Abstract
The use of pesticides may affect the aquatic ecosystem. Through soil, these contaminants are drained into rivers. In South America, glyphosate-base herbicides, such as Roundup, are widely used in agricultural activities. In this work, the genotoxic effects of Roundup Full II® were evaluated in blood, gill and liver cells of Piaractus mesopotamicus, species of great ecological and commercial value in South America. Previously, the lethal concentration 50 (LC50) for 96 h of exposure was determined, with a theoretical value of 8.92 mg l–1. Genotoxic analysis in cells of the three tissues was performed by the comet, micronuclei (MN) and nuclear abnormalities (NA) assays after 96 h exposing fish to a sub-lethal concentration of Roundup Full II® (2.75 mg l–1). Comet assay results for the three tissues showed a significantly greater damage compared to the negative control and no statistical differences were observed between tissues responses. For MN and NA significantly higher frequencies were also demonstrated, indicating diverse kinds of damage like clastogenic events in the three tissues, with gills being the most sensitive one.
1. Introduction
Pesticides are widely used throughout the world and have been recognized as a part of agricultural practice (Citation2010). Large amounts of these products are continuously being introduced into the environment and for aquatic ecosystems, herbicides are the most dangerous ones because they reach the water bodies through soil surface run-off (Çavas and Könen Citation2007; Cavalcante et al. Citation2008; Guilherme et al. Citation2010). Among herbicides, glyphosate-based formulations are the most used worldwide, particularly in South America (Guilherme et al. Citation2010; Vera-Candioti et al. Citation2013). Due to their higher solubility the exposure of non-target aquatic organisms to this kind of chemical is an ecological concern (Tsui and Chu Citation2003; Cavalcante et al. Citation2008).
In the commercial glyphosate-based formulation Roundup®, glyphosate is generally presented as an isopropylamine salt and a surfactant (polyethoxylene amine) is added to enhance the effectiveness of the herbicide (Tsui and Chu Citation2003). Even if the active ingredient (glyphosate) is considered slightly toxic in animals, this formulation exhibits a relatively higher toxicity and genotoxic effects compared to glyphosate alone (Bolognesi et al. Citation1997; Mann and Bidwell Citation1999; Guilherme et al. Citation2012a). Several authors have demonstrated the potential genotoxicity of Roundup® formulas and other glyphosate-based herbicides in diverse biological models such as mammals, fishes and reptiles, both in vivo and in vitro (Bolognesi et al. Citation1997; Ayoola Citation2008; Cavalcante et al. Citation2008; Poletta et al. Citation2009; Ghisi and Cestari Citation2013; Vera-Candioti et al. Citation2013).
In order to evaluate the effects of these herbicides on aquatic environments, fish are often used as sentinels, mainly due to their bioaccumulation potential and sensitivity to these chemicals (Al-Sabti and Metcalfe Citation1995; Ali et al. Citation2008). Even though glyphosate-based herbicides are widely used in South America, little information is available about the toxicity and genotoxic effects to native freshwater fishes. Piaractus mesopotamicus is a native fish distributed in the rivers Paraná, Uruguay, La Plata and Paraguay. For the region, this species not only has ecological value, it also represents an important economic source. Recently, it has been shown that the commercial herbicide Roundup Ready® caused severe tissue damage in P. mesopotamicus that eventually led to the death of these fish (Ringuelet et al. Citation1997; Shiogiri et al. Citation2012).
Assessment of DNA integrity is an issue of concern because DNA alterations are early-warning signals for long-term effects such as carcinogenesis (Van der Oost et al. Van der Oost et al. Citation2003; Bolognesi and Hayashi Citation2011). Micronucleus test and comet assay are widely applied techniques in the genotoxic field because they are fast and sensitive for evaluating genetic damage (Ali et al. Citation2008). Moreover, nuclear abnormalities are considered indicators of cyto and genotoxicity and therefore are often used as a complementary tool for the micronucleus test (Mallick and Khuda-Bukhsh Citation2003; Seriani et al. Citation2011).
In genotoxic studies with fish, a widely used tissue is peripheral blood mainly due to its easy sampling and usage in different protocols (Cavalcante et al. Citation2008; Ghisi and Cestari Citation2013). However, it has been suggested that other cell types such as hepatocytes and gill cells could provide a better understanding of the global effect of genotoxic agents since they are the main target organs for foreign molecules in fish (Shiogiri et al. Citation2012).
The aim of this study was to describe the toxicity of the herbicide Roundup Full II® on the freshwater fish P. mesopotamicus after 96 h of exposure through the estimation of the median lethal concentration and evaluate the genotoxic response in peripheral blood, gill and liver cells after an acute exposure (96 h) to a sub-lethal concentration using comet assay, micronucleus and nuclear abnormalities.
2. Materials and methods
2.1. Chemicals
Commercial glyphosate- based formulation Roundup Full II® (66.2% glyphosate potassium salt; CAS no. 70901-12-1) was purchased from an agricultural supplies store. Ethylmethanesulfonate (CAS no. 62-50-0), a direct alkylating agent was used as a positive control and was purchased from Sigma.
2.2. Animals
Juveniles of P. mesopotamicus (average length 11.42 ± 1.09 cm; average width 5.85 ± 0.50 cm) were obtained from the Estación Hidrobiológica y Piscicultura located in Candelaria, a private hatchery in Campo Viera, and from the Cooperativa Alto Uruguay Limitada (CAUL), located in 25 de Mayo, Misiones. Fishes were acclimated to the laboratory for at least 15 days and kept in 75 l aquaria under a natural photoperiod in aerated, dechlorinated; pH ~7 and temperature 25 ± 2 °C water conditions. During this period, the specimens were fed three times a week with commercial pellet food.
2.3. Determination of median lethal concentration (LC50)
The acute toxicity assay to determine the LC50 96 h value was conducted according to the OECD guideline (Citation1997). A total of 42 fish was exposed to seven herbicide concentrations (2.75, 4.50, 6.25, 8.00, 9.75, 11.50, 13.25 mg l–1). All treatments were performed in duplicate with groups of three specimens per concentration in a static system in glass aquarium containing 25 l of aerated and dechlorinated water. Six fish were used in each concentration. Fish were not fed throughout treatments and were visually examined daily, every 3 h the first day and every 8 h the next 72 h, in order to count the number of dead fish as the toxicity endpoint and record any behavioural change related to the exposure to the different Roundup Full II® concentrations. A fish was considered dead when no respiratory movements were observed or there was a lack of sudden swimming in response to gentle knocking the glass.
2.4. Genotoxicity tests
Based on the value of LC50 96 h, 14 specimens of P. mesopotamicus were exposed for 96 h in a static system to the sub-lethal concentration 2.75 mg l–1 (RU) with the aim of assessing genotoxic effects in the blood, gill and liver cells. This concentration was chosen due to no mortality was observed along the LC50 test. The quantification of glyphosate in test solutions was performed by QV Chem Laboratory (La Plata, Argentina) according to EPA Analytical Method 547. Glyphosate levels were measured by high performance liquid chromatography (HPLC) using a fluorometer and the derivatization was achieved with o-phthalaldehyde-2-mercaptoethanol complex. The detection limit for glyphosate was 0.005 mg l–1. Glyphosate determinations correspond to test solutions taken at the initial time and 96 h after treatment. Simultaneously, two control groups were performed: for a negative control (NC) 12 specimens were exposed to the same conditions of detoxification and a positive control (PC) was performed with 11 specimens exposed to a solution of 15 mg l–1 of ethylmethanesulphonate (EMS) for 96 h. At the end of the exposure period, fish were removed from the aquaria and peripheral blood was immediately collected from the caudal vein using heparinized plastic syringes. Subsequently, the animals were killed by spinal puncture for removing the gill arches and liver. Upon dissection, gills and liver were washed with phosphate-buffered saline (PBS) in order to remove the surrounding blood. Subsequently, the material was separated and prepared to perform the comet and micronucleus assays. All preparations were coded and evaluated blindly.
These procedures were according to usual protocols stated in the American Veterinary Medical Association (AVMA) guidelines for the Euthanasia of Animals (Citation2013) and the OECD guidance document on the recognition, assessment, and use of clinical signs as humane endpoints for experimental animals used in safety evaluation (Citation2000).
2.4.1. Micronucleus (MN) and nuclear abnormalities (NA) test
The MN was performed according to Barsiene et al. (Citation2006) for the blood samples. The corresponding smears were made and allowed to dry overnight. The preparation of the gill and liver slides was conducted according to Cavas et al. (Citation2005) with slight modifications: Tissues were fixed for 15 min in Farmer (3:1 methanol: acetic acid) and subsequently gills and liver were treated for 15 min with a solution of acetic acid at 20% and 45% respectively, for their maceration. Then, tissue pieces were disaggregated on slides using tweezers. All preparations were performed in duplicate and fixed in methanol for 15 min. After drying, blood and gills samples were stained with 10% Giemsa for 10 min and the liver slides were stained with 5% Giemsa during 8 min. For each tissue, 2000 cells per individual were analysed by light microscopy. The micronuclei identification criterion was adopted from CitationFenech et al. (2003) and Barsiene et al. (Citation2006). Nuclear abnormalities were scored into one of the following categories: notched nuclei (NOT), blebbed (BL), lobed (LOB), binucleated cells (BI), eight-shaped nuclei (ES), nuclear buds (BUD), and chromatin bridges (BR). This classification criterion was according to Carrasco et al. (Citation1990), Venier et al. (Citation1997) and Fenech et al. (Citation2003).
2.4.2. Comet assay
Before the beginning of the comet assay, the cell viability for erythrocytes was determined by the trypan blue exclusion assay. For each specimen, a total of 100 cells was counted using a Neubauer chamber and the viability was expressed as the percentage of viable cells in the total number of cells. In the case of gill and liver cells, the cell viability was determined by counting fragmented and vacuolated nuclei, and a total of 2000 cells per sample was counted. In order to run the comet assay, at least 80% of cells should be viable.
The comet assay was performed on the three tissues according to Speit and Hartmann (Citation1999) based on the original work of Singh et al. (Citation1998) with modifications: to obtain cell suspensions, the solid tissues were disaggregated in physiological solution and gently scraped with tweezers. For peripheral blood, 10 μl of sample were incubated in 1000 μl of PBS. The cell suspensions of solid tissues were centrifuged at 1500 rpm for 5 min. Then, 15 μl of these suspensions and 10 μl in the case of blood were mixed with 120 μl of low melting point agarose (0.5%) and layered on slides precoated with normal melting point agarose (1.5%). Slides were immediately covered with coverslips and kept for 30 min in a refrigerator to solidify. After gently removing the coverslips, the slides were immersed in a cold lysing solution (2.5 M NaCl, 100 mM EDTA, 10 mM TRIS, 10% DMSO, 1 ml Triton X-100, pH 10) for 1 h. Then, the slides were incubated in alkaline buffer (300 mM NaOH, 200 mM EDTA) for 20 min and electrophoresed at 300 mA and 25 V during 20 min. The slides were then covered with neutralization buffer (Tris 24.25 g in 475 ml distilled water, pH 7.5) for 5 min three times. Slides were stained with ethidium bromide and were analysed using an epifluorescence microscope at 400× magnification. For each individual, 100 nucleoids per tissue were scored and visually classified into five classes according to fluorescence intensity and tail size: class 0 (undamaged); class1 (minimal damage); class 2 (moderate damage); class 3 (high damage) and class 4 (maximal damage). With this data, the DNA damage index score was calculated according to the formula: DI = (A×0) + (B×1) + (C×2) + (D×3) + (E×4), where A, B, C and D are the number of cells in the corresponding comet classes (0 to 4).
2.5. Statistical analyses
All statistical analyses were performed using the software Infostat version 2013 (Di Rienzo et al. Citation2013). Data from LC50 test was analysed with Probit regression. The MN and NA frequencies and the DI obtained by comet assay, were tested for normality by the Shapiro–Wilks test. The non-parametric Wilcoxon (Mann–Whitney U) test was used in order to detect differences between RU treatment and controls (NC, PC). In addition, the Kruskal–Wallis test was performed to statistically compare the tissues responses to the treatment.
3. Results
3.1. Determination of LC50
The LC50 96 h for commercial glyphosate formulation (Roundup Full II®) to P. mesopotamicus was estimated by Probit analysis as 8.92 mg l–1 (range, 6.74–11.10 mg l–1). The progression of fish mortality is shown in Figure . Along Roundup exposure, P. mesopotamicus specimens showed several behavioural changes. First, they presented an occasional lack of their balance, but over the time, such condition was more frequent and showed a weak reaction in response to gentle knocking the glass. In addition, they secreted copious amounts of mucus from whole body which caused turbidity in the water. Finally, they barely moved to the water surface for breathing, then they remind in vertical position and the fish died. Those changes were recorded for all concentrations in which mortality was observed (4.50, 6.25, 8.00, 9.75, 11.50 and 13.25 mg l–1), and became evident at least 12 h before the fish died. Furthermore, at highest concentrations (11.50 and 13.25 mg l–1) fish presented lesions in fins and gills (14% of total exposed fish).
3.2. Genotoxicity tests
The sub-lethal concentration 2.75 mg l–1 was adopted for acute toxicity tests (96 h). Chemical analyses showed that the glyphosate concentration in water was 2.67 mg l–1 at the beginning of toxicity test and 2.48 mg l–1 after 96 h.
Peripheral blood, liver and gill cells showed a significant increase in MN and NA frequencies after 96 h of exposure to RU in relation to the NC (Figure ). For the three tissues, the most common NA after the RU treatment was not followed by BL and LOB. Moreover, other less frequent alterations such as BUD, ES, BI and BR were observed. The comparison between tissues responses exhibited a significant difference of MN and NA frequencies in gill cells respect to erythrocytes and hepatocytes exposed to RU (p < 0.05).
Figure 2. Mean frequencies (± standard deviation) of MN (A) and NA (B) observed in erythrocytes, gill cells and hepatocytes of P. mesopotamicus after 96 h of exposure to Roundup Full II® (RU) with their respective positive (PC) and negative (NC) control groups. aSignificant difference from NC (p < 0. 05). bSignificant differences between tissues responses (p < 0. 05).
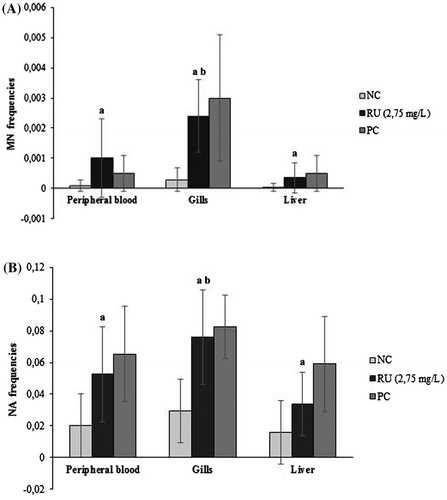
The results of the comet assay revealed a significantly higher DNA damage index in erythrocytes, hepatocytes and gill cells exposed to RU in relation to the NC, with comet class 1 (minimal damage) and 2 (moderated damage) being the most frequent (Table ). When tissue responses to treatment were compared, no significant differences between their DI were observed.
Table 1. Mean frequencies of each DNA damage class and DNA damage index (± standard deviation) observed in erythrocytes, gill cells and hepatocytes of P. mesopotamicus after 96 h of exposure to Roundup Full II® (RU) with their respective positive (PC) and negative (NC) control groups.
4. Discussion
Since the aquatic environment receives an increasing number of contaminants by industrial, agricultural and urban wastes that could compromise the integrity of the ecosystem and human health, the pollution of water bodies is a worldwide concern (Nwani et al. Citation2010; Bolognesi and Hayashi Citation2011). The development of several toxicity tests, such as determination of LC50 and the application of genotoxicity biomarkers, allows the establishment of permissible limits for chemical use and the evaluation of the impact of pollutant mixtures on aquatic biota (Albinati et al. Citation2007; Bolognesi and Hayashi Citation2011). In the present study, the median lethal concentration after 96 h of exposure to Roundup Full II® for P. mesopotamicus was 8.92 mg l–1. This LC50 value is considered to be of moderate toxicity to aquatic life (LC50 value between 1 and 10 mg l–1) according to the Globally Harmonized System of Classification and Labelling of Chemicals (USEPA Citation2004) and shows the capability of the species to tolerate concentrations more than 1000-fold greater than the maximum concentration measured in some surface waters in the USA (2.2 μg l–1) (Kolpin et al. Citation2006). However, in developing countries such values could be higher due to intense pesticide applications. In Brazilian waters, for example, were found glyphosate concentrations between 0.36 and 2.16 mg l–1 (Nwani et al. Citation2013). Therefore, the sub-lethal concentration tested in this work (2.75 mg l–1) is close to regional values.
The LC50 96 h obtained for P. mesopotamicus in the current study is higher than the LC50 48 h reported by Shiogiri et al. (Citation2012) for this species of fish exposed to Roundup Ready® (LC50 48 h=3.74 mg l–1). Hence, considering this LC50 parameter, P. mesopotamicus seems to exhibit diverse ranges of susceptibility for the different glyphosate-based formulas. Even though Roundup Ready® has a lower proportion of glyphosate (48%) it seems to be more toxic than Roundup Full II® (66.2% glyphosate) (Shiogiri et al. Citation2012). This could be explained by the fact that both commercial herbicides present a different glyphosate chemical formulation and include one or more adjuvants that might contribute to the overall toxicity (Guilherme et al. Citation2012a). The polyethoxylene amine (POEA) is the main surfactant in most Roundup® formulations and it has been demonstrated that it is more toxic to fish than pure glyphosate (Guilherme et al. Citation2012a).
The behavioural changes observed in P. mesopotamicus, like breathing in the water surface, abnormal swimming, decreased responsiveness, intense mucus secretion and loss of balance are similar to the intoxication symptoms reported by several authors in various fish species exposed to glyphosate and Atrazine (Ayoola Citation2008; Nwani et al. Citation2010; Shiogiri et al. Citation2012). At the highest concentrations tested, some fish presented lesions in fins and gills that sometimes spread along the body, and finally the animal died. It has been demonstrated that xenobiotics, like organophosphorus compounds, affect the immune system and eventually could compromise fish health, promoting the development of infectious diseases (El-Gendy et al. Citation1998; Rondón-Barragán et al. Citation2010). For example, a large amount of skin mucus might increase the risk of bacterial infections like Vibrio strains (Bols et al. Citation2001). Moreover, histopathological lesions in the gills of P. brachypomus and P. mesopotamicus were observed after an acute exposure to Roundup® formulas and those injuries could have affected vital functions such as breathing, osmoregulation and acid-base balance (Ramírez-Duarte et al. Citation2008; Shiogiri et al. Citation2012).
Due to their high reactivity, genotoxic compounds may contribute to other toxic responses leading to irreversible effects on the organisms (Mitchelmore and Chipman Citation1998). The MN and NA test is one of the most frequently used methods for the evaluation of genotoxicity in aquatic biota (Ergene et al. Citation2007; Vera-Candioti et al. Citation2013) because their presence is related to DNA damage and chromosome instability events (Fenech et al. Citation2011). Several authors have demonstrated that pure glyphosate and their commercial formulations induce MN formation in vivo and in vitro depending upon the endpoint, the cellular target, the compound and concentrations to test (Bolognesi et al. Citation1997; Çavas and Könen Citation2007; Cavalcante et al. Citation2008; Ghisi and Cestari Citation2013; Vera-Candioti et al. Citation2013; Ghisi et al. Citation2016). Indeed, our results have shown that Roundup Full II® induces an increase in MN and NA frequencies in erythrocytes, hepatocytes and gill cells of P. mesopotamicus after 96 h of exposure. For this biomarker, the gill cells seem to be the most sensitive tissue tested. Furthermore, the diversity observed in micronuclei sizes, mainly in erythrocytes, suggest losses of chromosomal fragments and complete chromosomes, showing that Roundup Full II® might induce clastogenic and aneugenic events (Ergene et al. Citation2007). Notably, tissues with higher MN frequencies presented the highest values of NA after RU exposure. Although the origin of the NA has not been clearly explained, it has been suggested that their presence in nuclei can be a primary response, prior to the MN formation, and many of them are considered indicators of cytotoxic and genotoxic effects (Bolognesi and Hayashi Citation2011; Seriani et al. Citation2011). Hence, the identification of nuclear abnormalities may be a suitable complement to the scoring of MN in genotoxicity assessment.
In organisms exposed to contaminants, the impact of chemicals on the integrity of cellular DNA is a primary event and can be efficiently evidenced through the comet assay (Mitchelmore and Chipman Citation1998; Frenzilli et al. Citation2009). In the present study, the level of DNA damage was significantly higher with respect to NC in all the tissues after RU treatment. This response was also observed by several authors in erythrocytes, hepatocytes and gill cells of another fish species exposed to Roundup® (Çavas and Könen Citation2007; Cavalcante et al. Citation2008; Guilherme et al. Citation2010; Guilherme et al. Citation2012b; Ghisi and Cestari Citation2013). In the current study, no differences between tissue responses were observed. A similar situation was reported by Ghisi and Cestari (Citation2013) for erythrocytes and hepatocytes of Corydoras paleatus after a short-term exposure to a low concentration of Roundup®.
Many xenobiotics promote oxidative DNA damage as a secondary effect that may produce reactive oxygen species through redox cycling. Such damage is related to the antioxidant status of the organism and DNA repair capacity (Mitchelmore and Chipman Citation1998). Roundup® is no exception, because the ability of these formulations to induce oxidative stress and suppress antioxidant defences in several tissues of different fish species was demonstrated (Lushchak et al. Citation2009; Modesto and Martinez Citation2010; Nwani et al. Citation2013). The specificity in toxic responses of an organ is generally related to their defensive capacity and their anatomic location, which defines the exposure route and distribution of pollutants. Gills possess a high mitotic index and it was suggested to be more susceptible to diverse kinds of damage than other organs due to the fact that are directly in contact with xenobiotics (Cavas et al. Citation2005; Santos et al. Citation2006; Cavalcante et al. Citation2008). Nwani et al. (Citation2013) reported that sub-lethal exposure to Roundup® induced genotoxic damage and stimulated oxidative stress that leads to elevated lipid peroxidation and suppressed antioxidant enzymes in the blood and gills of Channa punctatus, with the gills being the most affected tissue. In the current study, the highest MN and NA frequencies observed in gills could be on one hand related to their biological and physiological properties, and on the other, the consequences of the weak antioxidant defence mechanism that culminates in irreversible lesions in the DNA. For peripheral blood, intermediate values were recorded that can be attributed to the continuous replacement of the erythrocytes (Çavas and Könen Citation2007). But it also could indicate their adaptation to the exposure conditions by the mechanisms of detoxification and DNA repair as it was suggested by Ghisi et al. (Citation2016) in their meta-analysis after the finding of a negative correlation between glyphosate exposure time and MN formation in mature and immature erythrocytes. In contrast to the gills and blood, the liver showed the lowest micronucleus frequency. Despite the fact that the current results could be related partly as an outcome of the low hepatic mitotic index, the biotransformation activity is intense and the antioxidant response could be stronger and more efficient than in gills and blood. It has been demonstrated that Roundup® stimulates the biotransformation process, the metabolic activity and the antioxidant defences in the liver of Prochilodus lineatus after an acute exposure (Modesto and Martinez Citation2010).
Paradoxically, for the comet assay, all tissues appear to have a similar response after the treatment with Roundup Full II® and the damage observed could be classified between minimal and moderate. When such behaviour is compared with the tissues responses for the MN and NA test, it should be noted that these assays detect different aspects of genotoxicity because the micronucleus test detects lesions that survive at least one mitotic cycle, while the comet assay identifies repairable DNA lesions or alkali labile sites (Ali et al. Citation2008; Frenzilli et al. Citation2009). Therefore, the amount of repairable and fixed DNA damage detected in each tissue could be related to their biological properties and the antioxidant status. In this aspect, gills are more vulnerable and may respond earlier to pollutants (Santos et al. Citation2006). Hence, the repair mechanisms will quickly wear out and the fixed damage will be greater than the repaired DNA, while the liver will have a more efficient antioxidant response than the other two tissues due to its detoxifying function and therefore, fixed damage will be considerably lower than the repaired.
In conclusion, the glyphosate based herbicide Roundup Full II® has toxic and genotoxic effects on P. mesopotamicus. The LC50 96 h value obtained in the current study indicates the existence of a moderate toxicity and the genotoxicity evaluation showed an increased DI for the comet assay and high frequencies of MN and NA, with gills being the most sensitive tissue with the statistically highest MN and NA frequencies. These results reveal the presence of diverse kinds of DNA damage, like clastogenic events. The differences in the biological and physiological properties as well as antioxidant capacity may influence the amount of reversible and irreversible DNA damage found in the three tissues analysed. Moreover, both biomarkers proved to be important tools for genotoxicity assessment and their application in several tissues gives a more comprehensive view of the impact of xenobiotic agents in an organism.
Disclosure statement
The authors declare that there are no conflicts of interest.
Funding
This study was supported by the Programa de Incentivos of Facultad de Ciencias Exactas, Químicas y Naturales (FCEQyN – UNaM, Posadas, Misiones, Argentina) and the Comité Ejecutivo de Desarrollo e Innovación Tecnológica (Cedit, Posadas, Misiones, Argentina).
Acknowledgements
The authors are grateful to Mr Mario Ledesma and Dr Miguel Rhinas from the Estación Hidrobiológica y de Piscicultura and to the Cooperativa Alto Uruguay Limitada for the generous help with P. mesopotamicus specimens. We also thank Dr Alberto Fenocchio from Laboratorio de Citogenética General y Monitoreo Ambiental (FCEQyN) for the assistance.
References
- Albinati ACL, Moreira ELT, Albinati RCB, Carvalho JV, Santos GB, Lira AD. 2007. Acute toxicity of the herbicide Roundup® for piauçu (Leporinus macrocephalus). Revista Brasileira e Saúde y Produção Animal. 8(3):184–192.
- Ali D, Nagpure NS, Kumar S, Kumar R, Kushwaha B. 2008. Genotoxicity assessment of acute exposure of chlorpyrifos to freshwater fish Channa punctatus (Bloch) using micronucleus assay and alkaline single-cell gel electrophoresis. Chemosphere. 71(10):1823–1831. 10.1016/j.chemosphere.2008.02.007
- Ali FK, El- Shewawi AM, Seehy MA. 2008. Micronucleus test in fish genome: a sensitive monitor for aquatic pollution. Afr J Biotechnol. 7(5):606–612.
- Al-Sabti K, Metcalfe CHD. 1995. Fish micronuclei for assessing genotoxicity in water. Mutat Res/Genet Tox. 343(2):121–135. 10.1016/0165-1218(95)90078-0
- American Veterinary Medical Association (AVMA). 2013. AVMA guidelines for the Euthanasia of animals: 2013 edition. Schaumburg, Il: AVMA; p. 70.
- Ayoola SO. 2008. Histopathological effects of glyphosate on Juvenile African Catfish (Clarias gariepinus). Am Eurasian J Agric Environ Sci. 4(3):362–367.
- Baršienė J, Dedonytė V, Rybakovas A, Andreikėnaitė L, Andersen O. 2006. Investigation of micronuclei and other nuclear abnormalities in peripheral blood and kidney of marine fish treated with crude oil. Aquat Toxicol. 78:S99–S104. 10.1016/j.aquatox.2006.02.022
- Bolognesi C, Hayashi M. 2011. Micronucleus assay in aquatic animals (Review). Mutagenesis. 26(1):205–213. 10.1093/mutage/geq073
- Bolognesi C, Bonatti S, Degan P, Gallerani E, Peluso M, Rabboni R, Roggieri P, Abbondandolo A. 1997. Genotoxic activity of glyphosate and its technical formulation roundup. J Agric Food Chem. 45(5):1957–1962. 10.1021/jf9606518
- Bols NC, Brubacher JL, Ganassin RC, Lee LEJ. 2001. Ecotoxicology and innate immunity in fish. Dev Comp Immunol. 25(8):853–873. 10.1016/S0145-305X(01)00040-4
- Carrasco K, Tilbury K, Myers M. 1990. Assessment of the Piscine Micronucleus Test as an in-situ biological indicator of chemical contaminant effect. Can J Fish Aquat Sci. 47(11):2123–2136. 10.1139/f90-237
- de Castilhos Ghisi NC, Cestari MM. 2013. Genotoxic effects of the herbicide Roundup® in the fish Corydoras paleatus (Jenyns 1842) after short-term, environmentally low concentration exposure. Environ Monit Assess. 185(4):3201–3207.10.1007/s10661-012-2783-x
- Cavalcante DGSM, Martinez CBR, Sofia SH. 2008. Genotoxic effects of Roundup® on the fish Prochilodus lineatus. Mutat Res/Gen Tox En. 655(1):41–46.10.1016/j.mrgentox.2008.06.010
- Çavas T, Könen S. 2007. Detection of cytogenetic and DNA damage in peripheral erythrocytes of goldfish (Carassius auratus) exposed to a glyphosate formulation using the micronucleus test and the comet assay. Mutagenesis. 22(4):263–268.
- Cavas T, Garanko NN, Arkhipchuk V. 2005. Induction of micronuclei and binuclei in blood, gill and liver cells of fishes subchronically exposed to cadmium chloride and cooper sulphate. Food Chem Toxicol. 43(4):569–574. 10.1016/j.fct.2004.12.014
- Di Rienzo JA, Casanoves F, Balzarini MG, Gonzalez L, Tablada M, Robledo CW InfoStat. (versión 2013). Grupo InfoStat, FCA, Universidad Nacional de Córdoba, Argentina. [cited 2014 Sep 15]. Available from: http://www.infostat.com.ar.
- El-Gendy ΚS, Aly NM, El-Sebae AH. 1998. Effects of edifenphos and glyphosate on the immune response and protein biosynthesis of bolti fish (Tilapia nilotica). J Environ Sci Health. 33(2):135–149. 10.1080/03601239809373135
- Ergene S, Çavaş T, Çelik A, Köleli N, Kaya F, Karahan A. 2007. Monitoring of nuclear abnormalities in peripheral erythrocytes of three fish species from the Goksu Delta (Turkey): genotoxic damage in relation to water pollution. Ecotoxicology. 16(4):385–391.10.1007/s10646-007-0142-4
- Fenech M, Chang WP, Kirsch-Volders M, Holland N, Bonassi S, Zeiger E. 2003. HUMN project: detailed description of the scoring criteria for the cytokinesis-block micronucleus assay using isolated human lymphocyte cultures. Mutat Res/Gen Tox En. 534(1):65–75. 10.1016/S1383-5718(02)00249-8
- Fenech M, Kirsch-Volders M, Natarajan AT, Surralles J, Crott JW, Parry J, Norppa H, Eastmond DA, Tuckerand JD, Thomas P. 2011. Molecular mechanisms of micronucleus, nucleoplasmic bridge and nuclear bud formation in mammalian and human cell (Review). Mutagenesis. 26(1):125–132. 10.1093/mutage/geq052
- Frenzilli G, Nigro M, Lyons BP. 2009. The comet assay for the evaluation of genotoxic impact in aquatic environments (Review). Mutat Res/Rev Mutat. 681(1):80–92. 10.1016/j.mrrev.2008.03.001
- Ghisi NC, Oliveira EC, Prioli AJ. 2016. Does exposure to glyphosate lead to an increase in the micronuclei frequency? A systematic and meta-analytic review. Chemosphere. 145:42–54. 10.1016/j.chemosphere.2015.11.044
- Guilherme S, Gaivao I, Santos MA, Pacheco M. 2010. European eel (Anguilla anguilla) genotoxic and pro- oxidant responses following short-term exposure to Roundup®, a glyphosate-based herbicide. Mutagénesis. 25(5):523–530. 10.1093/mutage/geq038
- Guilherme S, Gaivão I, Santos MA, Pacheco M. 2012. DNA damage in fish (Anguilla anguilla) exposed to a glyphosate-based herbicide – Elucidation of organ-specificity and the role of oxidative stress. Mutat Res/Gen Tox En. 743(1):1–9. 10.1016/j.mrgentox.2011.10.017
- Guilherme S, Santos MA, Barroso C, Gaivão I, Pacheco M. 2012. Differential genotoxiciy of Roundup® formulation and its constituents in blood cells of fish (Anguilla anguilla): considerations on chemical interactions and DNA damaging mechanisms. Ecotoxicology. 21(5):1381–1390. 10.1007/s10646-012-0892-5
- Kolpin DW, Thurman EM, Lee EA, Meyer MT, Furlong ET, Glassmeyer ST. 2006. Urban contributions of glyphosate and its degradate AMPA to streams in the United States. Sci Total Environ. 354(2):191–197. 10.1016/j.scitotenv.2005.01.028
- Lushchak OV, Kubrak OI, Storey JM, Storey KB, Lushchak VI. 2009. Low toxic herbicide Roundup® induces mild oxidative stress in goldfish tissues. Chemosphere. 76(7):932–937. 10.1016/j.chemosphere.2009.04.045
- Mallick P, Khuda-Bukhsh AR. 2003. Nuclear anomalies and blood protein variations in fish of the Hooghly- Matlah River System, India, as an indicator of genotoxicity in water. Bull. Environ. Contam and Toxicol. 70(6):1071–1082. 10.1007/s00128-003-0092-3
- Mann RM, Bidwell JR. 1999. The toxicity of glyphosate and several glyphosate formulations to four species of South Western Australian frogs. Arch Environ Contam Toxicol. 36(2):196–199.
- Mitchelmore CL, Chipman JK. 1998. DNA strand breakage in aquatic organisms and the potential value of the comet assay in environmental monitoring. Mutat Res/Fund Mol M. 399(2):135–147. 10.1016/S0027-5107(97)00252-2
- Modesto KA, Martinez CBR. 2010. Roundup causes oxidative stress in liver and inhibits acetylcholinesterase in muscle and brain of the fish Prochilodus lineatus. Chemosphere. 78(3):294–299. 10.1016/j.chemosphere.2009.10.047
- Nwani CD, Nagpure NS, Kumar R, Kushwaha B, Kumar P, Lakra WS. 2010. Lethal concentration and toxicity stress of Carbosulfan, Glyposate and Atrazine to freshwater air breathing fish Channa punctatus (Bloch). Int Aquat Res. 2(2):105–111.
- Nwani CD, Nagpure NS, Kumar R, Kushwaha B, Lakra WS. 2013. DNA damage and oxidative stress modulatory effects of glyphosate-based herbicide in freshwater fish. Channa punctatus Environ Toxicol Pharmacol. 36(2):539–547. 10.1016/j.etap.2013.06.001
- OECD. 1997. Guideline for testing of chemicals n° 203: Fish Acute Toxicity Test. [cited 2013 Jun 10]. Available from: http://www.oecd.org/.
- OECD. 2000. Guidance document on the recognition, assessment and use of clinical signs as humane endpoints for experimental animals used in safety evaluations. [cited 2013 Jun 10]. Available from: http://www.oecd.org/.
- van der Oost R, Beyer J, Vermeulen NPE. 2003. Fish bioaccumulation and biomarkers in environmental risk assessment: a review. Environ Toxicol Pharmacol. 13(2):57–149. 10.1016/S1382-6689(02)00126-6
- Poletta GL, Larriera A, Kleinsorge E, Mudry MD. 2009. Genotoxicity of the herbicide formulation Roundup® (glyphosate) in broad- snouted caiman (Caiman latirostris) evidenced by the Comet assay and the Micronucleus test. Mutat Res/Gen Tox En. 672(2):95–102.10.1016/j.mrgentox.2008.10.007
- Ramírez-Duarte WF, Rondón-Barragán IS, Eslava-Mocha PR. 2008. Acute toxicity and histopathological alterations of Roundup® herbicide on “cachama blanca” (Piaractus brachypomus). Pesqui Vet Bras. 28(11):547–554.
- Ringuelet RA, Aramburu RM, Alonso de Aramburu A 1997. Los peces argentinos de agua dulce. Comisión de Investigación Científica, La Plata: Gobernación de la Provincia de Buenos Aires; p. 240–241.
- Rondón-Barragán IS, Pardo-Hernández D, Eslava-Mocha PR. 2010. Effects of herbicides on immune system: an approach to fish. Revista Complutense de Ciencias Veterinarias. 4(1):1–22.
- Santos MA, Pacheco M, Ahmad I. 2006. Responses of European eel (Anguilla anguilla L.) circulating phagocytes to an in situ closed pulp mill effluent exposure and its association with organ-specific peroxidative damage. Chemosphere. 63(5):794–801. 10.1016/j.chemosphere.2005.07.077
- Seriani R, Tavares Ranzani-Paiva M J., Silva- Souza A. T., Silva Roseli N. 2011. Hematology, micronuclei and nuclear abnormalities in fishes from São Francisco River, Minas Gerais state, Brazil. Acta Sci Biol Sci. 33(1):107–112.
- Shiogiri NS, Paulino MG, Carraschi SP, Baraldi FG, da Cruz C, Fernandes Marisa Narciso. 2012. Acute exposure of a glyphosate-based herbicide affects the gills and liver of the Neotropical fish, Piaractus mesopotamicus. Environ Toxicol Pharmacol. 34(2):388–396. 10.1016/j.etap.2012.05.007
- Singh NP, Mc Coy MT, Tice RR, Schneider EL. 1998. A single technique for quantification of low levels of DNA damage in individual cells. Exp Cell Res. 175(1):184–191.
- Speit G, Hartmann A. 1999. The comet assay (single-cell gel test): a sensitive genotoxicity test for the detection of DNA damage and repair. In: Henderson DS, editor. Methods in molecular Biology 113, DNA Repair protocols: eukaryotic Systems. Totowa, N. Y: Human Press Inc; p. 203–212.
- Tsui MTK, Chu LM. 2003. Aquatic toxicity of glyphosate-based formulations: comparision between different organisms and the effects of environmental factors. Chemosphere. 52(7):1189–1197. 10.1016/S0045-6535(03)00306-0
- USEPA. 2004. Chemical hazard classification and labeling: comparison of OPP requirements and the GHS. [cited 2015 Feb 04]. Available from: http://www.epa.gov/.
- Venier P, Maron S, Canova S. 1997. Detection of micronuclei in gill cells and haemocytes of mussels exposed to benzow[a]pyrene. Mutat Res/Gen Tox En. 390(1):33–44. 10.1016/S0165-1218(96)00162-0
- Vera-Candioti J, Soloneski S, Larramendy MI. 2013. Evaluation of the genotoxic and cytotoxic effects of glyphosate-based herbicides in the ten spotted live-bearer fish Cnesterodon decemmaculatus (Jenyns, 1842). Ecotoxicol Environ Saf. 89:166–173. 10.1016/j.ecoenv.2012.11.028