Biotechnol. 53:396–400.
- Moller S, Croning MDR, Apweiler R. 2001. Evaluation of methods for the prediction of membrane spanning regions. Bioinformatics. 17(7):646–653.
- Morris AL, McArthur MW, Hutchinson EG, Thornton JM. 1992. Stereochemical quality of protein structure coordinates. Proteins. 12:345–364.
- Muñoz-Bertomeu J, Sales E, Ros R, Arrillaga I, Segura J. 2007. Up-regulation of an N-terminal truncated 3-hydroxy-3-methylglutaryl CoA reductase enhances production of essential oils and sterols in transgenic Lavandula latifolia. Plant Biotechnol J. 5:746–758.
- Papini A, Stracquadanio G, Liò P, Nicosia G, Umeton R. 2010. Key enzymes for the optimization of CO2 uptake and nitrogen consumption in the C3 photosynthetic carbon metabolism. J Biotechnol. 150(suppl. 1):525–526.
- Petersen TN, Brunak S, Heijne GV, Nielsen H. 2011. SignalP 4.0: discriminating signal peptides from transmembrane regions. Nat Methods. 8:785–786.
- Romer S, Bramley PM, Fraser PD, Kiano JW, Shipton CA, Misawa N, Schuch W. 2000. Elevation of the provitamin A content of transgenic tomato plants. Nat Biotechnol. 18:666–669.
- Ruiz-Albert J, Cerda-Olmedo E, Corrochano LM. 2001. Genes for mevalonate biosynthesis in phycomyces. Mol Genet Genomics. 266:768–777.
- Schaller H, Grausem B, Benveniste P, Chye ML, Tan CT, Song YH, Chua NH. 1995. Expression of the Hevea brasiliensis (HBK) Müll Arg 3-hydroxy-3-methylglutaryl coenzyme A reductase 1 in tobacco results in sterol overproduction. Plant Physiol. 109:761–770.
- Schmidt-Dannert C, Umeno D, Arnold FH. 2000. Molecular breeding of carotenoid biosynthetic pathways. Nat Biotechnol. 18:750–753.
- Soriente A, De Rosa M, Scettri A, Sodano G, Terenico MC, Payá M, Alcaraz MJ. 1999. Manolide. Curr Med Chem. 6:415–431.
- Tamura K, Peterson D, Peterson N, Stecher G, Nei M, Kumar S. 2011. Mega5: Molecular evolutionary genetics analysis using maximum likelihood, evolutionary distance, and maximum parsimony methods. Mol Biol Evol. 28:2731–2739.
- Umeton R, Stracquadanio G, Papini A, Costanza J, Lio P, Nicosia G. 2012. Identification of Sensitive Enzymes in the Photosynthetic Carbon Metabolism. Adv Exp Med Biol. 736:441–459.
- Wani MC, Taylor HL, Wall ME, Coggon P, McPhail AT. 1971. Plant antitumor agents. VI. The isolation and structure of taxol, a novel antileukemic and antitumor agent from Taxus brevifolia. J Am Chem Soc. 93:2325–2327.
- Ye XD, Babili SA, Klöti A, Zhang J, Lucca P, Beyer P, Potrykus I. 2000. Engineering provitamin A (β-carotene) biosynthetic pathway into (caroteneoid free) rice endosperm. Science. 287:303–305.