Abstract
Cytogenotoxic effects of a commercial formulation of ethephon (ETF) and a commercially formulated mixture of ethephon+cyclanilide (ETF+CYC), which are used as plant growth regulators (PGRs) in modern agriculture, were investigated by using the anaphase-telophase chromosome aberration (CA) assay in root meristem cells of Allium cepa L. A. cepa roots were treated with 25 (EC50/2), 50 (EC50), and 100 ppm (EC50×2) of ETF, and with the same concentrations of ETF+CYC, for 24, 48, and 72 h periods. Both compounds increased various types of aberrations in the organization and morphology of the chromosomes in test material. These aberrations were clastogenic (bridges and fragments), aneugenic (disturbed anaphase-telophase, C-mitosis, binucleated cells, vagrant chromosomes, and polyploidy), and sticky (stickiness). Commercial formulations of ETF and ETF+CYC significantly increased the percentage of total aberrations, and the percentage of abnormal cells at all concentrations (25, 50, and 100 ppm), and treatment times (24, 48, and 72 h) when compared with the negative control. However, the commercially formulated mixture of ETF+CYC increased the CA formation more than commercial formulation of ETF alone (except the 50 ppm concentration, for 72 h). In addition, ETF and ETF+CYC significantly decreased the mitotic index (MI) at all concentrations and treatment times compared to both the negative and respective positive control, methyl methanesulfonate. The results of this study indicate that in the tested experimental conditions, the commercial formulations of ETF and ETF+CYC are genotoxic and induce cytotoxic effects in the root meristem cells of A. cepa.
Introduction
Plant growth regulators (PGRs) are an important component of agricultural production, and are used worldwide to improve the quality and yield of crops. The US Environmental Protection Agency (USEPA) proposed that PGRs should only be used at suitable times and quantities as prescribed, and if required (USEPA Citation1998; Bhadoria et al. Citation2015). Excessive use of these chemicals can be harmful to the environment and human health. In many studies, some PGRs have been found to be genotoxic, mutagenic, and cytotoxic in various test systems (Ateeq et al. Citation2002; Priya et al. Citation2014; Kocaman and Bucak Citation2016; Kocaman and Güven Citation2016; Özkul et al. Citation2016).
Ethephon (ETF) (2-chloroethylphosphonic acid), which is a systemic PGR in the defoliants and ethylene releasers classes of growth regulator, has been widely used because of its broad‑spectrum activities. ETF acts by releasing ethylene, which is absorbed by the plant and promotes fruit ripening, coloration, abscission, flower induction, and stimulates the production of endogenous ethylene (USEPA Citation1995). It is also used to promote pre‑harvest ripening of fruits, vegetables, and cereals (Bhadoria et al. Citation2015).
ETF is classified as a “Group D” carcinogen (i.e. not classifiable for human carcinogenicity) because at the time there was insufficient evidence regarding its cancer-causing potential (USEPA Citation1995). In addition, it was considered as non-genotoxic and non-carcinogenic by the European Food Safety Authority (EFSA Citation2008) and by the European Chemicals Agency (ECHA Citation2012). However, ETF has been shown to be mutagenic (Al-Twaty Citation2006; El Raouf and Girgis Citation2011), hepatotoxic (Bhadoria et al. Citation2015), teratogenic (El Raouf and Girgis Citation2011; Wang et al. Citation2011) and genotoxic (Nada and Saleha Citation2008; Priya et al. Citation2014; Hodjat et al. Citation2017) in various test systems. Thus, taking into account these adverse effects of ETF, it becomes necessary to further evaluate its genotoxicological properties.
In modern agriculture, ETF is widely used alone or in combination with cyclanilide (CYC). CYC [1-(2,4-dichloroanilinocarbonyl)cyclopropanecarboxylic acid] is also a PGR that is registered for use only in combination with other PGRs (ETF and mepiquat chloride). When used in combination with ETF, it accelerates senescence processes (enhance defoliation and boll opening) (Burton et al. Citation2008). In this combination, CYC appears to synergize or enhance the activity of ETF (Pedersen et al. Citation2006), because ETF alone will initiate defoliation. Although it has been widely used, there have not been any published studies on the possible genotoxic potential of CYC combinations.
In the present study, the genotoxicity of the commercially named Exit Master, which has 720 g l–1 ETF as its active ingredient, and the commercially named Finish® Pro SC 765, which has 720 g l–1 ETF+45 g l–1 CYC (ETF+CYC) as its active ingredients, were evaluated. When using these compounds in agriculture, not only the active ingredient, but also the whole combination, is applied, so humans, in particular agricultural workers, are exposed to these commercial formulations in the environment.
The Allium test, which is one of the best-recognized cytogenetics assays, was introduced by Levan (Citation1938) and is commonly used for evaluating the potential genotoxicity and cytotoxicity of environmental chemicals (Goujon et al. Citation2014; Pathiratne et al. Citation2015; Bianchi et al. Citation2016).
The purpose of this study was to investigate the genotoxic potential of commercial formulations of ETF and ETF+CYC by using the anaphase-telophase chromosome aberration (CA) assay in the root meristem cells of Allium cepa L. In addition, cytotoxic activity was investigated in Allium roots by monitoring the mitotic index (MI).
Materials and methods
Test samples and chemicals
In this study, the root tip cells of a commercial variety of A. cepa (2n = 16) were used as the test material. The commercial formulations of synthetic PGRs, ETF (Exit Master, containing 720 g l–1 ETF as the active ingredient) and ETF+CYC (Finish® Pro SC 765, containing 720 g l–1 ETF+45 g l–1 CYC as the active ingredients) were used as the test substances, and obtained from Agrofarm and Bayer CropScience, İstanbul, Turkey, respectively. The chemical structures and formulas of ETF and CYC are given in Table . Methyl methanesulfonate (MMS, purity 99%, CAS no: 66-27-3) was used as the positive control, and was obtained from Sigma-Aldrich (St. Louis, MO, USA). Feulgen dye and all other chemicals were purchased from Merck (Darmstadt, Germany).
Table 1. The chemical structures and formulas of ethephon and cyclanilide.
Concentration selection
In this study, the test concentrations used were chosen based on the half-maximal effective concentration (EC50) of the test substance, ETF. The Allium root growth inhibition test was carried out as described by Fiskesjö (Citation1985), with minor modifications for determining the EC50 value of ETF. EC50 is defined as the concentration that produces a 50% decrease in root growth rate when compared with the negative control. Clean and healthy A. cepa bulbs were germinated in distilled water at room temperature (21 ± 2 °C) for 24 h. After the roots had been homogeneously grown, five bulbs (per treatment) were exposed to different concentrations of ETF (40, 50, 60, 70, 80, and 90 ppm), and five bulbs were exposed to distilled water (negative control) for 96 h. During the experiment, the test solutions and distilled water were changed every 24 h. The 10 roots (i.e. 50 roots for each concentration) with the best development at the end of the exposure time were measured, and the mean root length calculated. The relative reduction in root length was calculated as the percentage of the deviation from the negative control. Taking the root length of the negative control as 100%, the EC50 value of ETF was determined as 50 ppm, which inhibits approximately 50% root growths when compared to the negative control root length. Therefore, the concentration range of the ETF was chosen as (25, 50 and 100 ppm), which corresponded to half of the EC50, EC50, and two times the EC50. The experiments were carried out in triplicate. In this study, a commercially formulated mixture of ETF+CYC was also tested at the same concentrations (25, 50 and 100 ppm). Serial dilutions of test substances were made in sterile distilled water, under sterile conditions.
Allium cepa test
Approximately equal-sized (25–30 mm in diameter) and healthy bulbs of a commercial variety of A. cepa were chosen. A. cepa bulbs were germinated in distilled water at room temperature (21 ± 2 °C). When the newly emerged roots had reached 1.5–2 cm in length, they were treated with 25, 50, and 100 ppm concentrations of ETF, and a mixture of ETF and CYC (ETF+CYC), for 24, 48, and 72 h. A negative control (untreated control), and a positive control (10 ppm, MMS) were also employed in every experiment. Five bulbs were used for each concentration, treatment period, and negative/positive control group. After completion of the treatment, roots from five bulbs were immediately cut and fixed in a solution of ethanol (99%) and glacial acetic acid (3:1, v/v) for 24 h. The fixative was replaced with 70% ethanol and stored at + 4 °C until use. For slide preparation, the root tips were hydrolyzed in a solution of 1 N HCl at 60 °C for 7 min. After hydrolysis, the roots were washed with distilled water three times. Chromosomes were stained with Feulgen for 1 h in the dark, followed by squashing in 45% acetic acid (Yıldız and Arıkan Citation2008). Slides were prepared from different onions, and one slide was prepared for each A. cepa bulb. Permanent slides were made from five roots for the control, and for each treatment time, at each concentration.
Microscopic evaluation
All slides were coded and examined using a Nikon E100 light microscope at 400× magnification. The CAs, MI and stage of mitotic cells were investigated for each concentration and exposure time. One hundred anaphase-telophase cells (a total of 500 cells per concentration) were examined per slide, for each treatment to determine CAs. The cytological abnormalities were scored in the mitotic cells and the results were tabulated. CAs were evaluated as clastogenic aberrations (fragments and bridges), aneugenic aberrations (disturbed anaphase-telophase, C-mitosis, vagrant chromosomes, polyploidy, and binucleated cells) and sticky aberrations (stickiness). More than one thousand cells per root from the five bulbs (a total of more than 5000 cells/sample) were scored for the MI, as a measure of cellular division. MI was calculated according to the formula MI = 100 × number of cells in mitosis/total number of cells. In addition, each stage of mitotic division was also scored to calculate the mitotic phases (%). All slides in this study were scored by only one individual (EK).
Statistical analysis
Statistical analysis was performed using one-way analysis of variance (ANOVA). Comparisons between groups were made using a post hoc analysis, least significant difference (LSD) test. Concentration–response relationships were determined from the correlation coefficients (r). A p-value < 0.05 was considered to be significant.
Results
In the present study, commercial formulations of ETF and ETF+CYC significantly decreased the MI at all concentrations (25, 50, and 100 ppm) and treatment times (24, 48, and 72 h) when compared with both the negative control and the respective positive control, MMS (Table ). However, these decreases in the MI were not concentration-dependent (p > 0.05), except for the ETF+CYC for the 24 h treatment period (r2 = 0.998, p < 0.05). As shown in Table , the commercial formulation of ETF decreased the MI as much as or more than the commercially formulated mixture of ETF+CYC. From the results in Table , it was noted that all treatments of ETF and ETF+CYC on the root meristem of A. cepa changed the frequency of mitotic phases when compared with the control group. Application of ETF and ETF+CYC for 24, 48, and 72 h resulted in an increased rate of prophase and metaphase (except 25, 50 ppm for ETF, at 48 h). Anaphase cell rates were lower than the negative control at all concentrations and treatment times for ETF and ETF+CYC (except 100 ppm for ETF+CYC, at 24 and 72 h). In addition, the percentage of the telophase stages decreased in all of the treatments.
Table 2. Effects of ethephon (ETF) and ethephon+cyclanilide (ETF+CYC) on mitotic index (MI) and mitotic phases (%) in the root tip cells of Allium cepa.
The results of the CA assay in Allium root meristem cells after treatment with ETF and ETF+CYC are summarized in Table . In the present study, the commercial formulations of both ETF and ETF+CYC induced a wide range of changes in the organization and morphology of the chromosomes in the root-tips of A. cepa. These abnormalities were classified according to the action mechanisms: The chromosomal fragments and bridges were apportioned into clastogenic aberrations, attributable to direct action on chromosomes; disturbed anaphase-telophase, C-mitosis, vagrant chromosomes, binucleated cells, and polyploidy were apportioned into aneugenic aberrations, attributable to mitotic spindle abnormalities. Finally, according to Goujon et al. (Citation2014), stickiness was classified as sticky aberrations in a specific category, due to various origins. Photographs of the aberrations are presented in Figure .
Table 3. Effects of ethephon (ETF) and ethephon+cyclanilide (ETF+CYC) on chromosomal aberrations in the root tip cells of Allium cepa.
Figure 1. Different types of aberrations induced by ETF and ETF+CYC in root tip cells of Allium cepa (1000×). (a) Chromosome bridge; (b) fragments; (c) disturbed anaphase; (d) disturbed telophase with stickiness; (e, f) C-mitosis; (g) vagrant chromosome; (h) binucleated cell; (i) polyploid cell; (j) stickiness.
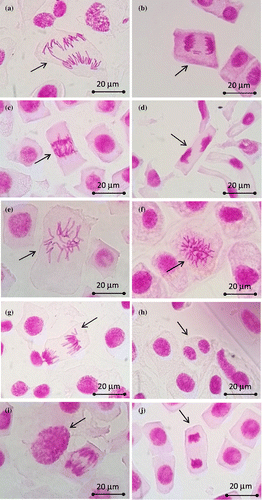
Both ETF and ETF+CYC significantly increased the percentage of total aberrations and also the percentage of abnormal cells at all concentrations (25, 50, and 100 ppm), for all treatment periods (24, 48, and 72 h) when compared with the negative control. However, in general, the aberrations were significantly lower in comparison with the respective positive control, MMS. The results of the CA test also revealed that the commercially formulated mixture of ETF+CYC significantly increased the CA formation more than the commercial formulation of ETF alone (except 50 ppm concentration, for 72 h) (Table ). The results of regression analysis showed that the increases in the CA formation were concentration dependent only for the 72 h treatment period for ETF (for the abnormal cells %: r2 = 0.994, p < 0.05). However, there was no concentration–response relationship in the CA formation for the combination of ETF+CYC, for any treatment times (p > 0.05).
Discussion
In this study, the cytotoxic and genotoxic effects of the commercial formulations of ETF and ETF+CYC, synthetic PGRs, were investigated in A. cepa root tip cells. The A. cepa test system has been widely used for the evaluation of cytotoxic and genotoxic activity of various compounds, due to its high sensitivity and good correlation with other in vivo mammalian assays (Grant Citation1982; Fiskesjö Citation1985; Leme and Marin-Morales Citation2009).
The MI was used to evaluate the cytotoxic potential of the treatments. In this study, with the treatment of ETF and ETF+CYC to the root meristem of A. cepa, the MI decreased significantly in all treated groups, compared to the negative and positive controls. It can be seen in Table that ETF and ETF+CYC significantly decreased the MI at all concentrations (25, 50, and 100 ppm) and treatment periods (24, 48, and 72 h) when compared to the positive control, MMS. Thus, according to the results of this study, it seems that the tested compounds inhibited cell division/cell growth more than MMS, and they showed a greater cytotoxic effect than MMS. In addition to this, the formulation of ETF alone decreased the MI as much as, or more than the combined formulation of ETF+CYC. Significant decreases in MI suggest that the tested formulations of compounds have mito-depressive and cytotoxic action, indicating interference with the normal sequence of the cell cycle, reducing the number of cells starting to divide at interphase. Similarly, Priya et al. (Citation2014) found that 200 and 500 ppm concentrations of ETF caused a significant reduction in the MI of A. cepa root meristems, after 2, 24, and 48 h treatment periods, compared to the negative control. These results are also in agreement with the results of many research groups that have reported the cytotoxic effects of ETF (El Raouf and Girgis Citation2011) and different synthetic PGRs (Ateeq et al. Citation2002; Bhatta and Sakya Citation2008; Kocaman and Bucak Citation2016; Kocaman and Güven Citation2016; Özkul et al. Citation2016).
In the present study, according to the results of the proportions of distribution of particular mitotic phases, the commercial formulations of ETF and ETF+CYC increased the percentage of the prophase stage at all concentrations and treatment times. The results are in agreement with observations of Liman et al. (Citation2010), Priya et al. (Citation2014), and Özkul et al. (Citation2016). Additionally, the percentage of the metaphase stage increased when compared with the negative control group (except 25, 50 ppm for ETF, at 48 h), but this increment was lower than the increment of the prophase stage. On the other hand, generally, percentage of anaphase and telophase stages decreased in all the concentrations and treatment times for both ETF and ETF+CYC applications. From these results, it can be concluded that the reduction of the anaphase and telophase stages, and hence MI, could be explained as being due to the arrest of one or more mitotic stages, or the slowing of the rate of cell progression in mitosis (Ping et al. Citation2012).
Results obtained from this study revealed that a commercial formulation of ETF and a commercially formulated mixture of ETF+CYC induced significant increases in the percentage of total aberrations, as well as the percentage of abnormal cells, compared with the negative control at all of the concentrations (25, 50, and 100 ppm) tested, and in all of the treatment periods (24, 48, and 72 h).
The commercial formulations of ETF and ETF+CYC caused chromosome bridges (Figure (a)) at all concentrations and treatment times with the frequency of 15.10 and 14.39, respectively. Bridges were probably organized with the breaking and reunion of the broken ends of chromosomes or chromatids (Shehab and Adam Citation1983; Liu et al. Citation1996; Türkoğlu Citation2007), or due to unequal translocation or inversion of chromosome segments (Gömürgen Citation2005). In this study, we also observed chromosomal fragments (Figure (b)), but in a low percentage. Fiskesjö (Citation1993) reported that chromosomal fragments can be derived from chromosomal breaks in anaphase bridges, which break up and become acentric fragments. Anaphase bridges and fragments are signs of clastogenic effects, both resulting from chromosome and chromatid breaks (Soh and Yang Citation1993; Leme and Marin-Morales Citation2009). Large numbers of anaphase bridges suggest clastogenic effects of these commercial formulations of PGRs on Allium root tip cells.
Disturbed anaphase-telophase (Figure (c) and 1(d)), as a common type, was the most frequently observed aberration noted in the cells treated with commercial formulations of ETF and ETF+CYC. This aberration may result from a misfunction in activity of microtubules in spindle fibers, leading to an unbalanced chromosome distribution in anaphase (Rank and Nielsen Citation1998). In addition, Suárez and Bullrich (Citation1990) reported that the disturbed polarity at anaphase and telophase might be the result of an alteration in the genes that control the reactions of biochemical pathways determining the position of spindle poles. C-mitosis (Figure (e) and 1(f)) and vagrant chromosomes (Figure (g)) were the other common aneugenic types of aberration found in dividing cells. The occurrence of C-mitosis in A. cepa root tip cells indicated that spindle formation was adversely affected (El-Ghamery et al. Citation2000). According to Rank (Citation2003), vagrant chromosomes are also indicators of spindle poisoning. The formation of these aberrations may be due to the disturbance in the spindle formation, which was affected by the tested substances. Large numbers of disturbed anaphase-telophase, C-mitosis, and vagrant chromosomes suggest that ETF and ETF+CYC act as potent spindle inhibitors (aneugenic agents). Odeigah et al. (Citation1997) reported that C-mitosis is probably indicative of a weak toxic effect, which may be reversible; however it may induce the formation of polyploid cells if it is not reversed.
The other abnormalities induced by ETF and ETF+CYC were binucleated (Figure (h)) and polyploid cells (Figure (i)). However, polyploid cells were observed in low frequency when compared to other aneugenic aberrations. Binucleated cells occur as a result of inhibition of the cytokinesis process or cell plate formation (Gömürgen Citation2005). Microtubules have been implicated in cell plate formation (De Keijzer et al. Citation2014), and these test substances might be affecting these microtubules, resulting in inhibition of cytokinesis. Similar inhibition of cytokinesis cells was also reported by Fernandes et al. (Citation2009), Liman et al. (Citation2010), and Bianchi et al. (Citation2016). Polyploidies could also occur due to disruption of the cytokinesis process, which can promote difficulties in the phragmoplast formation. The lack of the phragmoplast prevents the formation of the daughter cells that lead to the polyploid cells (Fernandes et al. Citation2007). Finally, aneugenic aberrations observed in this study were due to the effect of the tested compounds on the spindle formation and thus resulted in cell division disturbances.
In the present study, stickiness or sticky chromosomes (Figure (j)) were observed in high frequency in root-tips treated with both ETF and ETF+CYC. However, the commercial mixture of ETF+CYC increased the sticky chromosomes to a greater extent, compared with the ETF alone. Chromosomal stickiness is generally regarded as a physiological effect on chromosomes during division (Savage Citation1976), which is indicative of the pollutant affecting the organization of the chromatin. This effect is related to interchromosomal linkages of sub-chromatid strands, coupled with excessive formation of nucleoproteins, inappropriate protein–protein interactions (Badr and Ibrahim Citation1987; Chattopadhyay et al. Citation2004; Türkoğlu Citation2007), and entanglement of chromatin fibers, which lead to subchromatid connections between the chromosomes (Chauhan et al. Citation1986; Patil and Bhat Citation1992). Sticky chromosomes can result from incorrect folding of chromosome fibers, which makes the chromatids link by means of subchromatid bridges (Klasterska et al. Citation1976; Ping et al. Citation2012). It can be seen from Table that the anaphasic bridges were one of the common type aberration, in addition to sticky chromosomes. Kabarity et al. (Citation1974) reported that the stickiness of chromosomes prevented the separation of daughter-chromosomes and thus they remained connected by bridges. Sticky chromosomes have been reported in Allium roots after treatment with various agricultural chemicals, such as herbicides, fungicides, insecticides, and PGRs (Kaymak and Rasgele Citation2009; Yüzbaşıoğlu et al. Citation2009; Lamsal et al. Citation2010; Goujon et al. Citation2014).
Priya et al. (Citation2014) evaluated the genotoxic potential of ETF at 200, and 500 ppm concentrations for 2, 24, and 48 h treatment periods in A. cepa root tips. They reported that ETF caused genotoxic activity in the meristematic cells of A. cepa by inducing the various chromosomal abnormalities, such as laggard chromosomes, fragments, breaks, bridges, rings, clumps, and multipolar and aborted anaphases. These results are consistent with the results of our study, although some different types of CAs were observed. However, in our study, the much lower concentrations of ETF (25, 50, and 100 ppm) caused some of the CA types, such as binucleated and polyploid cells in A. cepa root meristem cells. These differences may have resulted from the differences of concentrations and treatment periods of used.
The results are also in agreement with El Raouf and Girgis Citation2011, who reported that ETF caused mutagenic, teratogenic effects, and increased the chromosomal aberrations (chromatid breaks and gaps, centromeric attenuation, endomitosis, end to end fusion, and fragments) in mouse dams and fetuses. They also found that ETF reduced DNA and RNA concentrations, protein content, and cholinesterase enzyme activity of dams and fetuses. The possible genotoxic effects of ETF was also reported by Nada and Saleha (Citation2008), who observed that ETF increased the chromosomal aberrations in both somatic (bone marrow) and gametic (spermatocytes) cells of male mice. Similarly, Al-Twaty (Citation2006) reported that ETF reduced the DNA and RNA concentrations in the liver and testis, as well as protein content and cholinesterase enzyme activity in the blood plasma of albino mice, concluding that ETF could be mutagenic in mice. Bhadoria et al. (Citation2015) reported that ETF induced inflammatory and degenerative changes in the liver of albino rats, which is suggestive of toxic hepatitis. Recently, Hodjat et al. (Citation2017) reported that ethephon has an in vitro genotoxic effect on murine embryonic fibroblast cells by inducing DNA damage. The results obtained in this study are also in accordance with many previous studies that have reported different and/or the same types of mitotic and chromosomal abnormalities, showing the genotoxic potential of various synthetic PGRs by using the A. cepa test system (Ateeq et al. Citation2002; Bhatta and Sakya Citation2008; Özkul et al. Citation2016).
A literature review has shown that although some toxicological effects of ETF alone have been evaluated in several of the above-mentioned studies, there are no reports of the toxic/genotoxic/mutagenic potential of ETF+CYC as a mixture in any test system. Thus, the results obtained from this study represent the first data related to the genotoxic potential of the commercial combination of ETF+CYC on A. cepa root tip cells. The results of the present study show that a commercially formulated mixture of ETF+CYC induced the CA formation more than the individual formulation of ETF (except 50 ppm concentration, for 72 h). Therefore, there appears to be some form of interaction between ETF and CYC on induction of chromosomal aberrations when compared with exposure to ETF alone. It seems that CYC enhanced the genotoxic effects of ETF in the root meristems of A. cepa.
Conclusion
The commercial formulations of ETF and ETF+CYC induced genotoxic and cytotoxic effects in the root meristem cells of A. cepa, under the conditions of the present study. Therefore, it could be concluded that these PGRs, at the tested concentrations, can show both genotoxic and cytotoxic effects on the genetic material of the exposed organism. Due to the very limited information on genotoxicity of ETF and ETF+CYC, in spite of their widespread use, this study emphasizes the need for further in vivo and in vitro genotoxicity testing of these PGRs formulations.
Declaration of interest
The authors declare that there is no conflict of interest.
Funding
This study was supported by Mustafa Kemal University Scientific Research Projects [project code: 332].
References
- Al-Twaty NHA. 2006. Mutagenic effects of ethephon on albino mice. J Biol Sci. 6(6):1041–1046.
- Ateeq B, Farah MA, Ali MN, Ahmad V. 2002. Clastogeneticity of pentachlorophenol, 2,4-D and butachlor evaluated by Allium root tip test. Mutat Res. 514(1–2):105–113. 10.1016/S1383-5718(01)00327-8
- Badr A, Ibrahim AG. 1987. Effect of herbicide glean on mitosis, chromosomes and nucleic acids in Allium cepa and Vicia faba root meristems. Cytologia. 52(2):293–302. 10.1508/cytologia.52.293
- Bhadoria P, Nagar M, Bahrioke V, Bhadoria AS. 2015. Effect of ethephon on the liver in albino rats: a histomorphometric study. Biomed J. 38(5):421–427. 10.4103/2319-4170.155589
- Bhatta P, Sakya SR. 2008. Study of mitotic activity and chromosomal behavior in root meristem of Allium cepa L.treated with magnesium sulphate. Ecoprint. 15:83–88. doi:10.3126/eco.v15i0.1947.
- Bianchi J, Fernandes TCC, Marin-Morales MA. 2016. Induction of mitotic and chromosomal abnormalities on Allium cepa cells by pesticides imidacloprid and sulfentrazone and the mixture of them. Chemosphere. 144(2016):475–483. 10.1016/j.chemosphere.2015.09.021
- Burton JD, Pedersen MK, Coble HD. 2008. Effect of cyclanilide on auxin activity. J Plant Growth Regul. 27(2008):342–352. 10.1007/s00344-008-9062-7
- Chattopadhyay I, Biswas K, Bandyopadhyay U, Banerjee RK. 2004. Turmeric and curcumin: Biological actions and medicinal applications. Curr Sci. 87(1):44–53.
- Chauhan LKS, Dikshith TSS, Sundararaman V. 1986. Effect of deltamethrin on plant cells I. Cytological effects on the root meristems of Allium cepa. Mutat Res. 171(1):25–30. 10.1016/0165-1218(86)90005-4
- De Keijzer J, Mulder BM, Janson ME. 2014. Microtubule networks for plant cell division. Syst Synth Biol. 8(3):187–194. 10.1007/s11693-014-9142-x
- ECHA. 2012. (European Chemicals Agency) Background document to the opinion proposing harmonised classification and labelling at community level of ethephon. ECHA/RAC/ CLH-O-0000001734-74-03/A1.
- EFSA. 2008. (European Food Safety Authority) Conclusion regarding the peer review of the pesticide risk assessment of the active substance ethephon. EFSA Sci Rep. 174:1–65. doi:10.2903/j.efsa.2006.174r.
- El Raouf AA, Girgis SM. 2011. Mutagenic, teratogenic and biochemical effects of ethephon on pregnant mice and their fetuses. Global Veterinaria. 6(3):251–257.
- El-Ghamery AA, El-Nahas AI, Mansour MM. 2000. The action of atrazine herbicide as an inhibitor of cell division on chromosomes and nucleic acids content in root meristems of Allium cepa and Vicia faba. Cytologia. 65(3):277–287. 10.1508/cytologia.65.277
- Fernandes TCC, Mazzeo DEC, Marin-Morales MA. 2007. Mechanism of micronuclei formation in polyploidizated cells of Allium cepa exposed to trifluralin herbicide. Pestic Biochem Phys. 88(3):252–259. 10.1016/j.pestbp.2006.12.003
- Fernandes TCC, Mazzeo DEC, Marin-Morales MA. 2009. Origin of nuclear and chromosomal alterations derived from the action of an aneugenic agent-trifluralin herbicide. Ecotoxicol Environ Saf. 72(6):1680–1686. 10.1016/j.ecoenv.2009.03.014
- Fiskesjö G. 1985. The Allium test as a standard in environmental monitoring. Hereditas. 102(1):99–112.
- Fiskesjö G. 1993. The Allium cepa test in wastewater monitoring. Environ Toxicol Water Qual. 8(3):291–298. 10.1002/(ISSN)1098-2256
- Gömürgen AN. 2005. Cytological effect of the potassium metabisulphite and potassium nitrate food preservative on root tip of Allium cepa L. Cytologia. 70(2):119–128. 10.1508/cytologia.70.119
- Goujon E, Sta C, Trivella A, Goupil P, Richard C, Ledoigt G. 2014. Genotoxicity of sulcotrione pesticide and photoproducts on Allium cepa root meristem. Pestic Biochem Phys. 113(6):47–54. 10.1016/j.pestbp.2014.06.002
- Grant WF. 1982. Chromosome aberration assays in Allium. Mutat Res. 99(3):273–291. 10.1016/0165-1110(82)90046-X
- Hodjat M, Baeeri M, Rezvanfar MA, Rahimifard M, Gholami M, Abdollahi M. 2017. On the mechanism of genotoxicity of ethephon on embryonic fibroblast cells. Toxicol Mech Methods. 27(3):173–180. 10.1080/15376516.2016.1273425
- Kabarity A, El-Bayoumi A, Habib A. 1974. Effect of morphine sulphate on mitosis of Allium cepa L. root tips. Biol Plantarum. 16(4):275–282. 10.1007/BF02921238
- Kaymak F, Rasgele PG. 2009. Genotoxic effects of raxil on root tips and anthers of Allium cepa L. Caryologia. 62(1):1–9.
- Klasterska I, Natarajan AT, Ramel C. 1976. An interpretation of the origin of subchromatid aberrations and chromosome stickiness as a category of chromatid aberrations. Hereditas. 83(2):153–162.
- Kocaman AY, Bucak S. 2016. Genotoxic and cytotoxic effects of flumetralin in human peripheral blood lymphocytes in vitro. Toxicol Ind Health. 32(12):1927–1934. 10.1177/0748233715595142
- Kocaman AY, Güven B. 2016. In vitro genotoxicity assessment of the synthetic plant growth regulator, 1-naphthaleneacetamide. Cytotechnology. 68(4):947–956. 10.1007/s10616-015-9847-z
- Lamsal K, Ghimire BK, Sharma P, Ghimiray AK, Kim SW, Yu CY, Chung M III, Lee YS, Kim J-S, Shakya SR. 2010. Genotoxicity evaluation of the insecticide ethion in root of Allium cepa L. Afr J Biotechnol. 9(27):4204–4210.
- Leme DM, Marin-Morales MA. 2009. Allium cepa test in environmental monitoring: A review on its application. Mutat Res. 682(1):71–81. 10.1016/j.mrrev.2009.06.002
- Levan A. 1938. The effect of colchicine on root mitoses in Allium. Hereditas. 24(4):471–486.
- Liman R, Akyıl D, Eren Y, Konuk M. 2010. Testing of the mutagenicity and genotoxicity of metolcarb by using both Ames/Salmonella and Allium test. Chemosphere. 80(9):1056–1061. 10.1016/j.chemosphere.2010.05.011
- Liu DH, Jiang WS, Wang CL. 1996. Effect of Zn2+ on root growth, cell division and nucleoli of Allium cepa L. J Environ Sci. 8(1):21–27.
- Nada HAA-T, Saleha YMA. 2008. Genotoxic effect of an organophosphorus pesticide “Ethephon” on somatic and germ cells of male mice. Biosci Biotechnol Res Asia. 5(1):1–8.
- Odeigah PGC, Nurudeen O, Amund OO. 1997. Genotoxicity of oil field wastewater in Nigeria. Hereditas. 126(2):161–167.
- Özkul M, Özel ÇA, Yüzbaşıoğlu D, Ünal F. 2016. Does 2,4-dichlorophenoxyacetic acid (2,4-D) induce genotoxic effects in tissue cultured Allium roots? Cytotechnology. 68(6):2395–2405.
- Pathiratne A, Hemachandra CK, De Silva N. 2015. Efficacy of Allium cepa test system for screening cytotoxicity and genotoxicity of industrial effluents originated from different industrial activities. Environ Monit Assess. 187(12):730. doi:10.1007/s10661-015-4954-z.
- Patil BC, Bhat TGI. 1992. A comparative study of MH and EMS in the induction of chromosomal aberrations on lateral root meristem in Clitoria ternatea L. Cytologia. 57(2):259–264. 10.1508/cytologia.57.259
- Pedersen MK, Burton JD, Coble HD. 2006. Effect of cyclanilide, ethephon, auxin transport inhibitors, and temperature on whole plant defoliation. Crop Sci. 46(4):1666–1672. 10.2135/cropsci2005.07-0189
- Ping KY, Darah I, Yusuf UK, Yeng C, Sasidharan S. 2012. Genotoxicity of Euphorbia hirta: an Allium cepa assay. Molecules. 17(7):7782–7791. 10.3390/molecules17077782
- Priya JS, Purushothaman P, Hannah C, Matthew S, Thomas S. 2014. Genotoxic effect of ethephon on the root meristems of Allium cepa L. Commun Plant Sci. 4(1–2):19–22.
- Rank J. 2003. The method of Allium anaphase-telophase chromosome aberration assay. Ekologija (Vilnius). 2003(1):38–42.
- Rank J, Nielsen MH. 1998. Genotoxicity testing of wastewater sludge using the Allium cepa anaphase-telophase chromosome aberration assay. Mutat Res. 418(2–3):113–119. 10.1016/S1383-5718(98)00118-1
- Savage JRK. 1976. Classification and relationships of induced chromosomal structural changes. J Med Genet. 13(2):103–122.
- Shehab AS, Adam ZM. 1983. Cytological effects of medicinal plants in Qatar III. Mitotic effect of water extract of Anastatica hierochuntica L. on Allium cepa. Cytologia. 48(2):343–348. 10.1508/cytologia.48.343
- Soh WY, Yang WY. 1993. Effect of plant growth regulators on mitotic chromosomes in Allium cepa L. The Nucleus. 36(3):109–113.
- Suárez E, Bullrich L. 1990. Meiotic spindle disturbances an a commercial bread wheat cultivar. Cytologia. 55(1):79–86. 10.1508/cytologia.55.79
- Türkoğlu Ş. 2007. Genotoxicity of five food preservatives tested on root tips of Allium cepa L. Mutat Res. 626(1–2):4–14.
- USEPA. 1995. (United States Environmental Protection Agency) EPA738-R-95-003 (April 1995). registration eligibility decision (RED): Ethephon. Washington: US EPA. Available from: http://archive.epa.gov/pesticides/reregistration/web/pdf/0382.pdf.
- USEPA. 1998. (United States Environmental Protection Agency) Gibberellic acid Tolerence Exemption 10/98, Federal Register 1998; 63: 56882‑6. Available from: http://pmep.cce.cornell.edu/profiles/herb‑growthreg/fatty‑alcohol‑monuron/gibberellic‑acid/Gibberellins_tol_exp_1098.html.
- Wang KS, Lu CY, Chang SH. 2011. Evaluation of acute toxicity and teratogenic effects of plant growth regulators by Daphnia magna embryo assay. J Hazard Mater. 190(1–3):520–528. 10.1016/j.jhazmat.2011.03.068
- Yıldız M, Arıkan ES. 2008. Genotoxicity testing of quizalofop-P-ethyl herbicide using the Allium cepa anaphase-telophase chromosome aberration assay. Caryologia. 61(1):45–52.
- Yüzbaşıoğlu D, Ünal F, Sancak C. 2009. Genotoxic effects of herbicide Illoxan (Diclofop-Methyl) on Allium cepa L. Turk J Biol. 33(4):283–290.