Abstract
Investigations on the antimitotic and anticancer effect of Selol have been carried out for more than a decade. In a previous work we analysed ultrastructural changes in onion root tip meristem cells (the Allium test), their division activity (the mitotic index), and proportion of division phases (the phase index). Our study revealed inhibition of cell divisions in response to both compounds; however, the effect of sodium selenate (IV) on cells was more toxic, involving frequent clastogenic aberrations. The aim of the present work was to compare the effect of both compounds (organic and inorganic), containing selenium with oxidation number at +4, i.e. Selol and sodium selenate (IV), on ultrastructure of plant cells of the Allium test, and their concentration in plant cells. Analysis of ultrastructural changes showed high toxicity of sodium selenate (IV), with degradation of cytoplasm, nucleus and cell organelles. Treatment with Selol did not cause such dramatic changes in ultrastructure. It resulted in gradual increase of heterochromatinization of cell nuclei and a change in their shape, correlated with increasing concentrations of Selol and incubation period. Additionally, a comparison of concentration of both compounds in Allium test cells showed that the organic form of selenium (Selol) penetrated them much more slowly than the inorganic one (sodium selenate (IV)). The results obtained indicate much lower toxicity of Selol than sodium selenate (IV), which supports the necessity of further investigations of this compound as a potential safe anticancer drug.
1. Introduction
Selenium is a microelement having a very wide range of biological effects, both beneficial and toxic. It is an essential element for plant and animal organisms. In animals, selenium occurs in the form of selenoproteins, which participate in numerous biochemical processes; most importantly, protection against oxidative stress, and activation and inactivation of thyroid hormones (Stewart et al. Citation1999). Among selenoproteins, the family of glutathione peroxidases is the best known, protecting DNA, proteins, lipids and lipoproteins against damage (Wolf et al. Citation1997). In the human body, selenium is usually connected with amino acids, cysteine (selenocysteine) and methionine (selenomethionine), or incorporated in proteins (Gromadzińska et al. Citation2008; Zwolak and Zaporowska Citation2012). As an antioxidant, it takes part in metabolic processes at the cellular level, protecting cell membranes against reactive oxygen species.
The physiological role of selenium in plants is not yet fully understood. Its content in tissues depends on its levels in soil. There are plants capable of accumulating selenium (some species of Astragalus, Xylorrhiza, Oonopsis and Stanleya). In such plants, named selenium hyperaccumulators, the selenium content reaches 2–3% of dry mass (Brown and Shrift Citation1982; Wachowicz Citation1993). Selenium accumulators store it mainly in the form of selenocysteine, typical of animal cells, and selenomethionine, typical of plant cells. Selenium compounds are easily absorbed and accumulated by plants, although most species show low tolerance to that element, and its accumulation in tissues induces numerous metabolic disturbances (Terry et al. Citation2000). However, there is increasing evidence that low concentrations of selenium are advantageous for plants. It may enhance plants’ tolerance to oxidative stress induced by UV radiation (Hartikainen and Xue Citation1999), and delay their ageing (Xue et al. Citation2001). Their synergistic effect has been shown on transcription of antioxidative enzymes, such as superoxide dismutase (SOD) and glutathione peroxidase (GSH-PX) (Seppänen et al. Citation2003). These data may suggest that, in plants, selenium may act as an antioxidant or activate mechanisms alleviating effects of oxidative stress, preventing damage of lipids in cell membranes (Hawrylak and Szymańska Citation2007).
On the other hand, selenium is considered to be extremely toxic. The dose toxic to humans is 700 μg/24 h, while the daily request ranges from 50 to about 200 μg/24 h (Zagrodzki Citation2000). An abnormal concentration of selenium in an organism leads to inhibition of cell proliferation, DNA replication, protein synthesis, oxidative stress and intensified lipid peroxidation, and formation of complexes with metals accumulating in brain cells (Żbikowska Citation1997). The bioassimilability of selenium depends on its chemical form, food composition, and the individual traits of an organism. The easiest to assimilate are selenates with oxidation number at +4 and +6, selenomethionine, selenocysteine, and selenium amine compounds.
Due to the antioxidative properties of selenium and glutathione peroxidase and their protective role towards DNA, they have been long used in anticancer therapy (Floriańczyk Citation1999; Ganther Citation1999; El-Bayoumy and Sinha Citation2004; Abdulah et al. Citation2005). Selenium compounds are highly effective in therapy of breast cancer (Dorgan Citation1998), prostate cancer (Costello Citation2001; Clark and Marshall Citation2001), lung cancer (Van Zandwijk and Hirsch Citation2003), liver and colorectal cancer (Early et al. Citation2002) and astrocytoma (Zhu et al. Citation1995).
Sodium selenate is an inorganic compound containing selenium with oxidation number at +4, and is frequently used in anticancer therapy. It is highly effective; however, it displays high toxicity (LD50 3.5 mg kg−1 body mass), which limits its use in effective therapy, although it is still used in experimental studies (Gasmi et al. Citation1997; Jiang et al. Citation2001; Van Zandvijk and Hirsh Citation2003; Wierzbicka et al. Citation2007). An organic selenium compound, named Selol, also having oxidation number at +4, was synthesized at the Department of Drug Analysis, Medical University of Warsaw more than 10 years ago (Fitak et al. Citation1999). It is a mixture of selenitetriglycerides, obtained from sunflower oil, whose fatty acids contain most double bonds. It has been shown that it has antioxidative properties (“free radical scavenger”), and is an oxidant itself. It also shows comparatively low toxicity and thus may be safely used in therapy in doses reaching several mg kg−1 of the body mass (Zagrodzki et al. Citation2000). The preparation is increasingly widely used in experimental (Suchocki et al. Citation2007; Sochacka et al. Citation2014) and clinical studies (with volunteer participation). However, basic data on its effects on plant, animal and human cells are still unsatisfactory. Thus it is necessary to perform wider and more thorough studies on its cytological effects, cytotoxicity, and cytostatic effects, and to carry out a wider range of basic laboratory tests and preclinical and clinical studies that might lead to registration of Selol as an anticancer drug. In our previous work (Ślusarczyk et al. Citation2014), we compared the effect of Selol and sodium selenate (IV), with oxidation number at +4, on plant cells in the Allium test (Levan Citation1938). Based on analysis of structural changes in cells, their mitotic activity (mitotic index) and proportion of division phases (phase index), incubation in both selenium compounds led to changes in chromosome structure, consisting in their strong condensation and contraction (“cc chromosomes”), which was accompanied by gradual reduction or complete inhibition of cell division. Both compounds caused inhibition of cell division; however, sodium selenate (IV) was more toxic to cells, often leading to clastogenic aberrations, which were not observed in the case of Selol. Selol instead induced changes in structure of chromosomes, their condensation and contraction. This led to turbogenic effects, blocking further phases of cell division. The results obtained indicate much lower toxicity of Selol as compared with sodium selenate (IV), which confirms the need to further investigate it as a potential safe anticancer drug. This work, a continuation of our studies on mechanism of Selol action on plant cells, presents a comparison of Selol and sodium selenate (IV) effect on ultrastructure and their passage to meristematic cells of onion (Allium cepa L.) root tips, i.e. using the Allium test.
2. Materials and methods
2.1. Procedure of preparation of Selol 2%
Selol is a mixture of selenitetriglycerides containing selenium with oxidation number at +4. Synthesis of Selol is preceded by oxidation of a mixture of triglycerides (Suchocki et al. Citation2003) with saturated solution of KMnO4 in 0.5% H2SO4. The obtained emulsion is left for several days for breaking. Hydroxyl derivatives of triglycerides are subject to esterification with selenous acid (IV) (H2SeO3) in dioxane solution. A precise description of Selol 2% synthesis was published in a patent by Fitak et al. (Citation1999).
2.2. Procedure of preparation of sodium selenate (IV)
Sodium selenate (IV) (880 mg; Na2SeO3 from Sigma-Aldrich, Darmstadt, Germany) was dissolved in 100 ml of saline solution (0.9% NaCl), giving a solution of sodium selenate (IV) at concentration of 2 mg ml−1 Se, used for preparation of its micellar fraction.
2.3. Obtaining micelles containing 2% Selol and sodium selenate (IV)
Selol is a lipophilic compound, and its solubility in water posed some problems in our studies. The experiments presented herein required obtaining a form of Selol which would make a small-size fraction of micelles suspended in a water solution. The solution of sodium selenate (IV) was also prepared in the form of micelles. To 3 ml of Selol 2% and sodium selenate (IV), 0.5 g chicken egg lecithin was added; the ingredients were triturated in a porcelain mortar to a homogenous mixture, and, while constantly grinding, small amounts of saline solution were gradually added to obtain 50 ml of total volume of the suspension. After careful grinding, the solution of micelles was sonified at 80°C for 20 min (Fisher Sonic Dismembrator Model 150, sonifying head power 500 W; Fisher Scientific, Warsaw, Poland). Preparations of micelles obtained this way were kept at –20°C. Before starting the experiment, they were heated in a thermostat up to 22°C, and the required concentrations were prepared.
2.4. Allium test
Meristems of fibrous roots of onion (Allium cepa L.) were used, of genetically selected Dawidowska cultivar obtained from the Gospodarstwo Warzywno-Rolne (Vegetable and Agricultural Holding) in Babice Stare near Warsaw. The plants were grown in distilled water in a 200 ml Erlenmeyer flask until reaching the fibrous root length of about 3 cm (± 0.5 cm). They were used for testing (control) of penetration ability of Selol and sodium selenate (IV) meristematic cells, and studying their ultrastructure. The plants, together with roots, were incubated in 2% Selol in the form of micelle suspension, at concentrations of 25, 100, 400, 800 and 1600 μg ml−1 Se, and in a solution of sodium selenate (IV) in 0.9% NaCl, at concentrations of 25, 100, 200 and 400 μg ml−1 Se. Samples were taken after 6, 24 and 48 h. For each concentration, roots of two onions were used for incubation, several single roots taken for fixation after each period.
2.5. Study of Selol and sodium selenate (IV) concentrations in root tip meristem cells of onion (Allium test)
For the investigations, 2% Selol was used in the form of a micelle suspension, and sodium selenate (IV) (Na2SeO3) in 0.9% NaCl, containing 400 μg ml−1 Se and 800 μg ml−1 Se (IV). The roots were incubated in Selol and sodium selenate (IV) for 48 h. After 0, 6, 24 and 48 h they were rinsed with saline, cut off, frozen at –20°°C, and their Se (IV) content was determined using atomic spectrometry (ASA). To this end, portions of roots weighing 10–20 mg, ± 3 cm long, were transferred to Teflon crucibles, adding 3 ml 65% HNO3 to each, and mineralized in a Plazmatronika microwave mineralizer (Plazmatronika S.A., Wrocław, Poland). The mineralized samples were put in volumetric flasks and diluted 2500× with distilled and demineralized water. The last dilution was made with a 1% HNO3 solution, containing 5 mg ml−1 NiNO3. Measurements of selenium (IV) contents were made with a GBC Scientific Equipment (Hampshire, UK) Avanta Ultra Z single beam atomic absorption spectrometer with Zeeman-effect background correction (Suchocki et al. Citation2003).
2.6. Study of ultrastructure of Allium test cells in TEM
Onion root tips 2 mm in length, from all studied combinations, were cut off and fixed according to procedure developed by Kuraś (Citation1984), further used, among others, in our investigations, Ślusarczyk et al. (Citation2003). This process consists in 2-h fixation of root tips, incubation in solutions of Selol 2% or sodium selenate (IV), at the concentrations specified above, for 6, 24 and 48 h, in 2% glutaraldehyde at pH 7.2 (0.1 M cacodylate buffer). The material was then additionally fixed for 2 h in 2% OsO4 at 4°C, dehydrated in ethanol, and, through propylene oxide, embedded in the Epon/Spurr mixture of epoxy resins. Ultrathin sections (± 80 nm) were cut with RMC MTX Products (Boeckeler Instruments, Tucson, Arizona, USA) ultramicrotome. They were contrasted in saturated water solution of uranyl acetate (30 min) and lead citrate (30 min), according to Reynolds (Citation1963). The preparations were observed in JEM 1400 transmission electron microscope (JEOL Co., Tokyo, Japan).
3. Results
3.1. Analysis of selenium (IV) concentration in root tips of Allium cepa treated with Selol and sodium selenate (IV)
Penetration and accumulation of selenium coming from both compounds studied was varied and depended on the type of preparation, its concentration and incubation period. The quickest to penetrate cells was selenium in the form of sodium selenate (IV), in solutions of the lower concentration (400 μg ml−1 Se), reaching more than 10 times higher concentration that coming from solutions of 800 μg ml−1 Se. This is probably due to high toxicity of sodium selenate (IV), which damages cells more quickly at the concentration of 800 μg ml−1 Se (Table ). Penetration of selenium in the form of Selol into the roots depended also on the treatment period, but it was markedly slower. The amount of selenium measured in the roots took 48 h to reach the same level as that at 6 h with incubation in sodium selenate (IV) (Table ). This means that penetration of selenium in an organic form (Selol) into Allium test cells was significantly slower in comparison with its penetration in the inorganic form (sodium selenate (IV)).
Table 1. Contents of selenium (IV) in Allium cepa root tips treated with Selol and sodium selenate (IV).
3.2. Ultrastructure of control cells of onion root tip meristems (Allium test)
In the meristematic zone of onion roots, cells are highly differentiated; for this reason, analysis of ultrastructure was performed only using a small zone of actively dividing cells, located over the initial centre of the meristem. Those cells are not differentiated and constitute easy to identify, homogeneous material, suitable for comparative analyses of changes induced by different factors, including studies on solutions of Selol and sodium selenate (IV).
These cells show specific organization of ultrastructure, typical of undifferentiated and actively dividing cells (Figure a). They had thin cell walls of even thickness, with numerous plasmodesmata (Figure a). Their cytoplasm was dense, with low degree of vacuolation. In their central part, a large, oval nucleus was located, taking some 2/3 of the cell volume. It contained evenly distributed euchromatin, in a form of small darker spaces suspended in karyolymph (Figures a, b, d). One to three nucleoli were visible within a nucleus, with distinct granular and fibrillar components, containing vacuolated areas in the central part (Figures a, d). The cytoplasm contained evenly dispersed single ribosomes (Figures b, d) and scarce, small and electron-lucent vacuoles (Figure a, b, d). Mitochondria, having a comb-like structure, were usually oval, and distributed all over the cell cytoplasm (Figures a, d). They contained electron-grey matrix and a well-developed system of inner membranes (Figures b, c, d). Plastids were observed as oval structures with homogeneous and electron-dark matrix (Figures b, c). Rough (rER) endoplasmic reticulum was comparatively abundant, taking shape of cisternae and tubules of different length (Figures b, c, d). Active dictyosomes were often observed in its neighbourhood, surrounded by mitochondria (Figures b, c, d).
Figure 1. Ultrastructure of control Allium test cells. Bars = 2 μm. (a) Portion of cell with nucleus and typical organelles. (b) Portion of a cell with nucleus. Cytoplasm containing mitochondria, dictyosomes and rough ER. (c) Portion of cell cytoplasm with ER cisternae and mitochondria. (d) Portion of a cell with nucleus and typical organelles. G – Golgi apparatus, m – mitochondria, N – cell nucleus, n – nucleolus, p – plastids, r – ribosomes, rER – rough endoplasmic reticulum, v – vacuole.
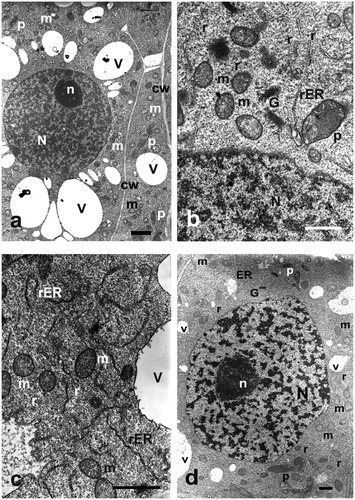
3.3. Ultrastructure of Allium test cells following treatment with Selol
Incubation in different concentrations of Selol resulted in changes in ultrastructure of Allium test cells. They were more intensive as the selenium concentration in the studied solutions and incubation time increased. Even the lowest concentration of Selol induced changes in cell nucleus shape and growth of cytoplasm vacuolation by formation of large number of small, merging vacuoles, and appearance of numerous areas of vacuolated cytoplasm.
Treatment with a higher concentration of Selol or increased incubation time caused more intensive condensation of nuclear chromatin and the appearance of a larger number of nuclei, having more irregular, lobe-like shapes (Figures a, b). Cytoplasm contained multiple small vacuoles with dark floccular aggregations inside (Figures b). Mitochondria were comparatively abundant within cells. They were usually ball-shaped and a little smaller than in the control cells. Their matrix was generally dark and dense, with numerous cristae (Figure c). Treatment with the highest concentration of Selol caused strong condensation of chromatin, and nuclei were often very irregular in shape (Figures d). Numerous, swollen ER cisternae and active Golgi structures were observed (Figure c). Vacuoles were very large and they still contained floccular deposits (Figure d). Treatment with Selol did not cause any observable damage to outer or inner membranes of organelles (Figures c, d).
Figure 2. Ultrastructure of Allium test cells following incubation in Selol solutions of different concentration and incubation periods. Bars = 2 μm. (a) Cell following incubation in Selol, at concentration of 100 μg ml−1 Se after 24 hours of incubation. Apparent increased vacuolation and cell nucleus of typical changed shape, with chromatin condensed (arrow). (b) Portion of a cell following incubation in Selol solution of 800 μg ml−1 Se for 48 hours. Nucleus of typical changed shape with condensed chromatin (arrow). (c) Border between two cells following incubation in Selol solution of 800 μg ml−1 Se for 48 hours. (d) Portion of a cell following incubation in Selol solution of 1600 μg ml−1 Se for 24 hours, revealing deformed nucleus, vacuoles with floccular deposits and cell wall traversed by plasmodesmata. cw – cell wall, d – deposits, G – Golgi apparatus, m – mitochondria, N – cell nucleus, n – nucleolus, p – plastids, rER – rough endoplasmic reticulum, v – vacuole.
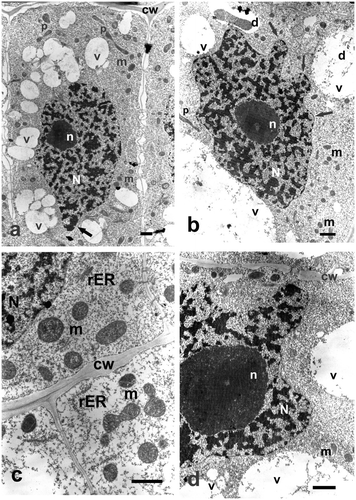
3.4. Ultrastructure of Allium test cells following treatment with sodium selenate (IV)
For all the studied concentrations and incubation periods, changes in cell ultrastructure following treatment with sodium selenate (IV) were very dramatic and clearly indicated its toxic effect. As early as after 6 h of incubation in the lowest concentration (25 μg ml−1 Se) of sodium selenate (IV), nuclei were deformed and their chromatin condensed (Figure a). Cytoplasm contained aggregations of mitochondria and plastids, most of them with electron-dense matrix, and large vacuoles. As the incubation period or concentration of sodium selenate (IV) solution increased, degradation of cells was more and more conspicuous. Incubation in higher concentrations of sodium selenate (IV) caused shrinking of cell nuclei and their nucleoli, and still stronger condensation of chromatin. Cytoplasm and organelles within it were shrunken and degraded; quite frequently only their remainders were seen (Figures b, c).
Figure 3. Ultrastructure of Allium test cells following incubation in sodium selenate (IV) solutions of different concentrations and incubation periods. Bars = 2 μm. (a) Cells containing deformed nuclei with strongly condensed chromatin, following incubation in sodium selenate (IV) solution of 25 μg ml−1 Se for 24 hours. (b) Cells containing deformed nuclei with strongly condensed chromatin following incubation in sodium selenate (IV) solution of 200 μg ml−1 Se for 24 hours. Apparent increase of heterochromatinization and progressing degradation of cell cytoplasm. (c) Portion of a cell following incubation in sodium selenate (IV) solution of 400 μg ml−1 Se for 48 hours. Cell nucleus of a typical changed shape, with strongly condensed chromatin. (d) Cell undergoing degradation following incubation in sodium selenate (IV) solution of 400 μg ml−1 Se for 48 hours. Cell nucleus displaying a typical changed shape, with strongly condensed chromatin, protruding outwards (arrows). cw – cell wall, lb – lipid body, m – mitochondria, N – cell nucleus, n – nucleolus, v – vacuole.
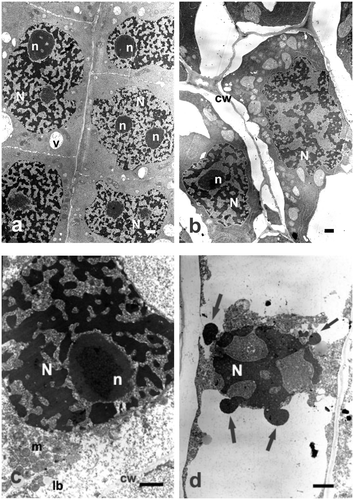
At the highest concentration used (400 μg ml−1 Se), chromatin was extremely condensed, mitochondria and plastids swollen, with lucent matrix and damaged membranes. Cytoplasm contained very long, concentric ER cisternae, and numerous vacuoles. It was detached from the cell wall in many places. Finally, total degradation of cells took place. The entire cell was filled with a large vacuole with the remains of cytoplasm and cell organelles (Figure a), containing numerous lipid bodies, or sometimes multivesicular bodies (Figures b, c). Cell nuclei were shrunken, more or less ball-shaped, and the nuclear envelope broken. Chromatin leaked out of them in a form of strongly condensed vesicles (Figures d, a, b, c, d). Long incubation in the highest sodium selenate concentration thus caused total degradation of cytoplasm and organelles within it (Figures a). Only an extremely shrunken nucleus remained, without nucleoli, its perforated chromatin forming specific chromatin protrusions (Figures d, b, c, d).
Figure 4. Ultrastructure of Allium test cells following incubation in sodium selenate (IV) solutions of 400 μg ml−1 Se after 48 hours of incubation. Bars = 5 μm. (a) Allium test cells in the process of degradation. (b) Highly degraded cell nucleus with chromatin vesicles leaking out. (c) A cell following 48 hours of incubation in sodium selenate (IV) solution. Degraded cytoplasm and organelles, cell nucleus displaying chromatin leaking out (arrows). (d) Strongly degraded cell nucleus with chromatin leaking out (arrows). cw – cell wall, lb – lipid body, mv – multivesicular body, N – cell nucleus.
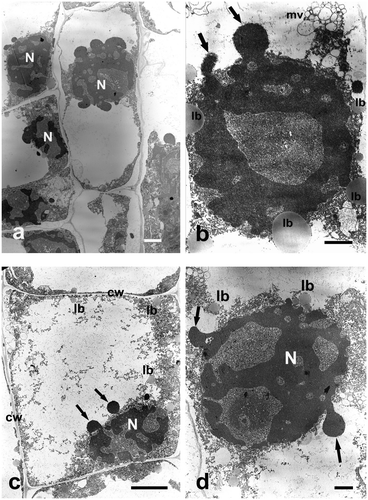
4. Discussion
Selenium is a microelement essential for proper functioning of animal and human organisms. In 1970s, the earliest reports on the relation between selenium and the risk of neoplastic diseases appeared. Introduction of selenium preparations to prophylaxis and therapy has potential as an alternative medicine strategy.
In our work, we compared the effects of organic (Selol) and inorganic (sodium selenate (IV)) selenium compounds, using the Allium plant test. The test was developed by Levan (Citation1938) and uses root tip meristem cells of onion, Allium cepa. Its main advantage is the simplicity of obtaining a large amount of genetically homogeneous material at a single stage of ontogenesis, and its use in karyological studies: the small number (16) of large chromosomes means that it is useful,for analysis of chromosome morphology, course of divisions, and or mutagenic aberrations and changes. These elements are crucial for etiology and therapy of cancerous diseases. This test is often used in screening tests and analysis of potential anticancer drugs, with respect to their cytostatics and cytotoxicity, as well as pathogens and environmental pollution (Gabara et al. Citation2006; Kuraś et al. Citation2006). Our studies compared the effect of treatment with both forms of selenium on Allium test cell ultrastructure, and their ability to penetrate inside the onion roots in the Allium test.
The measurements of Se concentration in root tips of Allium cepa L., treated with Selol and sodium selenate (IV), showed that Selol 2% in micelles penetrates the roots, where it accumulates. The intensity of the process depends on concentration of Selol and incubation time. However, sodium selenate (IV) penetrates much more quickly and accumulates in the roots in higher amounts. Sodium selenate (IV) concentration of 800 μg ml−1 Se impaired the cells and, in particular, it damaged membranes of cell structures. For this reason, higher accumulation of selenium was observed during incubation at the concentration of 400 μg ml−1 Se. Remarkably, 25 times more selenium was accumulated in roots after 48 hours of incubation in sodium selenate (IV) solution at concentration of 400 μg ml−1 Se than after treatment with Selol at the same concentration of selenium. This may mean that it is easier for cells to absorb selenium as sodium selenate, which is confirmed by literature data. Sodium selenate (IV) is one of the easiest selenium compounds for plants to absorb. It is known to penetrate inside the cells and move inside the plant organism by symplastic transportation (Whanger Citation2002; Wierzbicka et al. Citation2007). Inside the cell, it is further transformed,to Se-methyl-selenocysteine, among other compounds (Wierzbicka et al. Citation2007). The synthesized selenoamino acid is a non-protein amino acid. However, it is known that selenocysteine and selenomethionine are also synthesized in plant cells. Those two amino acids are non-specifically incorporated into newly synthesized proteins, replacing cysteine and methionine, often deactivating the protein functions (Whanger Citation2002). This is the main mechanism of the toxic effect of selenium. The results obtained herein confirm the toxic effect of selenium IV on the Allium test cells. Even the lowest concentrations of this compound used for incubation caused significant changes in ultrastructural cell organization. One of its effects was deformation of interphase cell nuclei shape to an irregular shape. Cell nuclei maintained their post-telophase shape and contained strongly spiralled chromatin. Changes of nucleus shape are often observed after exposure of a plant cell to different toxic factors, such as heavy metal ions or toxic compounds (Lemasters et al. Citation1997; Majewska et al. Citation2002). It is likely that the irregular shape of nuclei is caused by pressure of numerous vesicles and vacuoles that form as a result of action of those substances in the cell, which was also observed by us. Such changes may also result from disturbances in function of the cytoskeleton, which is responsible for maintaining the cell’s shape. Prolonged incubation and higher concentration of sodium selenate (IV) led to strong condensation of nuclear chromatin, and consequent degradation of the nucleus and the whole cell. Changes in ultrastructure of mitochondria and plastids included swelling and disorganization of inner membranes, and complete degradation. Another typical change resulting from treatment with sodium selenate (IV) was increased vacuolation of cells. Additionally, some vacuoles contained electron-dense deposits. Similar changes in the shape of cell nuclei, condensation of nuclear chromatin and alterations in mitochondria of Allium test cells were observed following incubation in extract of Taxus baccata (Majewska et al. Citation2002) and roots of Rhodiola rosea (Majewska et al. Citation2003). Increased vacuolation of cytoplasm is a response of cells to a foreign chemical compound infiltrating the cell. Plant cells have a strongly developed mechanism of detoxification, with the formation of additional spaces of apoplast for storage of toxic or unnecessary substances in a cell (Antosiewicz and Wierzbicka Citation1999). Simultaneous changes in activity of the endoplasmic reticulum might result from synthesis of vacuolar or secretion proteins within it, playing a lytic role and connected with cell defence response. On the other hand, increased vacuolization of cells, appearance of lipid bodies and strong condensation of nuclear chromatin together with its specific degradation, are typical changes that may indicate triggering of the programmed cell death mechanism. Similar changes were observed in plants, e.g. during the abortion of three of four megaspores, which was already recognized as the result of a programmed cell death (PCD) process in Larix leptolepis (Pinaceae) (Cecchi Fiordi et al. Citation2002) and Tillandsia (Bromeliaceae) (Papini et al. Citation2011), or during degeneration of nucellus cells in Tillandsia (Bromeliaceae) (Brighigna et al. Citation2006). Our study indicates that higher concentration and longer incubation period in the solution of sodium selenate IV could induce the programmed death process in meristematic Allium cepa cells, which resembles apoptotic process in animals, and leads to formation of apoptotic bodies. In plant cells, the PCD process may take various forms (Krishnamurthy et al. Citation2000). Remarkably, as confirmed by our previous work (Ślusarczyk et al. Citation2014), cells treated with Selol cease division; however, the structure of their interphase nuclei is unchanged. Only the high concentration solution (about four times higher) causes changes in structure of cell nuclei. This may mean that the effect of Selol is much more specific than the one of sodium selenate (IV), and does not cause general intoxication of cells. This is reflected by the fact that treatment with even the lowest concentrations of Selol (25 and 50 μg ml−1 Se) caused changes in shape of cell nuclei, which intensified following incubation with higher concentrations of Selol. Deformation of nuclei was accompanied by increasing condensation of chromatin. As shown in our earlier studies comparing the effect of both compounds on structure of chromosomes and mitotic activity, such treatment results in chromosome contraction and condensation (cc chromosomes), i.e. turbogenic changes, but not mutagenic ones. Thus, unlike sodium selenate (IV), Selol is not toxic to cells.
Analysis of ultrastructural changes showed high toxicity of sodium selenate (IV), with degradation of nucleus and cytoplasm. Selol did not induce such dramatic changes in ultrastructure. The results obtained indicate that Selol is much less toxic than sodium selenate (IV), and should be investigated as a potential safe anticancer drug.
Funding
This work was supported by Jan Kochanowski University [612464].
Disclosure statement
No potential conflict of interest was reported by the authors.
References
- Abdulah R, Miyazaki K, Nakazawa M, Koyama H. 2005. Chemical forms of selenium for cancer prevention. J Trace Element Med Biol. 19(2–3):141–150.
- Antosiewicz DM, Wierzbicka M. 1999. Localization of lead in Allium cepa L., cell by electron microscopy. J Microsc. 195:139–146.
- Brighigna L, Milocani E, Papini A, Vesprini JL. 2006. Programmed cell death in the nucellus of Tillandsia (Bromeliaceae). Caryologia. 59(4):334–339.
- Brown TA, Shrift A. 1982. Selenium: toxicity and tolerance in higher plants. Biol Rev. 57(1):59–84.
- Cecchi Fiordi A, Papini A, Brighigna L. 2002. Programmed cell death of the nonfunctional megaspores in Larix leptolepis (Sieb Et Zucc.) Gordon (Pinaceae): ultrastructural aspects. Phytomorphology. 52(2–3):187–195. ISSN .
- Clark LC, Marshall JR. 2001. Randomized, controlled chemoprevention trials in populations at very high risk for prostate cancer: elevated prostate-specific antigen and high-grade prostatic intraepithelial neoplasia. Urology. 57(4a):185–187.
- Costello AJ. 2001. A randomized, controlled chemoprevention trial of selenium in familial prostate cancer: rationale, recruitment, and design issues. Urology. 57(4a):182–184.
- Dorgan JF. 1998. Relationships of serum carotenoids, retinol, cc-tocopherol, and selenium with breast cancer risk: results from a prospective study in Columbia, Missouri (United States). Cancer Causes Control. 9(l):89–97.
- Early DS, Hill K, Burk R, MD., Palmer I. 2002. Selenoprotein levels in patients with colorectal adenomas and cancer. Am J Gastroenterol. 97(3):745–748.
- El-Bayoumy K, Sinha R. 2004. Mechanisms of mammary cancer chemoprevention by organoselenium compounds. Mutat Res. 551(1–2):181–197.
- Fitak BA, Grabowski M, Suchocki P. 1999. Patent–B. Preparat przeciwnowotworowy i sposób jego wytwarzania. Pol. PL, 176530.
- Floriańczyk B. 1999. Selen i selenoproteiny w zdrowiu i w chorobie. Nowiny Lekarskie. 68(2):244–253.
- Gabara B, Kalwinek J, Koziróg A, Żakowska Z, Brycki B. 2006. Influence of N, N-bis (aminopropylo)dodecyloamine on the ultrastructure of nuclei in Aspergillus niger mycelium and on cell proliferation and mitotic disturbances in Allium cepa L. root meristem. Acta Biol Cracov Ser Bot. 48(1):45–52. ISSN .
- Ganther HE. 1999. Selenium metabolism, selenoproteins and mechanisms of cancer prevention: complexities with thioredoxin reductase. Carcinogenesis. 20(9):1657–1666.
- Gasmi A, Garnier R, Galliot-Guilley M, Gaudillat C, Quartenoud B, Buisine A, Djebbar D. 1997. Acute selenium poisoning. Veterinary and Human Toxicology. 39(5):304–308.
- Gromadzińska J, Reszka E, Bruzelius E, Wąsowicz W, Akesson B. 2008. Selenium and cancer: biomarkers of selenium status and molecular action of selenium supplements. Eur J Nutrit. 47(Suppl 2):29–50. doi:10.1007/s00394-008-2005-z.
- Hartikainen H, Xue T. 1999. The promotive effect of selenium on plant growth as trigged by ultraviolet irradiation. J Environ Qual. 28:1272–1275. doi:10.2134/jeq1999.00472425002800040043x.
- Hawrylak B, Szymańska M. 2007. Wybrane aspekty antyoksydacyjnej i prooksydacyjnej aktywności selenu w korzeniach kukurydzy (Zea mays L.). Roczniki Akademii Rolniczej w Poznaniu. Rocz. AR Pozn. 41:487–491. ISSN .
- Jiang C, Wang Z, Ganther H, Lu J. 2001. Caspases as key of methyl selenium – induced apoptosis (Anoiks) of DU – 145 prostate cancer cells. Cancer Res. 61(7):3062–3070.
- Krishnamurthy KV, Krishnaraj R, Chozhavendan R, Samuel CF. 2000. The programme of cell death in plants and animals – a comparison. Curr Sci. 79(9):1169–1118.
- Kuraś M. 1984. Action of rape (Brasseca napus L.) embryo during seed germination. III. Ultrastructure of dry embryo axis. Acta Soc Bot Pol. 53(2):171–186.
- Kuraś M, Nowakowska J, Śliwińska E, Pilarski R, Ilasz R, Tykarska T, Zobel A, Gulewicz K. 2006. Changes in chromosome structure, mitotic activity and nuclear DNA content from cells of Allium test induced by bark water extract of Uncaria tomentosa (Willd.) DC. J Ethnopharmacol. 107:211–21.
- Lemasters J, Nieminen A, Qian T, Trost L, Herman B. 1997. The mitochondrial permeability transition in toxic, hypoxic and reperfusion injury. Mol Cell Biochem. 174(1–2):159–165.
- Levan A. 1938. The effect of colchicine on root mitoses in Allium. Hereditas. 24(4):471–486. doi:10.1111/j.1601-5223.1938.tb03221.x.
- Majewska A, Furmanowa M, Głowniak K, Guzewska J, Zobel A, Kuraś M. 2002. Influence of extract from shoots of Taxus baccata var. elegantissima on ultrastructure and tubulin cytoskeleton of meristematic cells of Allium cepa L. roots. Acta Soc Bot Pol. 71(3):211–221.
- Majewska A, Wolska E, Furmanowa M, Urbańska N, Pietrosiuk A, Zobel A, Kuraś M. 2003. Antimitotic effect, G2/M accumulation, chromosomal and ultrastructure changes in meristematic cells of Allium cepa L. root tips treated with the extract from Rhodiola rosea rhizomes. Caryologia. 56:333–347. doi:10.1080/00087114.2003.10589343.
- Papini A, Mosti S, Milocani E, Tani G, Di Falco P, Brighigna L. 2011. Megasporogenesis and programmed cell death in Tillandsia (Bromeliaceae). Protoplasma. 248:651–662.
- Reynolds ES. 1963. The use of lead citrate at high pH as an electron opaque stain in electron microscopy. J Cell Biol. 17(1):208–212.
- Seppänen M, Turakainen M, Hartikainen H. 2003. Selenium effects on oxidative stress in potato. Plant Sci. 165:311–319.
- Ślusarczyk J, Dudek M, Wierzbicka M, Suchocki P, Kuraś M. 2014. Antimitotic effect of Selol and sodium selenate (IV) on Allium test cells. Caryologia. 67(3):250–259.
- Ślusarczyk J, Wierzbicki A, Przewłoka M, Tykarska T, Kuraś M. 2003. Influence of change in the proportion of H1 histone variants on microsporogenesis and development of male gametophyte in transgenic plants of tobacco (Nicotiana tabacum L.). Acta Soc Bot Pol. 72(1):25–35.
- Sochacka M, Giebułtowicz J, Remiszewska M, Suchocki P, Wroczyński P. 2014. Effects of Selol 5% supplementation on the activity or concentration of antioxidants and malondialdehyde level in the blood of healthy mice. Pharmacol Rep. 66:301–310.
- Stewart MS, Spallholz JE, Neldner KH, Pence BC. 1999. Selenium compounds have disparate abilities to impose oxidative stress and induce apoptosis. Free Radical Biol Med. 26:42–48.
- Suchocki P, Jakoniuk D, Fitak BA. 2003. Specific spectrophotometric method with trifluoroacetic acid for the determination of selenium (IV) in selenitetriglycerides. J Pharm Biomed Anal. 32(4):1029–1036.
- Suchocki P, Misiewicz I, Skupińska K, Wacławek K, Fijałek Z, Kasprzycka-Guttman T. 2007. The activity of Selol in multidrug – resistant and sensitive human leukemia cells. Oncol Rep. 18:893–899.
- Terry N, Zayed M, De Souza MP, Tarun AS. 2000. Selenium in higher plants. Annu Rev Plant Physiol Plant Mol Biol. 51:401–432.
- Van Zandwijk N, Hirsch FR. 2003. Chemoprevention of lung cancer: current status and future prospects. Lung Cancer. 42(2):71–79. doi:10.1016/S0169-5002(03)00307-6.
- Wachowicz B. 1993. Selen w roślinach. Wiadomości Botaniczne. 37:87–89.
- Whanger PD. 2002. Selenocompounds in plants and animals and their biological significance. J Am Coll Nutrit. 21(3):223–232.
- Wierzbicka M, Pyrzyńska K, Bulska E. 2007. Selen – pierwiastek ważny dla zdrowia, fascynujący dla badacza. Warsaw: Malamut Press. ISBN 978-83-925269-0-2.
- Wolf C, Lewis A, Adams D, Allan D, Ansel D. 1997. The role glutathione in determining the response of normal and tumors cells to anticancer drugs. Biochem Soc Trans. 15:728–730.
- Xue T, Hartikainen H, Piironen V. 2001. Antioxidative and growth-promoting effect of selenium in senescing lettuce. Plant Soil. 237:55–61.
- Zagrodzki P. 2000. Selen w żywieniu człowieka. Bromatol Chem Toksykol. 33:209–214.
- Zagrodzki P, Bik D, Fitak B, Suchocki P, Niemczuk K. 2000. Selenoenzymes in animal tissues after supplementation with Selol. Bull Vet Inst Pulawy. 44(2):215–220.
- Żbikowska HM. 1997. Metabolizm selenu w komórce i organizmie człowieka. Postępy Biol Komórk. 24(3):303–313.
- Zhu Z, Kimura M, Itokawa Y, Nakatsu S, Oda Y, Kikuchi H. 1995. Effect of selenium on malignant tumor cells of brain. Biol Trace Element Res. 49(1):1–7.
- Zwolak I, Zaporowska H. 2012. Selenium interactions and toxicity: a review. Cell Biol Toxicol. 28:31–46.