Abstract
Lycopene is an acyclic isomer of β-carotene and it is mainly found in tomatoes. It is a potent singlet oxygen-quenching carotenoid and one of the most potent antioxidants. In addition to its antioxidant properties, lycopene shows various biological effects including anti-mutagenic and anti-carcinogenic activities. Pesticides form an important group of man-made noxious chemicals. Their potential synergistic or antagonistic side effects in humans have not yet been extensively investigated. In this study, the protective effect of lycopene against cytotoxic and genotoxic effects of two pesticide mixtures (lambda-cyhalothrin (LCT) and dimethoate (DIMET)) on human peripheral blood lymphocytes have been investigated using the Trypan blue exclusion and comet assays. For this purpose, human lymphocytes have been treated with 5 μg ml−1 and 10 μg ml−1 lycopene concentrations for 1 h. After lycopene treatment, lymphocytes were treated with pesticides DIMET (1 and 2 μl ml−1) and LCT (0.15 and 0.3 μl ml−1) and mixtures of 1 μl ml−1 DIMET + 0.15 μl ml−1 LCT and 2 μl ml−1 DIMET + 0.3 μl ml−1 LCT (1:1 v/v) for 2 h. DMSO was used in 0.1% as negative control, and 0.1 mM H2O2 was used as positive control. As a result, it was found that lycopene has a protective benefit against cytotoxic and genotoxic effects of pesticides and pesticide mixtures. In this context, 5 μg ml−1 lycopene concentration was found to be more effective than 10 μg ml−1 lycopene concentration (p < 0.05, ANOVA).
1. Introduction
For centuries, several hundred pesticides have been used in agricultural practice in order to enhance food production by eradicating unwanted insects and controlling disease vectors (Anwar Citation1997). Unfortunately this situation has resulted in some negative side effects on human health and on the environment. Many people are potentially at risk of becoming intoxicated when using pesticides in agriculture, in health programs and even at home. Moreover, people trying to commit suicide by using pesticides, and some consumers eating pesticide-contaminated foods are at risk, due to easy accessibility of pesticides and high levels of pesticide residues found in, for example vegetables on the market (Konradsen et al. Citation2003; Hamilton et al. Citation2004; Maroni et al. Citation2006). The World Health Organization (WHO Citation1992, Citation1999) reported that around three million pesticide poisoning cases occur annually and result in 220,000 deaths worldwide. Many of these chemicals are mutagenic (Galloway et al. Citation1987; Garaj-Vrhovac and Zaljezic Citation2000).
Chemical pesticides are widely used in modern agriculture alone or in combination and they represent an important group of environmental pollutants. Recently, it has been demonstrated that pesticides and many other industrial chemicals may induce free radical production into experimental animals, which possibly play an important role in causing toxicity. Occupational or environmental exposure to mixtures of pesticides has been associated with increased genotoxicity in human lymphocytes (Rupa et al. Citation1989; De Ferrari et al. Citation1991; Carbonell et al. Citation1995; Gómez-Arroyo et al. Citation2000; Shaham et al. Citation2001; Pastor et al. Citation2001; Bolognesi et al. Citation2002, Citation2004). Although there are many studies on the genotoxicity of individual insecticides, very limited data are available on the genotoxicity of insecticide mixtures in the literature. Unfortunately, predicting the toxicity of pesticide mixtures based on the results of the toxicity of a single component is not possible (Marinovich et al. Citation1996). Some pesticides individually induce genotoxicity and some other pesticide mixtures have much greater genotoxic effects. Exposure to known genotoxic compounds can induce DNA damage not only directly but also through other mechanisms such as oxidative stress or inflammatory processes (Lebailly et al. Citation1998, Shadnia et al. Citation2005; Hernández et al. Citation2013). It has been shown that pesticides may induce oxidative stress by producing free radicals, enhancing lipid peroxidation, causing drastic increase in the activities of antioxidant enzymes and by forming a pool of vital antioxidant components in mammalian systems (Banerjee et al. Citation1999; Nordberg and Arner Citation2001; Etemadi-Aleagha et al. Citation2002; Rai and Sharma Citation2007). On the other hand, some epidemiological studies have shown the association between chronic exposure to pesticides and recorded cases of human malignancy (Safi Citation2002).
The endogenous antioxidant defenses are inadequate to reduce the pesticide-induced free radical overproduction. Antioxidant intervention seems to inhibit or reduce free radical toxicity and therefore offers protection against pesticides. A number of dietary antioxidants have been reported to decrease free radical attack on biomolecules (El-Habit et al. Citation2000).
Lycopene is an acyclic carotenoid with 11 linearly arranged conjugated double bounds (Clinton Citation1998). A naturally occurring carotenoid in tomatoes and tomato products, it has attracted considerable attention as a potential chemo-preventive agent. Recently, lycopene has become the focus of much interest because of its highly efficient antioxidant scavenging activity against singlet-oxygen and free radicals (Cohen Citation2002; Heber and Lu Citation2002; Jonker et al. Citation2003; Michael and Bausch Citation2003; Stahl and Sies Citation2003; Tapiero et al. Citation2004; Velmurugan et al. Citation2004; Wertz et al. Citation2004). This antioxidant activity is a potential mechanism by which lycopene may contribute to the prevention of a range of oxidative damage, toxicity, and diseases. Lycopene has an antioxidant activity due to its free radical-scavenging ability (Di Mascio et al. Citation1989; Srinivasan et al. Citation2007; Alshatwi et al. Citation2010). Experimental evidence suggests that lycopene can quench singlet oxygen, scavenge free radicals of nitrogen dioxide (NO2), thiyl (RS) and sulphonyl (RSO2). Because of its antioxidant properties, lycopene has gained attention and become one of the most studied chemo-preventive agents (Scolastici et al. Citation2007). The available reports have shown that little or no work has been carried out on the protective effects of lycopene against pesticide-induced toxicity in human lymphocytes.
The aim of this study, in view of the known antioxidant effects of lycopene, is to evaluate the possible protective effects of lycopene on different concentrations of two pesticide mixtures, dimethoate (DIMET), which is an organophosphate pesticide (OP); and lambda-cyhalothrin (LCT), which is a Type II synthetic pyrethroid, against the cytotoxic and genotoxic effects induced in human peripheral blood lymphocytes.
2. Materials and methods
2.1. Chemicals
The chemicals dimethylsulfoxide (DMSO), RPMI 1640 medium, Histopaque 1077, phosphate-buffered saline (PBS), Trypan blue, Triton X-100, low melting point agarose, normal melting agarose and ethidium bromide were purchased from Sigma Aldrich (St Louis, MO, USA). Korumagor 40 EC (dimethoate EC 400 g l−1, Koruma, İzmit, Turkey) and Karate Zeon (lambda-cyhalothrin 50 g l−1, Syngenta, İzmir, Turkey) were purchased from a local agricultural drug store in Aydın, Turkey. Lycopene (Lyc-O-Mato Lycored, Soft Gel Technologies, Inc., LA, CA, USA) was purchased from Vega Natural Products, Konya, Turkey.
2.2. Preparation of the lycopene solution
We bought the lycopene as soft gels (30 soft gels in a package) and each soft gel contained 10 mg lycopene. Lycopene (5 mg) was dissolved in 12.5 μl dimethylsulfoxide (DMSO) and made up to 5 ml sterile distilled water which was preheated to 37°C. The stock solution of lycopene was 1 mg ml−1. From this stock, 5 and 10 μg ml−1 concentrations were used in 1 ml lymphocyte culture (RPMI). The final concentration of DMSO in the lymphocyte culture was 0.1%. In the experiment, 0.1% DMSO was used as solvent control.
2.3. Isolation of lymphocytes
The peripheral blood of one volunteer was taken after free informed consent. A 5 ml blood sample was aseptically collected in heparinized sterile glass tube from one 30-year-old non-smoking healthy female individual not exposed to radiation or drugs. The volunteer did not present disease symptoms and reported not having used prescription drugs for at least 30 days prior to collection. Lymphocytes were isolated from blood using Histopaque-1077 (Sigma) and cultured as described previously (Boyum Citation1968). Blood was diluted 1:1 with phosphate-buffered saline (PBS) and layered onto the Histopaque in the ratio of 4:3 (blood + PBS: Histopaque). The blood was centrifuged at 400 ×g for 30 min at room temperature. The lymphocyte layer was removed and washed twice in PBS at 250 ×g for 10 min. Then, the cells were washed with RPMI-1640 media. Each culture contained 1 × 103 cells ml−1 culture medium.
2.4. Treatment of lymphocytes with lycopene and pesticides
After 24 h following the culture initiation, lymphocytes were treated with 5 and 10 μg ml−1 concentrations of lycopene at 37°C for 1 h. After 1 h lycopene treatment, the lymphocytes were treated with two concentrations of pesticides (1 and 2 μl ml−1 DIMET, 0.15 and 0.30 μl ml−1 LCT) and pesticide mixtures (PM) – 1 μl ml−1 dimethoate + 0.15 μl ml−1 LCT and 2 μl ml−1 dimethoate + 0.30 μl ml−1 LCT – at 37°C for 2 h. In the experiment, 0.1% DMSO was used as solvent control; and 0.1 mM hydrogen peroxide (H2O2) (a direct acting mutagen) was used as positive control.
2.5. Cytotoxicity determination by Trypan blue dye exclusion assay
For the determination of cytotoxicity in lymphocytes, viable cell numbers were determined using the Trypan blue exclusion assay (Son et al. Citation2003; Lee et al. Citation2005). Trypan blue was added to all lymphocyte cultures in a ratio of 1:1 after the lycopene and pesticide treatments. The preparations were examined under a standard light microscope at 100× magnification. The ratio of live cells to dead cells (cell viability) was determined and the results were expressed as a percentage.
2.6. Single cell gel electrophoresis (comet assay)
The DNA damage in lymphocytes was determined by alkaline comet assay (Singh et al. Citation1988). After the lycopene and pesticide treatments, lymphocytes were suspended in 150 μl of molten 0.5% low melting-point agarose (LMPA) in PBS (phosphate buffered saline) without calcium and magnesium. Then 150 μl aliquots of the cell suspension were rapidly spread on three slides pre-coated with 85 μl of 1% normal-melting agarose (NMA) and cover-slipped (24 × 50 mm). After the agarose was allowed to solidify for 5 min at 0°C the coverslips were gently removed and a third layer of 75 μl LMPA was added. The slides were then placed in a tank fiLMPA with lysis solution (2.5 M NaCl, 0.1 M EDTA, pH 10, 10% DMSO and 1% Triton X-100 both freshly added) at 4°C. After 1 h, the slides were removed from the lysis solution and incubated in fresh electrophoresis buffer (0.3 M NaOH and 1 M EDTA, pH > 13) for 20 min at room temperature for unwinding of DNA. Electrophoresis was then carried out at room temperature in the same electrophoresis buffer for 30 minutes at 0.7 V/cm and 300 mA. After electrophoresis, the slides were gently washed twice for 5 min in fresh neutralization buffer (0.4 M Tris-HCL, pH 7.59) followed by dehydration in absolute methanol. The slides were stained with 75 μl 1× ethidium bromide and cover-slipped. For each treatment, 100 randomly selected cells from each of the three slides (300 cells per concentration) were evaluated for DNA damage visually using a 40× objective on a flbromide a microscope (Olympus BX 51; Olympus, USA). The DNA damage was quantified by visual classification of cells into fiof categories of “comets” corresponding to the tail lengths (Anderson et al. Citation1994): Type 0, undamaged; Type I, low-level damage; Type II, medium-level damage; Type III, high-level damage; and Type IV, complete damage. From the arbitrary values assigned to the different categories (from Type 0 = 0 and Type 4 = 4), a genetic damage index (GDI) was calculated for each concentration (Pitarque et al. Citation1999). GDI values were calculated based upon differential weightings given to the different grades of damage for five arbitrary categories from Type 0 (undamaged) to Type IV (highly damaged). This categorization was used to obtain quantitative measures for the slide which was based on a weighting which was applied to the number of cells with the different grades of damage where the GDI= (Type I + 2 × Type II + 3 × Type III + 4 × Type IV) / (Type 0 + I + II + III + IV).
2.7. Statistical analysis
All the experiments were performed in triplicate form, and were analyzed by one way ANOVA (SPSS 11.5 software package). The statistically significant difference was considered to be p < 0.05. The normality of variables was evaluated using the Kolmogorov–Smirnov Z test. The statistical differences between the control and treatment groups were carried out using the non-parametric Mann–Whitney test (for independent samples). The correlations between different variables were determined using the Spearman rank correlation test.
3. Results
The protective effect of lycopene against the cytotoxic effects of DIMET and LCT and mixture of two pesticides (DIMET and LCT) on human peripheral blood lymphocytes were determined by the Trypan blue dye exclusion method. The viability percent of the cells decreased with the increase in the concentration of the pesticide. The results are presented in Table and Figure .
Table 1. Cytotoxicity in human peripheral blood lymphocytes treated with pesticide mixtures and lycopene.
Figure 1. Cytotoxicity in human peripheral blood lymphocytes treated with pesticide mixtures and lycopene.
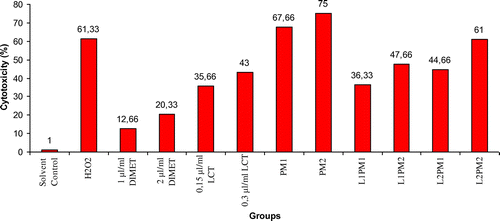
Then, with 1 μl ml−1 and 2 μl ml−1 dimethoate treatment on lymphocytes, cell death rates were increased based on the concentration. As indicated in Table , especially after the 2 μl ml−1 dimethoate treatment, the cytotoxic effects of the pesticides on lymphocytes was found to be significant in comparison with the solvent control (20.33% and 1.00%, respectively). LCT showed the cytotoxic effect on lymphocytes. This effect increased parallel to the increasing pesticide concentration. The cytotoxic effect of 0.15 μl ml−1 and 0.3 μl ml−1 concentrations of LCT on lymphocytes were found significantly high when compared with the solvent control (p < 0.05). Also, LCT was found more cytotoxic than dimethoate.
When lymphocytes were treated with various concentrations of the pesticide mixtures (1 μl ml−1 dimethoate + 0.15 μl ml−1 LCT and 2 μl ml−1 dimethoate + 0.30 μl ml−1 LCT) for 2 h, it was found out that cell survival rates were decreased significantly in comparison with the DIMET, LCT and solvent control (DMSO). Pesticide mixtures and H2O2 exhibited significant inhibition of cell viability (p < 0.05). As shown in Table , the cytotoxic effects of the pesticide mixtures was linearly correlated with the concentration (67.66% and 75.00%, respectively), and this effect was higher than the positive control H2O2 (61.33%), which was used as the positive control group. Furthermore, the cytotoxic effects of the pesticide mixtures was significantly higher than the individual cytotoxic effects of DIMET (12.66 and 20.33%), LCT (35.66 and 43.00%).
The cytotoxic effects of individual pesticides and pesticide mixtures were decreased in the lymphocytes which were treated with lycopene before the pesticides and pesticide mixture treatments. The findings of this study suggest that the protective effects of a 5 μg ml−1 concentration of lycopene against the cytotoxic effects of two pesticides and their mixtures was higher than at the 10 μg ml−1 concentration (Table , Figure ).
Genotoxicity assessments using the comet assay indicated that both pesticide mixtures could increase the DNA damage at concentrations much below the individual treatments. The results of the damaged nuclei, the genetic damage index and the arbitrary units as measured in the alkaline comet assay are shown in Table . Although the DNA damage could be monitored at higher concentrations of pesticides when used individually, the same extent of damage could also be obtained at very low concentrations of pesticide mixtures (Table ).
Table 2. Analysis of DNA damage as measured by comet assay in human peripheral blood lymphocytes treated with pesticide mixtures and lycopene.
Dimethoate at concentrations of 1 μl ml−1 and 2 μl ml−1 induced DNA damage in lymphocytes, and the DNA damage increased based on the concentration. The LCT also induced the DNA damage in lymphocytes, which increased with increasing pesticide concentration. The DNA damaging effect of 0.15 μl ml−1 and 0.3 μl ml−1 concentrations of LCT in lymphocytes was found to be significantly higher than with 1 μl ml−1 and 2 μl ml−1 concentrations of dimethoate.
It was found that all the concentrations of the pesticide mixtures induced significant DNA damage in lymphocytes compared to the solvent control group (p < 0.05) (Table , Figure ). In particular, the DNA damage percentage in lymphocytes reached 73.66% after treatment with the 2 μl ml−1 dimethoate + 0.30 μl ml−1 LCT mixture. The genetic damage index and the arbitrary unit values also increased in pesticide treatment groups based on the concentration and in positive control group (H2O2) (69.67%) compared to the solvent control group, and reached statistically significant level, mainly by the increased percentage of type II, III and IV damages (p < 0.05). The DNA damaging effect of the pesticide mixtures on lymphocytes decreased lycopene treatment before the treatment of pesticide mixtures. It was found that the protective effects of a 5 μg ml−1 concentration of lycopene against the DNA damaging effect of the two pesticide mixtures was higher than with 10 μg ml−1 lycopene (Table ).
4. Discussion
Pesticides may induce the oxidative stress, leading to the generation of free radicals and causing lipid peroxidation, and may be the underlying molecular mechanism that gives rise to pesticide-induced toxicity (Agrawal et al. Citation1991; Khrer Citation1993; Almeida et al. Citation1997; Köprücü et al. Citation2008). The increased lipid peroxidation and oxidative stress can affect the activities of a number of protective enzymatic and non-enzymatic antioxidants that are known to be sensitive indicators of the increased oxidative stress.
Different pesticide formulations are often used simultaneously in complex mixtures, including a significant number of genotoxic compounds (Bolognesi Citation2003). Organophosphate and pyrethroid pesticides have been shown to pose a significant risk of adverse DNA effects (Bhali et al. Citation2009). Occupational exposure to mixtures of pesticides has been associated with an increase in genotoxic damage. The cytogenetic damage induced by pesticides appears to depend on the degree of exposure. A dose–response relationship can be hypothesized. Negative results have been associated with the low levels of exposure. By contrast, clear positive results were reported in populations which were subjected to high exposure levels. People suffering from severe intoxication as a result of attempted suicide or employees neglecting occupational safety measures during the spraying season are typical victims in this context.
Dimethoate is one of the most important OPs used extensively on a large number of crops against several pests (Gargouri et al. Citation2011; Ayed-Boussema et al. Citation2012). In acute exposure, organophosphate pesticides have the ability to induce toxicity in mammals through inhibition of acethylcholin esterase (AChE) (Hazarika et al. Citation2003; Ayed-Boussema et al. Citation2012). In chronic and sub-chronic exposures, in addition to acethylcholin esterase inhibition, the induction of oxidative stress has been reported as the main mechanism of OP toxicity (Banerjee et al. Citation2001; Ranjbar et al. Citation2002; Abdollahi et al. Citation2004; Gargouri et al. Citation2011). Other mechanisms responsible for the genotoxicity include,those related to the alkylating (Dedek et al. Citation1984; Mehl et al. Citation2000) and phosphorylating properties (Piña-Guzmán et al. Citation2005).
LCT is a third generation type-II synthetic pyrethroid containing 3-cyno group (Meister Citation1992). The genotoxic effects of synthetic pyrethroids depend on the test system or organism used in experiments. According to Bhunya and Pati (Citation1988), cypermethrin causes micronuclei in mouse erythrocytes. Miadokova et al. (Citation1992) also reported that supercypermethtin led to gene conversion in Saccharomyces cerevisiae and to increasing frequency of aberrant anaphase-telophase in root tips of Hordeum vulgare and Vicia faba.
The findings of this study suggest that the cytotoxicity and genotoxicity (i.e. the DNA damage) in positive control (H2O2) group, pesticides (DIMET and LCT) and pesticide mixtures (DIMET+ LCT) treatment groups increased significantly compared with the solvent control group. Organophosphates and pyrethroids have been reported to be genotoxic and as generating free radicals that react with the cell membranes and initiate the process of lipid peroxidation. The accumulation of these radicals can cause oxidative stress (Hérnandez et al. Citation2005; Salvador et al. Citation2008). Gargouri et al. (Citation2011) suggested that dimethoate has cytotoxic effects on human lymphocytes, which was evidenced by increasing lipid peroxidation and protein oxidation as well as antioxidant enzyme activities such as superoxide dismutase (SOD) and catalases (CAT). Our study showed that DIMET, LCT and their mixture may disrupt the structure and function of SOD and CAT in lymphocytes. Thus, increased oxidative stress may cause cytotoxicity and DNA damage in lymphocytes.
Moreover, we found decreased cytotoxicity and DNA damage in lymphocytes pretreated with lycopene before the treatments with pesticides and pesticide mixtures (DIMET+ LCT) in comparison with the pesticide mixtures treated lymphocytes. This might be due to the protective effect of the lycopene, because lycopene has the ability to act as a powerful antioxidant and singlet oxygen quencher, thereby inhibiting the destructive effects of the ROS (Rao and Agarwal Citation1998). Because of its antioxidant properties, lycopene has gained attention and has become one of the most studied chemo-preventive agents. The high number of conjugated dienes makes lycopene one of the most potent singlet oxygen quenchers among natural carotenoids (Di Mascio et al. Citation1989; Matos et al. Citation2006; Abdul-Hamid and Salah Citation2013). It has been suggested that lycopene can prevent carcinogenesis by protecting critical biomolecules including the DNA (Di Mascio et al. Citation1989). Lycopene may protect lipids, proteins, and DNA against oxidation in vivo (Reifen et al. Citation2004; Tapiero et al. Citation2004). Scolastici et al. (Citation2007, Citation2008) added that lycopene is a suitable agent for preventing chemically induced DNA and chromosome damage. Weng Kong et al. (Citation2010) reported that lycopene protected cells against DNA damage.
Matos et al. (Citation2001) reported that lycopene supplementation decreased 77% of 8-oxo-dGuo levels in Fe-NTA/ascorbate-treated cells. These results indicate that lycopene provides strong protection against DNA-based oxidation. Furthermore, consumption of tomato products has been reported to reduce the susceptibility of lymphocyte DNA to oxidative damage (Riso et al. Citation1999). Our previous studies also suggested that pre- and post-treatment with lycopene reduced total chromosomal aberrations induced by ethyl methane sulfonate in Allium cepa (Aslantürk and Aşkın Çelik Citation2005, Citation2006).
It is known that mechanisms underlying the inhibitory effects of lycopene on carcinogenesis and mutagenesis may involve ROS scavenging, up-regulation of detoxification systems, interference with cell proliferation, induction of gap-junctional communication, inhibition of cell cycle progression, and modulation of signal transduction pathways (Bhuvaneswari and Nagini Citation2005). Among the effects of lycopene, reduction of DNA damage (Yeh and Hu Citation2000; Reddy et al. Citation2006), induction of apoptosis (Muller et al. Citation2002; Reddy et al. Citation2006) and inhibition of cell proliferation (Tang et al. Citation2005; Nahum et al. Citation2006) have been described.
In our present study, the higher concentration of lycopene (10 μg ml−1) has been shown to be effective against pesticide mixture-induced cyto- and genotoxicity, but not as effectively as 5 μg ml−1. This may be because this concentration may have resulted in byproducts which interfered with the antioxidant activity of lycopene, thus decreasing its effect. Hwang and Bowen (Citation2005) studied the effect of lycopene on LNCaP human prostate carcinoma cell line and asserted that lycopene in low concentration showed only a lipid antioxidant effect, whereas at higher concentrations its inhibitory effect on cancer cells was mediated through increased DNA damage. Moreover, 10 μg ml−1 lycopene could behave also as pro-oxidant. Yeh and Hu (Citation2000) reported that lycopene and β-carotene could be pro-oxidant depending on their applied dosage and the type of the oxidant used in Hs68 cells. Srinivasan et al. (Citation2009) also reported that 1, 5 and 10 μg ml−1 lycopene had radio-protective effect against 1, 2 and 4 Gy γ-radiation-induced cellular damages in cultured human lymphocytes; however, they also added that 5 μg ml−1 of lycopene was more effective than the other concentrations.
Our results show that pretreatment with lycopene protects the lymphocytes against cytotoxic and genotoxic effects induced by pesticides and pesticide mixture. Lycopene could be a suitable agent for preventing the toxicity of DIMET and genotoxicity-induced DNA damage. According to our results and to other reports about the potential health benefits of lycopene, consumption of lycopene-rich foods can help protect health against exogenous harmful effects. For more information and clear conclusions about the protective effects of lycopene against exogenous harm, further studies should be performed with different test systems.
Acknowledgments
This study was performed in Science and Technology Research Centre (BILTEM), Adnan Menderes University, Aydın, Turkey.
Disclosure statement
No potential conflict of interest was reported by the authors.
References
- Abdollahi M, Ranhbar A, Shadnia S, Nikfar S, Rezaiee A. 2004. Pesticides and oxidative stress: a review. Med Sci Monit. 10(6):141–147.
- Abdul-Hamid M, Salah M. 2013. Lycopene reduces deltamethrin effects induced thyroid toxicity and DNA damage in albino rats. J Basic Appl Zool. 66(4):155–163.
- Agrawal D, Sultana P, Gupta GSD. 1991. Oxidative damage and changes in the glutathione redox system in erythrocytes from rats treated with hexachlorocyclohexane. Food Chem Toxicol. 29(7):459–462.
- Almeida MG, Fanini F, Davino SC, Aznar AE, Koch OR, Barros SBM. 1997. Pro- and anti-oxidant parameters in rat liver after short-term exposure to hexachlorobenzene. Hum Exp Toxicol. 16(5):257–261.
- Alshatwi AA, Al Obaaid MA, Al Sedairy SA, Al-Assaf AH, Zhang JJ, Lei KY. 2010. Tomato powder is more protective than lycopene supplement against lipid peroxidation in rats. Nutr Res. 30(1):66–73.
- Anderson D, Yu TW, Philips BJ, Schmezer P. 1994. The effect of various antioxidants and other modifying agents on oxygen-radical-generated DNA damage in human lymphocytes in the comet assay. Mutat Res. 307:261–271.
- Anwar WA. 1997. Biomarkers and human exposure to pesticides. Environ Health Perspect. 4:801–806.
- Aslantürk ÖS, Aşkın Çelik T. 2005. Preventive effect of lycopene on chromosome aberrations in Allium cepa. Pak J Biol Sci. 8(6):482–486.
- Aslantürk ÖS, Aşkın Çelik T. 2006. Protective effect of lycopene on ethyl methane sulfonate induced chromosome aberrations in Allium cepa. Caryologia. 59(3):220–225.
- Ayed-Boussema I, Rjiba K, Mnasri N, Moussa A, Bacha H. 2012. Genotoxicity evaluation of dimethoate to experimental mice by micronucleus, chromosome aberration tests, and comet assay. Int J Toxicol. 31(1):78–85.
- Banerjee BD, Seth V, Ahmed RS. 2001. Pesticide-induced oxidative stress: perspective and trends. Rev Environ Health. 16(1):1–40.
- Banerjee BD, Seth V, Bhattacharya A, Pasha ST, Chakraborty AK. 1999. Biochemical effects of some pesticides on lipid peroxidation and free radical scavengers. Toxicol Lett. 107:33–47.
- Bhali JA, Ali T, Asi MR, Khalid ZM, Ceppi M, Khan QM. 2009. DNA damage in Pakistani agricultural workers exposed to mixture of pesticides. Environ Mol Mutagen. 50:37–45.
- Bhunya SP, Pati PC. 1988. Genotoxic effects of a synthetic pyrethroid insecticide, cypermethrin, in mice in vivo. Toxicol Lett. 41:223–230.
- Bhuvaneswari V, Nagini S. 2005. Lycopene: a review of its potential as an anticancer agent. Curr Med Chem Anticancer Agents. 5:627–635.
- Bolognesi C, Perrone E, Landini E. 2002. Micronucleus monitoring of a floriculturist population from western Liguria, Italy. Mutagenesis. 17:391–397.
- Bolognesi C. 2003. Genotoxicity of pesticides: a review of human biomonitoring studies. Mutat Res. 543:251–272.
- Bolognesi C, Landini E, Perrone E, Roggieri P. 2004. Cytogenetic biomonitoring of a floriculturist population in Italy: Micronucleus analysis by fluorescence in situ hybridization (FISH) with an all-chromosome centromeric probe. Mutat Res. 557:109–117.
- Boyum A. 1968. Isolation of mononuclear cells and granulocytes from human blood. Scand J Clin Lab Invest Suppl. 21:77.
- Carbonell E, Valbuena A, Xamena N, Creus A, Marcos R. 1995. Temporary variations in chromosomal aberrations in a group of agricultural workers exposed to pesticides. Mutat Res. 344:127–134.
- Clinton SK. 1998. Lycopene: chemistry, biology, and implications for human health and disease. Nutrit Rev. 562(1):35–51.
- Cohen LA. 2002. A review of animal model studies of tomato carotenoids, lycopene, and cancer chemoprevention. Exp Biol Med. 227:864–868.
- Dedek W, Grahl R, Schmidt RA. 1984. A comparative study of guanine N7-alkylation in mice in vivo by the organophosphorus insecticides trichlorphon, dimethoate, phosmet and bromophos. Acta Pharmacol Toxicol (Copenh). 55(2):104–109.
- De Ferrari M, Artuso M, Bonassi S, Bonatti S, Cavalieri Z, Pescatore D, Marchini E, Pisano V, Abbondandolo A. 1991. Cytogenetic biomonitoring of an Italian population exposed to pesticides: Chromosome aberration and sister-chromatid exchange analysis in peripheral blood lymphocytes. Mutat Res. 260:105–113.
- Di Mascio P, Kaiser S, Sies H. 1989. Lycopene as the most efficient biological carotenoid singlet oxygen quencher. Arch Biochem Biophys. 274(2):532–538.
- El-Habit OHM, Saada HN, Azab KHSH, Abdel Rahman M, El-Malah DF. 2000. The modifying effect of beta-carotene on gamma-irradiation-induced elevation of oxidative reactions and genotixicity in male rats. Mutat Res. 466:179–186.
- Etemadi-Aleagha A, Akhgari M, Abdollahi M. 2002. A brief review on oxidative stres and cardiac diseases. Mid East Pharmac. 10:8–9.
- Galloway SM, Armstrong MJ, Reuben C, Colman Brown SB, Cannon C, Bloom AD, Nakamura F, Ahmed M, Duk S, Rimpo J, Margolin BH, Resnick MA, Anderson B, Zeiger E. 1987. Chromosome aberrations and sister chromatid exchanges in Chinese hamster ovary cells, evaluation of 108 chemicals. Environ Mol Mutagen. 10:1–175.
- Garaj-Vrhovac V, Zaljezic D. 2000. Evaluation of DNA damage in workers occupationally exposed to pesticides using single-cell gel electrophoresis (SCGE) assay: pesticide genotoxicity revealed by comet assay. Mutat Res. 469:279–285.
- Gargouri B, Mansour BM, Abdallah FB, Elfekih A, Lassoued S, Khaled H. 2011. Protective effect of quercetin against oxidative stress caused by dimethoate in human peripheral blood lymphocytes. Lipids Health Dis. 10:149–152.
- Gómez-Arroyo S, Dvaz-Sánchez Y, Meneses-Pérez MA, Villalabos-Pietrini R, Leo´n-Rodrı´guez JD. 2000. Cytogenetic biomonitoring in a Mexican floriculture worker group exposed to pesticides. Mutat Res. 466:117–124.
- Hamilton D, Ambrus A, Dieterle R, Felsot A, Harris C, Petersen B, Racke K, Wong SS, Gonzales R, Tanaka K, Earl M, Roberts G, Bhula R. 2004. Pesticide residues in food—acute dietary exposure. Pest Manag Sci. 60:311–339.
- Hazarika A, Sarkar SN, Hajare S, Kataria M, Malik JK. 2003. Influence of malathion pretreatment on the toxicity of anilofos in male rats: a biochemical interaction study. Toxicology. 185:1–8.
- Heber D, Lu QY. 2002. Overview of mechanisms of action of lycopene. Exp Biol Med. (Maywood). 227:920–923.
- Hérnandez AF, López O, Rodrigo L, Gil F, Pena G, Serrano JL, Parrón T, Alvarez JC, Lorante JA, Pla A. 2005. Changes in erythrocyte enzymes in humans long term exposed to pesticides influence of several markers of individual susceptibility. Toxicol Lett. 159:13–21.
- Hernández AF, Parrón T, Tsatsakis AM, Requena M, Alarcón R, López-Guarnido O. 2013. Toxic effects of pesticide mixtures at a molecular level: Their relevance to human health. Toxicology. 307:136–145.
- Hwang ES, Bowen PE. 2005. Effects of lycopene and tomato paste extracts on DNA and lipid oxidation in LNCaP human prostate cancer cells. Biofactors. 23:97–105.
- Jonker D, Kuper CF, Frail N, Estrella A, Rodrigues OC. 2003. Ninety-day oral toxicity study of lycopene from Blakeslea trispora in rats. Regul Toxicol Phamacol. 37:396–406.
- Khrer JP. 1993. Free radicals as mediator of tissue injury and disease. Crit Rev Toxicol. 23:21–48.
- Konradsen F, Van der Hoek W, Cole DC, et al. 2003. Reducing acute poisonings in developing countries—options for restricting the availability of pesticides. Toxicology. 192:249–261.
- Köprücü SS, Yonar E, Seker E. 2008. Effects of deltamethrin on antioxidant status and oxidative stress biomarkers in freshwater mussel. Unio elongatulus eucirrus. Bull Environ Contam Toxicol. 81:253–257.
- Lebailly P, Vigreux C, Lechevrel C, Ledemeney D, Godard T, Sichel F, LeTalaer JY, Henry-Amar M, Gauduchon P. 1998. DNA damage in mononuclear leucocytes of farmers measured using the alkalinecomet assay: modifications of DNA damage levels after a one day field spraying period with selected pesticides. Cancer Epidemiol Biomark Prev. 7:929–940.
- Lee JC, Lee KY, Son YO, Choi KC, Kim J, Truong TT, Jang YS. 2005. Plant-originated glycoprotein, G-120, inhibits the growth of MCF-7 cells and induces their apoptosis. Food Chem Toxicol. 43(6):961–968.
- Marinovich M, Ghilardi R, Galli CL. 1996. Effects of pesticides mixtures on in vitro nervous cells: comparison with single pesticides. Toxicology. 108:201–206.
- Maroni M, Fanetti AC, Metruccio F. 2006. Risk assessment and management of occupational exposure to pesticides in agriculture. Med Lav. 97(2):430–437.
- Matos HR, Capelozzi VL, Gomes OF, Mascio PD, Medeiros MHG. 2001. Lycopene inhibits DNA damage and liver necrosis in rats treated with ferric nitrilotriacetate. Arch Biochem Biophys. 396:171–177.
- Matos HR, Marques SA, Gomes OF, Silva AA, Heimann JC, Di Mascio P, Medeiros MH. 2006. Lycopene and betacarotene protect in vivo iron induced oxidative stress damage in rat prostate. Brazil J Med Biol Res. 39:203–210.
- Mehl A, Rolseth V, Gordon S, Bjøraas M, Seeberg E, Fonnum F. 2000. Brain hypoplasia caused by exposure to trichlorfon and dichlorvos during development can be ascribed to DNA alkylation damage and inhibition of DNA alkyltransferase repair. Neurotoxicology. 1(1–2):165–173.
- Meister RT, editor. 1992. Farm chemicals handbook. Willoughby: Mister Pub. Co.
- Miadokova E, Vlckova V, Duhova V, Trebaticka M, Garajova L, Grolmus J, Podstavkova S, Vlcek D. 1992. Effects of supercypermethrin, a synthetic developmental pyrethroid, on four biological test systems. Mutat Res. 280:161–168.
- Michael RM, Bausch I. 2003. Summary of safety studies conducted with synthetic lycopene. Regul Toxicol Pharmacol. 37:274–285.
- Muller K, Carpenter KL, Challis IR, Skepper JN, Arends MJ. 2002. Carotenoids induce apoptosis in the T lymphoblast cell line Jurkat E6.1. Free Radical Res. 36:791–802.
- Nahum A, Zeller L, Danilenko M, Prall OW, Watts CK, Sutherland RL, Levy J, Sharoni Y. 2006. Lycopene inhibition of IGF induced cancer cell growth depends on the level of cyclin D1. Eur J Nutrit. 45:275–282.
- Nordberg J, Arner ES. 2001. Reactive oxygen species, antioxidants and mammalian thioredoxin system. Free Radic Biol Med. 31:1287–1312.
- Pastor S, Gutie´rrez S, Creus A, Cebulska-Wasilewska A, Marcos R. 2001. Micronuclei in peripheral blood lymphocytes and buccal epithelial cells of polish farmers exposed to pesticides. Mutat Res. 495:147–156.
- Piña-Guzmán B, Solis-Heredia MJ, Quintanilla-Vega B. 2005. Diazinon alters sperm chtomatin structure in mice by phosphorylating nuclear protamines. Toxicol Appl Pharmacol. 202(2):189–198.
- Pitarque M, Creus A, Marcos R, Hughes JA, Anderson B. 1999. Examination of various biomarkers measuring genotoxic endpoints from Barcelona airport personnel. Mutat Res. 440(2):195–204.
- Rai DK, Sharma B. 2007. Carbofuran-induced oxidative stress in mammalian brain. Mol Biotechnol. 37:66–71.
- Ranjbar A, Pasalar P, Abdollahi M. 2002. Induction of oxidative stress and acethycholiesterase inhibition in organophosphorus pesticide manufacturing workers. Hum Exp Toxicol. 21(4):179–182.
- Rao AV, Agarwal S. 1998. Bioavailability and in vivo antioxidant properties of lycopene from tomato products and their possible role in the prevention of cancer. Nutr Cancer. 31(3):199–203.
- Reddy L, Odhay B, Bhoola K. 2006. Aflatoxin B1 induced toxicity in HepG2 cells inhibited by carotenoids: morphology, apoptosis and DNA damage. Biol Chem. 387:87–93.
- Reifen R, Nissenkorn A, Matas Z, Bujanover Y. 2004. 5-ASA and lycopene decrease the oxidative stress and inflammation induced by iron in rats with colitis. J Gastroenterol. 39:514–519.
- Riso P, Pinder A, Santangelo A, Porini M. 1999. Does tomato consumption effectively increase the resistance of lymphocyte DNA to oxidative damage? Am J Clin Nutr. 69:712–718.
- Rupa DS, Reddy PP, Reddi OS. 1989. Chromosomal aberrations in peripheral lymphocytes of cotton field workers exposed to pesticides. Environ Res. 49:1–6.
- Safi JM. 2002. Association between chronic exposure topesticides and recorded cases of human malignancyin Gaza Governorates (1990–1999). Sci Total Environ. 284:75–84.
- Salvador M, Bordin DI, Andreazza AC, da Silva J, Henriques J, Erdtmann B. 2008. Determination of oxidative stress markers and serum cholinesterase among pesticide sprayers in southern Brazil. Toxicol Environ Chem. 4:809–814.
- Scolastici C, Alves de Lima RO, Barbisan LF, Ferreira AL, Ribeiro DA, Salvadori DMF. 2007. Lycopene activity against chemically induced DNA damage in Chinese hamster ovary cells. Toxicol in Vitro. 21:840–845.
- Scolastici C, Alves de Lima RO, Barbisan LF, Ferreira AL, Ribeiro DA, Salvadori DMF. 2008. Antigenotoxicity and antimutagenicity of lycopene in HepG2 cell line evaluated by the comet assay and micronucleus test. Toxicol in Vitro. 22:510–514.
- Shadnia S, Azizi E, Hosseini R, Khoei S, Fouladdel S, Pajoumand A, Jalali N, Abdollahi M. 2005. Evaluation of oxidative stres and genotoxicity in organophosphorus insecticide formulators. Human Exp Toxicol. 24:439–445.
- Shaham J, Kaufman Z, Gurvich R, Levi Z. 2001. Frequency of sister-chromatid exchange among greenhouse farmers exposed to pesticides. Mutat Res. 491:71–80.
- Singh NP, McCoy MT, Tice RR, Schneider EL. 1988. A simple technique for quantization of low levels of DNA damage in individual cells. Exp Cell Res. 175(1):184–191.
- Son YO, Kim J, Kim JC, Chung Y, Chung GH, Lee JC. 2003. Ripe fruits of Solanım nigrum L. inhibits cell growth and induces apoptosis in MCF-7 cells. Food Chem Toxicol. 41:1421–1428.
- Srinivasan M, Devipriya N, Kalpana KB, Menon VP. 2009. Lycopene: an antioxidant and radioprotector against γ-radiation-induced cellular damages in cultured human lymphocytes. Toxicology. 262:43–49.
- Srinivasan M, Sudheer AR, Pillai KR, Kumar PR, Sudhakaran PR, Menon VP. 2007. Lycopene as a natural protector against gamma-radiation induced DNA damage, lipid peroxidation, and antioxidant status in primary culture of isolated rat hepatocytes in vitro. Biochim Biophys Act. 1770:659–665.
- Stahl W, Sies H. 2003. Antioxidant activity of carotenoids. Mol Aspects Med. 24:345–351.
- Tang L, Jin T, Zeng X, Wang JS. 2005. Lycopene inhibits the growth of human androgen independent prostate cancer cells in vitro and in BALB/c nude mice. J Nutrit. 135:287–290.
- Tapiero H, Townsend DM, Tew KD. 2004. The role of carotenoids in the prevention of human pathologies. Biomed. Pharmacother. 58:100–110.
- Velmurugan B, Santhiya ST, Nagini S. 2004. Protective effect of S-allylcysteine and lycopene in combination against N-methyl N-nitro-N-nitrosoguanidine-induced genotoxicity. Polym J Pharmacol. 56:241–245.
- Weng Kong K, Rajab NF, Prasad KN, Ismail A, Markom M, Tan CP. 2010. Lycopene-rich fractions derived from pink guava by product and their potential activity towards hydrogen peroxide-induced cellular and DNA damage. Food Chem. 123:1142–1148.
- Wertz K, Siler U, Goralczyk R. 2004. Lycopene: modes of action to promote prostate health. Arch Biochem Biophys. 430:127–134.
- WHO. 1992. Our planet, our health: report of the WHO Commission on Health and Environment. Geneva: World Health Organization.
- WHO. 1999. Guidelines for poison control. Bulletin. Geneva World Health Organization.
- Yeh S, Hu M. 2000. Antioxidant and prooxidant effects of lycopene in comparison with betacarotene on oxidant induced damage in Hs68 cells. J Nutrit Biochem. 11:548–554.