Abstract
Hybrids were made between triticale (AABBRR) line T182 and a Thinopyrum amphiploid 8801(AABBEE) and progenies advanced up to F6. Multicolor genomic in situ hybridization (McGISH) revealed different ratios of R and E genome chromosomes in each of the lines examined. R and E genome chromosomes were eliminated by F5 in two of the lines studied. The ratios of R:E genome chromosomes varied from 2:8 to 12:2 in other F6 lines. Translocations between R and E genome chromosomes were detected as early as F3. A single wheat/R translocation was detected in one line in F6.
Introduction
Interspecific hybridization has, since the nineteenth century, been an important way to enrich the primary gene pool of wheat. Over the past 50 years, numerous crosses have been made between wheat and its relatives to transfer desirable genes into wheat. The Thinopyrum species, which carry important traits such as disease resistance (Martin et al. Citation1976; Wang and Liang Citation1977; Zhang et al. Citation2004) and stress tolerance (Foster et al. Citation1988; McDonald et al. Citation2001; Colmer et al. Citation2006), have been utilized frequently. Jauhar and Peterson (Citation1998, Citation2001) identified Fusarium head blight (FHB) resistance in hybrid derivatives from crosses between durum wheat cultivars and Th. elongatum, as well as an accession of Thinopyrum junceiforme. Colmer et al. (Citation2006) screened salinity tolerance of wheat relatives and found that the Thinopyrum species showed very high performance. In fact, many reports proved that wheat-elongatum amphiploids and chromosomes addition lines can enhance the salt tolerance of wheat (Dvořák and Ross Citation1986; Colmer et al. Citation1995). Resistance genes for Claviceps purpurea fungus were also present in Thinopyrum species (Cox et al. Citation2005). To date, at least 18 different genes have been introgressed into wheat from various Thinopyrum species (Fedak Citation2000; Ceoloni et al. Citation2000). In many cases, producing wheat/Thinopyrum amphiploids is only the first step in the process of transferring the desired genes from Thinopyrum to wheat (Xu et al. Citation1994; Zhang et al. Citation1996). Subsequently, additional manipulations need to be carried out between these amphiploids and wheat, barley, and triticale, in order to transfer the traits.
Triticale, the first synthetic hybrid cereal crop produced between the female parent wheat and the male parent rye, is being grown worldwide. Hexaploid triticale (2n = 42, AABBRR) has proven to be the most commercially successful to date, and most breeding and research continues with this type because of its superior vigor and reproductive stability compared to the octaploid type. However, it lacks the DD chromosomes from Triticum tauschii, which donated the baking quality genes to bread wheat. Moreover, it always requires substantial breeding work to remove the inherent problems which it expresses. These problems generally include partial floret sterility, shriveled seed, low yield potential, poor adaptation to local production conditions, and poor agronomic characteristics. Hence it has been treated as a minor crop in many countries. However, recent reports indicate that it has a potential to be an industrial crop, providing raw materials for biofuel, etc., for its production in marginal lands (Lam et al. Citation2001; Mergoum et al. Citation2009). Thus, it is necessary to broaden the genetic base of triticales by using multiple approaches. One approach is to synthesize new combinations of primary triticales using a diversity of wheat and rye parents. Another is to integrate the extensive variability of Thinopyrum into triticale.
Genomic in situ hybridization (GISH) is a powerful technique to identify alien chromatin in wheat–alien hybrids (Le et al. Citation1989; Fedak and Han Citation2005). The multicolor GISH technique enables three or more genomes of a polyploid species to be detected simultaneously, thus providing a more detailed genome analysis.
In this study, we report the introgression of E genome chromosomes into hexaploid triticale, utilizing multicolor GISH to monitor the process.
Materials and methods
The amphiploid 8801(AABBEE) with the E genome from Thinopyrum elongatum [Host] D. Dewey, derived from the hybrid Triticum turgidum (L.) (AABB)/Thinopyrum elongatum (EE) (Han, F. P, pc) is an excellent source of FHB resistance. We produced a hybrid between the amphiploid 8801 and the Canadian triticale × Triticasecale witt) line T182 (AABBRR) (Salmon, D. pc). All generations of progeny plants were grown in controlled-environment chambers at day/night temperatures of 20/15°C and a 16-h photoperiod. The F2 to f6 progenies were obtained by selfing of the previous generation.
Somatic chromosomes counts were determined on sections of root apical meristems that were pretreated in ice water at 4°°C for 24 h and fixed in 3:1 ethanol:acetic acid fixative solution. Chromosome staining was carried out by standard methods using 2% aceto carmine or Feulgen solution after hydrolysis in 1 N HCl for six minutes.
For studies of chromosome behavior at meiosis, young inflorescences were collected and fixed in Carnoy’s solution for 48 hours then transferred to 70% ethanol and stored in refrigerated conditions until used. Anthers were then squashed in 2% aceto carmine. Chromosome configurations were scored in a minimum of 20 cells per line.
For multicolor GISH, genomic DNAs of rye and Th. elongatum were extracted using the standard CTAB method (Kidwell and Osborn Citation1992), then purified with NaAc. Purified DNAs were labeled with biotinylated-dUTP and digoxigenin-dUTP respectively using nick translation kits (Roche, Germany). Total genomic DNA of Chinese Spring was used for blocking. Slide preparation, hybridization, washing conditions and probe detection were carried out as described by Han et al. (Citation2003) and rarely modified. Slides were examined with a Zeiss fluorescence microscope and photographs were taken on AxioCam HRm (Carl Zeiss, Canada).
Results
The somatic chromosome constitution of six progenies from hybrids between amphiploid 8801 and triticale strain T182 was monitored from the F2 to F6 generation using multicolor GISH. The results are shown in Table . Chromosome numbers in F2 progenies were only studied in three combinations; lines 1, 3 and 5. The chromosome numbers were 42 in all plants studied. Two of the progenies had 7 R genome and 7 E genome chromosomes, while the third had 8 R and 6 E genome chromosomes.
Table 1. McGISH analysis of segregating populations derived from triticale T182 (ABR) × partial amphiploid 8801(ABE).
The chromosome constitution of each line shown in Table was different as generations were advanced from F3 to F5 or F6. The progeny of plant 4b presented a relatively uncomplicated stream beginning with a total chromosome number of 41–43 in F4 with variable numbers of R and E genome chromosomes. Somewhat similar numbers of total chromosomes, R and E genome chromosomes were observed in F5. The final chromosome number in F6 was 42 including 12 R and two E genome chromosomes as shown in Figure .
Figure 1. An F6 mitotic cell of line 4b showing a total of 42 chromosomes consisting of 28 wheat plus 12 R genome chromosomes (green) and 2 E genome chromosomes (red).
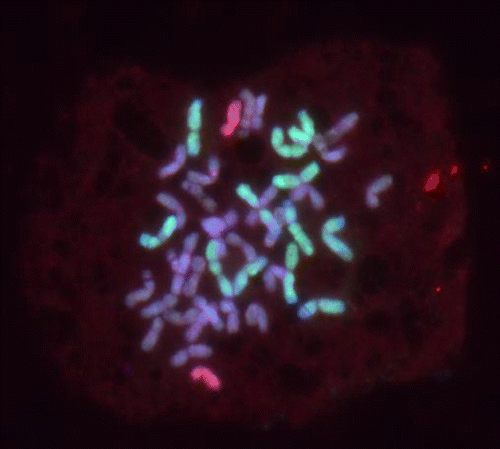
Progenies of plants in line 4a showed a more complex lineage. Beginning in F3, all categories of chromosomes showed wide ranges of numbers. Total chromosome numbers ranged from 42 to 46 while R and E chromosomes ranged from 4 to 11 and 5 to 9, respectively. E/R translocations were detected in F3; either one per cell (Figure a) or two per cell as shown in Figures b, c, and d.
Figure 2. E/R translocated chromosomes: (a) An F4 cell of line 5 with 28 chromosomes including one R (green) genome chromosome plus an E/R translocated chromosome (arrowed); (b) an F4 cell of line 4a showing four E genome chromosomes, 10 plus two telo R genome chromosomes plus two E/R translocated chromosomes (arrowed); (c) an F4 cell of line 4a showing eight E genome chromosomes, seven R genome chromosomes plus two E/R translocated chromosomes (arrowed); (d) an F4 cell of line 4a showing 10 E genome chromosomes, five plus telo R genome chromosomes plus two E/R translocated chromosomes (arrowed).
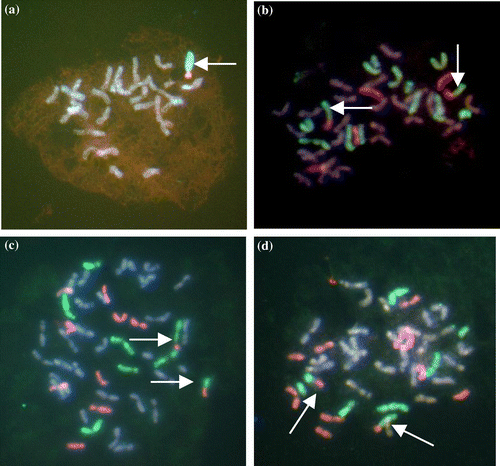
Wide ranges in chromosome numbers and constitutions were also detected in F4, with 30–45 total chromosomes and ranges of 1–10 R and 2–10 E genome chromosomes. The numbers were more stable in F5 with 42 chromosomes, including three R and 10 E chromosomes. The F6 chromosome constitution was a total of 36 comprised of two R plus eight E and two translocated chromosomes, an E/R and one wheat/R translocation (Figure ).
Figure 3. An F4 cell of line 4A showing eight E genome chromosomes, two from the R genome plus one E/R and one wheat/R translocated chromosomes (arrowed).
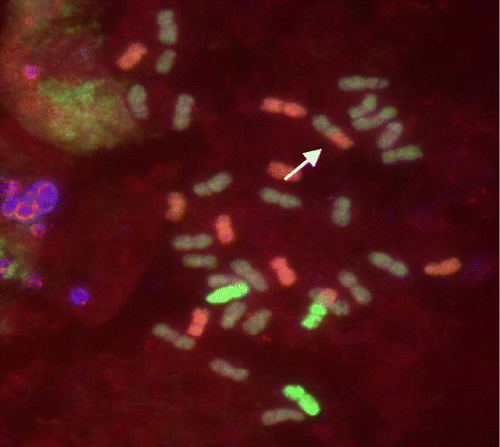
Line 3 exhibited 42 chromosomes in F2, consisting of 28 wheat, eight R and six E. The total chromosome number in F3 was 42 with a preponderance of R genome chromosomes (11) and only two E and two E/R translocations. The situation was similar in F4; however the F5 constitution was a total of 42 with 14 R genome chromosomes. Presumably the E genome and translocated chromosomes had been eliminated by this stage.
Line 1 showed a total chromosome number of 42 in F2 with seven E and seven R. Variable numbers of all three categories were observed in F3 and F4.
Lines 5 and 6 had highly variable numbers of E and R genome chromosomes in F3 and F4; however, by F5 only 28 chromosome plants were recovered.
Line 3, although it showed small numbers of E and translocated chromosomes in F4, returned to 28 wheat and 14 rye chromosomes in F5.
Lines 5 and 6 although they had variable numbers of E and R genome chromosomes in F3 and F4 both reverted to 28 chromosomes by F5.
Some common features were observed in progenies of all lines. E/R translocations were observed in all lines, beginning in F3; however by F5 and F6 only line 4a contained translocations. In most but not all lines in all generations, R genome chromosomes were more numerous than E genome chromosomes. Line 4a was an exception, where the proportion of E genome chromosomes was high in all generations, culminating with eight E genome chromosomes in F6.
Line 4a showed other unusual characteristics compared to the other lines. Most lines contained 42 chromosomes with 28 being of wheat origin. This was the case for line 4a in F5, however in F6 the chromosome constitution was somewhat unusual with a total chromosome number of 36, consisting of two R, eight E and two translocated chromosomes. That means that there were only 24 wheat chromosomes in that line.
Meiotic behavior was studied in F4 and F5 plants of line 4b containing a total of 42 chromosomes consisting of 28 wheat plus 12 R and two E chromosomes. Meiosis was very regular in these lines and similar in both generations. The total number of bivalents per cell was about 20 with an average univalent frequency of slightly in excess of one per cell (Table ).
Table 2. Meiotic characteristics of F4 and F5 progenies of line 4 derived from intercrosses of triticale T182 (ABR) × partial amphiploid 8801(ABE).
Discussion
The multicolor GISH technique was very efficient in determining the numbers of R and E genome chromosomes in various progenies at all generations. The ratio of R:E genome chromosomes through succeeding generations varied for all the lines. The ratio varied from 10:2 to 2:8 in various lines. In general, the number of alien chromosomes decreased with later generations in most populations. In some lines, such as 5 and 6, the alien chromosomes were eliminated entirely. Generally, the ratios of alien chromosomes varied with each line. From the patterns observed, it can be assumed that if sufficient numbers of lines were studied, it would be possible to identify lines with desired ratios of R and E genome chromosomes.
Intergenomic chromosome translocations have been previously reported in various types of intergeneric hybrids and partial amphiploids, etc. Intergeneric chromosome translocations were detected in progenies of wheat–Elymus repens hybrids (Zeng et al. Citation2013). Numerous intergenomic translocations were reported in partial amphiploids involving E1E2J and Js genomes (See Fedak and Han Citation2005, plus references contained therein). Spontaneous Robertsonian translocations (Ropiquet et al. Citation2008), are the most common in these types of amphiploids, but terminal, interstitial and trigenomic types have also been reported. Robertsonian translocations probably occur spontaneously between unpaired chromosomes, such as E and R in this study. There is not likely any affinity between these two genomes since no multivalents were observed in meiosis in this study (Table ). Therefore the E/R translocations must occur spontaneously as do the 1B/1R translocations in wheat rye studies. In this study they were detected as early as F3.
Alloploidy includes irreversible structural changes at the genome and chromosome levels (Leitch and Bennett Citation1997), DNA sequence levels (Song et al. Citation1995), gene expression (Scheid et al. Citation1996; Comai et al. Citation2000), or elimination of highly repetitive sequences (Salina et al. Citation2000). In this study, the only visible signs of structural changes in the aneuploids were the spontaneous translocations. These cytogenetic stocks might reveal additional alterations mentioned above, if the lines were so analyzed.
The parental amphiploid 8801 (ABE genome) possesses several useful agronomic traits that are lacking in triticale line T182. For example, 8801 has excellent resistance to Fusarium head blight (Miller et al. Citation2011), a trait that is lacking in most lines/cultivars of triticale. In addition, 8801 also has good resistance to Ug99 (the new race of stem rust-unpublished data).
Even after studying this small population of progenies there are numerous indicators of the potential of transferring E genome traits on E genome chromosomes into triticale. Another potential would be the establishment of novel amphiploids with combinations of both E and R genome traits.
Funding
China Scholarship Council 10.13039/501100004543
Disclosure statement
No potential conflict of interest was reported by the authors.
References
- Ceoloni C, Forte P, Ciaffi M, Nenno M, Bitti A, De Vita P, D’Egidio P. 2000. Chromosomally engineered durum wheat: the potential of alien gene introgressions affecting disease resistance and quality. Seminar on durum wheat improvement in the Mediterranean Region: new challenges; 2000 Apr 12–14; Zaragoza, Spain. Ciheam-options Mediterraneennes. p. 363–371.
- Colmer TD, Epstein E, Dvořák J. 1995. Differential solute regulation in leaf blades of various ages on salt-sensitive wheat and a salt-tolerant wheat X Lophopyrum elongatum (Host) A. Love amphiploid. Plant Physiol. 108(4):1715–1724.
- Colmer TD, Flowers TJ, Rana M. 2006. Use of wild relatives to improve salt tolerance in wheat. J Exp Bot. 57(3):1059–1078.
- Comai L, Tyagi AP, Winter K, Holmes-Davis R, Reynolds SH, Stevens Y, Byers B. 2000. Phenotypic instability and rapid gene silencing in newly formed Arabidopsis allotetraploids. Plant Cell. 12:1551–1567.
- Cox CM, Garett KA, Bockus WW. 2005. Meeting the challenge of disease management in perennial grain cropping systems. Renew Agric Food Syst. 20(1):15–24.
- Dvořák J, Ross K. 1986. Expression of tolerance of Na+, K+, Mg2+, Cl-, and SO42- ions and sea water in the amphiploid of Triticum aestivum × Elytrigia elongata. Crop Sci. 26(3):658–660.
- Fedak G. 2000. Wheat improvement using interspecific and intergeneric hybridization. In: Scientific principles of yield stability in plant production. An international symposium dedicated to the 90th anniversary of the founding of the V. Ya. Yurev Institute of Plant Production; Kharkiv, Ukraine.
- Fedak G, Han F. 2005. Characterization of derivatives from wheat-Thinopyrum wide crosses. (special issue: plant cytogenetics). Cytogenet Genome Res. 109:360–367.
- Foster BP, Miller TE, Law CN. 1988. Salt tolerance of two wheat/Agropyron junceum disomic addition lines. Genome. 30(4):550–564.
- Han FP, Fedak G, Benabdelmouna A, Armstrong K, Ouellet T. 2003. Characterization of six wheat × Thinopyrum intermedium derivatives by GISH, RFLP, and multicolor GISH. Genome. 46(3):490–495.
- Jauhar PP, Peterson TS. 1998. Wild relatives of wheat as sources of resistance to Fusarium head blight. Proceedings of the 1998 National Fusarium Head Blight Forum, 1998 Oct 26–27; Michigan University, East Lansing, MI. p. 77–79.
- Jauhar PP, Peterson TS. 2001. Hybrids between durum wheat and Thinopyrum junceiforme: prospects for breeding for scab resistance. Euphytica. 118(2):127–136.
- Kidwell KK, Osborn TC. 1992. Simple plant DNA isolation procedures. In: Beckman JS, Osborn TC, editors. Plant genomes: methods for genetic and physical mapping. Dordrecht: Kluwer Academic; p. 1–13.
- Lam HQ, Bigot LY, Delmas M, Avignon G. 2001. A new procedure for the destructuring of vegetable matter at atmospheric pressure by a catalyst/solvent system of formic acid/acetic acid. Applied to the pulping of triticale straw. Indust Crops Products. 14(1):139–144.
- Le HT, Armstrong KC, Miki B. 1989. Detection of rye DNA in wheat–rye hybrids and wheat translocation stocks using total genomic DNA as a probe. Plant Mol Biol Rep. 7(2):150–158.
- Leitch IJ, Bennett MD. 1997. Polyploidy in angiosperms. Trends Plant Sci. 2(12):470–476.
- Martin TJ, Harvey TL, Livers RW. 1976. Resistance to wheat streak mosaic virus and its vector, Acer tulipae. Phytopathology. 66(3):346–349.
- McDonald MP, Galwey NW, Ellneskog P, Coleme TD. 2001. Evaluation of Lophopyrum elongatum as a source of genetic diversity to increase the waterlogging tolerance of hexaploid wheat (Triticum aestivum). New Phytol. 151(2):369–380.
- Mergoum M, Singh PK, Pena RJ, Rio L, Cooper KV, Salmon DF, Macpherson HG. 2009. Triticale: a “new” crop with old challenges. Handbook of plant breeding ‘cereals’ edited by Carena, MJ. Springer New York Pub., pp 1–21.
- Miller SS, Watson EM, Lazebnik J, Gulden SM, Fedak G, Ouellet T. 2011. Characterization of an alien source of resistance to Fusarium head blight transferred to Chinese Spring wheat. Botany. 89(2):301–311.
- Ropiquet A, Gerbault-Seureau M, Deuve JL, Gilbert C, Pagacova E, Chai N, Rubes J, Hassanin A. 2008. Chromosome evolution in the subtribe Bovina (Mammalia, Bovidae): the karyotype of the Cambodian banteng (Bos javanicus birmanicus) suggests that Robertsonian translocations are related to interspecific hybridization. Chromosome Res. 16(4):1107–1118.
- Salina EA, Ozkan H, Feldman M, Shumny VK. 2000. Subtelomeric repeat reorganization in synthesized amphiploids of wheat. In: Salina EA, editor. Proceedings of the International Conference on Biodiversity and Dynamics of Systems, Institute of Cytology & Genetics. Novosibirsk, North Eurasia, Russia; p. 102–105.
- Scheid OM, Jakovleva L, Afsar K, Maluszynska J, Paszkowski J. 1996. A change in ploidy can modify epigenetic silencing. Proc Natl Acad Sci USA. 93(8):7114–7119.
- Song K, Lu P, Tang K, Osborn TC. 1995. Rapid genome change in synthetic polyploids of Brassica and its implications for polyploid evolution. Trends Ecol Evol. 9(3):348–352.
- Wang RR-C, Liang GH. 1977. Cytogenetic location of genes for resistance to wheat streak mosaic in an Agropyron substitution line. J. Hered. 68(2):375–378.
- Xu SJ, Banks PM, Dong YS, Zhou RH, Larkin PJ. 1994. Evaluation of Chinese Triticeae for resistance to barley yellow dwarf virus (BYDV). Gen Res Crop Evol. 41(1):35–41.
- Zeng J, Cao W, Hucl P, Yang Y, Xue AG, Chi DT, Fedak G. 2013. Molecular cytogenetic analysis of wheat – Elymus repens introgression lines with resistance to Fusarium head blight. Genome. 56(1):75–82.
- Zhang XY, Dong YS, Wang RR-C. 1996. Characterization of genomes and chromosomes in partial amphidiploids of the hybrid Triticum aestivum × Thinopyrum ponticum by in situ hybridization, isozyme analysis, and RAPD. Genome. 39(6):1062–1071.
- Zhang Z, Xu J, Xu Q, Larkin P, Xin Z. 2004. Development of novel PCR markers linked to the BYDV resistance gene Bdv2 useful in wheat for maker-assisted selection. Theor Appl Genet. 109(2):433–439.