Abstract
Ludwigia L. is a pantropic genus of aquatic and subaquatic herbs. Recently, L. stolonifera (Guill. & Perr.) P.H. Raven became a dominant aquatic macrophyte in Egypt and it expanded from fresh water into salty habitats. Interestingly, the plant showed morphological plasticity in several characters such as leaf shapes, vesicles, flower sex, fertilization efficiency, fruit parameters and number of produced seeds/fruit. Accordingly, in Egypt this species morphologically grouped into seven morphotypes (1–7). The lack of information about the plant genome further complicates the identification of these morphotypes. Thus, it was crucial to investigate the morphotypes in terms of karyotyping and genetic profile to understand if this morphological plasticity is genetically based or it is an impression of habitat diversity. In this study, seven morphotypes located in different Egyptian habitats were compared using karyotyping and random amplified polymorphic DNA (RAPD-PCR) technique. Karyotyping indicated that some morphotypes were tetraploid (2n=32), while others morphotypes were triploid (2n=24). The measured similarity percentage based on RAPD data revealed a highest value (98.6%) between the triploid M4 and M5 morphotypes; similarly between the tetraploid M1 and M2 morphotypes showed 95.4%. Meanwhile, the lowest similarity (73.4%) was between the aquatic morphotype (M2) and the salt affected wetland morphotype (M3). The possible link between the genetic composition and ecological variation of this phenoplastic species will be further discussed.
Introduction
Ludwigia L. is the only genus of the tribe Jussiaeae, family (Onagraceae). The genus diverged from the common family ancestor between 80–83 Ma (Sytsma et al. Citation2004). It is a pantropic genus of 82 species grouped under 23 sections (Ramamoorthy and Zardinin Citation1987; Zardini et al. Citation1991; Zardini and Raven Citation1992); well represented in South and North America, these sections are distributed as: 10 sections (39 species) endemic or centred in S America, three sections (23 species) in N America, five sections (seven species) in Africa, three sections (three species) in Asia (10 species) and two sections are not clearly centred in a single continent (Wagner et al. Citation2007).
In Egypt genus Ludwigia L. is represented by two species, L. stolonifera (Guill. & Perr.) P.H. Raven and L. erecta (L.) Hara. L. stolonifera became one of the dominant aquatic macrophytes in water canals and drains (Täckholm Citation1974; Boulos Citation2000, Citation2009). Meanwhile, L. erecta is very rare in Nile valley and canal banks (Täckholm Citation1974; Boulos Citation2000). L. stolonifera belongs to sect. Jussiaea (L.) Hoch, W.L. Wagner & P.H. Raven, comb. nov., which is characterized by a cosmopolitan distribution and polyploidy. It consists of nine species (11 taxa): three diploid (n=8); four tetraploid (n=16); one hexaploid (n=24); one decaploid (n=40) and one persistent natural triploid hybrid (L. × taiwanensis C. I Peng, 2n=24) based on basic number x=8 (Wagner et al. Citation2007). Seven species of these nine are out-crossing as a group (Raven and Tai Citation1979). L. erecta belongs to sect. Pterocaulon which is a diploid section of five species; four species out of five are autogamous, among them L. erecta (Ramamoorthy Citation1979; Ramamoorthy and Zardini Citation1987).
Economically, L. stolonifera can be used for water bioremediation, helping to improve quality of drinking water (Larson Citation1999); its roots and leaves can be used also as biofilters for heavy metals (Elifantz and Tel-or Citation2002). Many pharmacologists reported the clinical uses of L. stolonifera as hepatoprotective, anti-inflammatory, antidiabetic, antibacterial and fibrinolytic; its aerial parts are composed of metabolites including rutin, kaempferol, quercetin, terpenes and triterpenes. (Firoj et al. Citation2005; Barik and Banerjee Citation2003; Ghani Citation2003).
The growth habit of L. stolonifera showed notable morphological plasticity, and its morphological identification has always been very difficult. From a taxonomic point of view, the above mentioned pheno-plasticity complicates the plant identification (Dutartre et al. Citation2007). Recently, Amer et al. (Citation2016) recorded the plant in different Egyptian habitats, from fresh water to marine and salt affected wetlands habitats; the macro and micro-morphological characters were used to investigate the pheno-plasticity within the Egyptian L. stolonifera populations and finally the study recognized seven morphotypes. Jain (Citation1978), Sultan (Citation1995) and Pigliucci (Citation2001) found that such interspecific variability could be of adaptive significance and affect population fitness, particularly in inhabiting new habitats. However, Karam (Citation1994), Frankel et al. (Citation1995) and EL-Sadek and Ayyad (Citation2000) demonstrated that genetic diversity within species gives the opportunity to evolve under changing environments.
The aim of this work is to study the genetic profile of L. stolonifera morphotypes in Egypt. This is essential to understand how genetic structure is dissipated in relation to different environmental conditions. Chromosome number of each morphotype was determined using karyotype analysis to detect the effect of polyploidy on the overall plant fitness.
Unfortunately, no information is available in the database about the L. stolonifera genome, which further complicates the genome identification of these morphotypes. Accordingly, RAPD technique was selected to study the DNA polymorphism among the seven investigated morphotypes. This postulation was adopted by Fischer et al. (Citation2000), who mentioned that RAPD is a superior technique used whenever prior knowledge of the genome under research is lacking. It is mainly used to determine DNA polymorphism, detect species-specific bands and identify genetic distance to distinguish between investigated taxa (Klinbunga et al. Citation2000). This technique had been proven to be efficient for detecting DNA polymorphisms among various life organisms (Pham et al. Citation2011; Dar et al., Citation2017).
The current study is novel as it determined, for the first time, the chromosome number and DNA polymorphism of the seven L. stolonifera morphotypes. This study will contribute to our understanding of how L. stolonifera, as a model aquatic plant, can adapt and expand into new habitats.
Materials and methods
Plant material
Seven representative specimens of the earlier identified Ludwigia stolonifera morphotypes (M1–M7) were collected from different habitats in Egypt based on Amer et al. (Citation2016) (Table ). The detected morphotypes were characterized by notable morphological variations outlined in Table . Aquadrat of 4 m × 25 m was selected, and 10 individuals were selected to represent each morphotype during this work. Localities are given in Table and mapped in Figure . Voucher specimens of the studied plants were kept in Cairo University Herbarium (CAI).
Table 1. Localities of the collected morphotypes (M1–M7); M=morphotypes. Water depth was measured based on Hamed (Citation2016).
Table 2. Morphological characterization of the studied morphotypes (based on Amer et al. Citation2016). Abundant vesicles ≤ 10 vesicles/node; occasional vesicles ≤ 3 vesicles/node.
Field data
Salinity values of localities for each morphotype are listed in Table . Annual average salinity values (g l–1) were obtained from different certified ecological surveys; Salinity of Faiyum (Tall El-Nayrouz) was obtained from El-Fellaly and Saleh (Citation2004); Kafr Saqr, Qanatir (Gezirat El-shaier) and Ismailia (Tall Al Kabir) was obtained from EEAA Annual Reports (Citation2001, Citation2015); Dakahlia (wet land) and El-Feisha from Serag and Khedr (Citation1996, Citation2001) and Dakahlia (lake Manzala) from Abd El-Rasheed (Citation2011). According to Hamed (Citation2016), the collected morphotypes were supported by water depth ranges of 0.05–2.50 m as shown in Table . However the water depth was apparently not affected as the plant is rooted and all its vegetative organs are floating (Amer field observation).
Cytological procedures
The seven morphotypes (M1-M7) of L. stolonifera were karyotyped to determine their chromosome number. Actively growing root tips from 10 replicates/morphotype were pretreated with 8-hydroxyquinoline for 3–4 h and fixed in Carnoy solution (3 ethanol:1 acetic acid) for 4 h at room temperature. The root tips were then hydrolysed in 0.2 N HCl for 5–7 min at 60°C before staining with 1% orcein (Lobal Chemie, Mumbai, India) according to Fukui and Nakayama (Citation1996). Incubation of root tips in an ice pocket over night before being fixed in Carnoy solution was found more efficient in chromosome dispersal for this plant when compared to 8-hydroxyquinoline treatment.
Data analysis and karyotyping
Chromosome counting was performed on mitotic metaphase cells under a light microscope (Leica DM 2500, Wetzlar, Germany). For each morphotype, 10 clearly observable and well-spread metaphases from 10 individual plants from each morphotype were selected and photographed, using Image Processing Analysis System Standard and high resolution automated karyotyping software processing (Leica CW4000). Metaphase chromosomes of each morphotype were arranged in a decreasing order of size and keeping in view the centromere position that constitutes a karyotype. The length of short arm chromosomes (p) and the lengths of long arm chromosomes (q) were measured and total length (TL) of each chromosome was calculated (p + q). The relative length (RL) of the chromosomes was calculated , the mean relative length (MRL) of each chromosome pair was calculated to represent the relative length of a particular chromosome pair. The centromeric index (CI) was also estimated by
. The mean centromeric index (MCI) was calculated to represent the centromeric index value of particular chromosome pair. MCI was also determining the position of centromere, the chromosome was considered metacentric when the centromeric index was ranged between 45.0 and 50.0 and telocentric when this value was zero (Hassan and Abd El-Gawad Citation2013).
DNA extraction
Genomic DNA was extracted from juvenile plant leaves, five plants/morphotype to illuminate the individual variation were selected to DNA extraction according to Pallotta et al. (Citation2003) with some modifications. Plant leaves (0.3 g) were ground in liquid nitrogen and homogenized in 500 μl lysis buffer (0.1 M Tris-HCl, 0.05 EDTA, 1.25% SDS, pH=8) and incubated at 65°C for 30 min. The samples were cooled down and mixed with 250 μl of 6 M ammonium acetate for 15 min on ice then centrifuged at 4000 rpm for 15 min. DNA in the supernatant was precipitated with 360 μl cold isopropyl alcohol and kept in the freezer for 20 min. The DNA pellet was centrifuged at 4000 rpm for 15 min and washed twice in 70% ethanol. Briefly dried pellet was dissolved in 100–200 μl sterile double distilled H2O. DNA concentration was measured spectro-photometrically. DNA integrity was confirmed on 1% agarose gel.
RAPD-PCR
Genomic DNA (50 ng/sample) was used as a template. Nine random primer pairs were used in this analysis. Sequences of the used primers are listed in Table . The PCR reaction components are as follows: 3 μl DNA template, 1 unit Taq polymerase buffer, 2 μl dNTPs mix (Puregene, PGN019-A, 5 units Taq polymerase (Promega, Madison, WI, USA), 2.5 mM MgCl2, 1 μl of 100 pm primers (forward and reverse, Bio Basic Canada Inc., Ontario, Canada). The PCR program was adjusted as follows: 94°C for 3 min, 30 cycles of 94°C for 30 s, 34°C for 40 s and 72°C for 2 min followed by final extension step at 72°C for 10 min. Samples were cooled down to room temperature and ran onto 3% agarose gel. Molecular weight of different bands was revealed using 100 bp DNA ladder (Vivantis NL 1407, Oceanside, CA, USA). All primers were synthesized by Bio Basic Canada Inc., Ontario, Canada. To assure the reproducibility of the generated PCR bands, the experiment was repeated five times per morphotype to confirm the consistency of the generated bands.
Table 3. Sequence of the used RAPD primers.
NT-SYS-PC DNA analysis
Profiles for RAPD primers were established using the presence 1 or absence 0 method to form the data matrix. Resolved DNA bands on the gel were considered as characters. The level of polymorphism for each marker system was calculated according to Belaj et al. (Citation2003) using NTSYS-PC 2.21 software (Rohlf Citation2009). Matrix was generated on NTedit then standardized on NTSYS-PC 2.21. The dendrograms were obtained through clustering analysis (SAHN) using the unweighted pair-group method (UPGMA) and the Jacquard coefficient based on RAPD data was used to determine the similarity between the studied morphotypes. Analysis NTSYS-PC is software that is used in statistical biology to find relationships in multivariate data based on agglomerative cluster analysis of some similarity and dissimilarity matrix (Rohlf Citation1998; Tarinezhad et al. Citation2005). The genetic distance between morphotypes was measured according to (Nei Citation1972).
Results
Chromosome number
The seven morphotypes of L. stolonifera with its differential phenoplastic characters are outlined in Table . The chromosome count of these morphotypes in mitotic metaphase of the meristematic root cells revealed the presence of two types, 2n=32 and 2n=24. Based on the observed chromosome number as shown in figure , the seven studied morphotypes (M1-M7), were divided into two groups: Group one (M1–M3) exhibited a chromosome set of 2n=4x=32, named as tetraploid, while Group two (M4–M7) exhibited a chromosome set of 2n=3x=24, named as triploid.
Karyotype analysis
Karyotype of the seven L. stolonifera morphotypes are presented in Figure and Tables . Chromosome sets of eight (x=8) were highly variable in their lengths among the different studied morphotypes (M1–M7). The first chromosome pair is the longest one in the complement of all morphotypes; its length ranged from 2.43 to 3.68 μm (in M1 and M2; respectively; Table ) and a mean relative length (MRL) ranged from 17.07 to 18.18% (in M3 and M4, respectively). The last chromosome pair is the shortest one in the complement of all morphotypes; its length ranged from 1.09 to 1.75 μm (in M5 and M2, respectively; Table ) and a mean relative length (MRL) ranged from 7.49 to 9.68% (in M5 and M3, respectively; Table ).
Figure 3. Karyotypes of L. stolonifera morphotypes M1, M2 and M3 are tetraploids (2n=4x=32), morphotypes M4 to M7 are triploids (2n=3x=24). Note the presence of satellite chromosomes on the top of the second chromosome pair of M7.
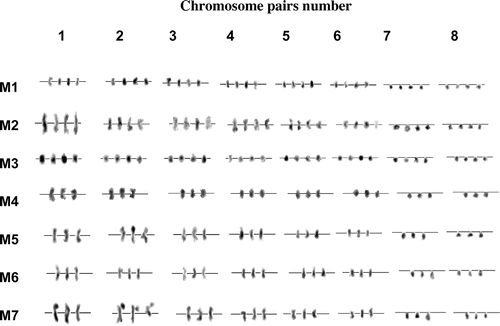
Table 4. Data on homologous chromosome pairs (1–8) in the complement of the seven L. stolonifera morphotypes; (2n=32 in M1, M2 & M3; 2n=24 in M4, M5, M6 & M7).
Table 5. Mean relative length percentage of each chromosome pair with respect to the total genome in the complement of the studied L. stolonifera morphotypes (M1–M7).
Table 6. Mean centromeric index of each chromosome pair in the complement of the studied L. stolonifera morphotypes (M1–M7).
The retrieved results showed that the total genomic length was ranging from 13.45 ± 0.46 μm in M1 to 20.79 ± 0.66 μm in M2 (Table ). However, the overall analysis indicated that chromosomes are exceptionally small in size. The second chromosome pair of morphotype 7 exhibited satellite chromosome (Figure ). The chromosomes are clearly heterogeneous with respect to their mean relative lengths (MRLs), and were arranged from the longest to the shortest (Table and Figure ). The centromeres were telocentrically arranged in all morphotypes within chromosome pairs 7 and 8. In the rest of the chromosomes, centromeres acquired metacentric positions (Table and Figure ).
Molecular fingerprinting
The RAPD fingerprinting results with regard to the number of total, polymorphic, monomorphic, absent and unique bands, are summarized in Table . A total of 86 RAPD bands were revealed by the nine applied primer pairs. Of these, 26 bands are polymorphic and 41 are monomorphic. Obviously, the highest matching among the used primer pairs was OP-M13 & OP-A1, where 11 bands developed, followed by the OP-P2 & OP-A1 primer pair which developed 10 bands. Meanwhile, the lowest matching was noticed with primer pair r 86 & r1325.2, where only three bands developed. One the other hand, primer pair OP-M13 & OP-A1 produced the highest number of polymorphic bands (seven bands), representing the highest polymorphism (63.64%) among the tested primer pairs; in contrast to primers r 86 & r1325.2 and r 38 & r1325.2, which were unable to produce polymorphic bands.
Table 7. Characteristic bands and polymorphism percentages detected with nine primer pairs as resolved from RAPD-PCR analysis.
On the other hand, primer pair OP-M13 & OP-P2 were found to be useful to generate unique band at 2000 bp with triploid (2n=24) marine morphotype (M7). OP-M13 & OP-A1, OP-P2 & OP-A1, r 1302-1 & OP-P2, r 1302-1 & OP-A1 and r 38 & r 86 were able to distinguish triploid M6 from the closely related triploid morphotypes M4 & M5. In the same way, OP-M13 & OP-A1 and r 38 & r 1302-1 were found to be useful to differentiate between the tetraploid (2n=32) fresh water morphotypes M1 & M2 where several bands are missing in M2. The tetraploid wetland morphotype (M3) could be distinguished by the loss of several bands with primer pairs OP-P2 & OP-A1 at 1500 bp, r 38 & r 86 at 500 bp, OP-M13 & OP-P2 at 1500 bp, and others.
UPGMA analysis
The UPGMA tree based on RAPD-PCR data where the developed bands scored 0 for absence and 1 for presence is illustrated in Figure , demonstrating the separation of tetraploid terrestrial morphotype (M3) in separate clusters. The other morphotypes were divided into two groups, with group one including the tetraploid morphotypes (M1 and M2), and group two including the triploid morphotypes (M4–M7). Jaccard’s coefficient based on RAPD data outlined in Table showed that the percentage of similarity between the aquatic tetraploid morphotypes (M1 & M2) was high (95.4%). Similarly, the percentage of similarity between the fresh to brackish triploid morphotypes (M4 & M5) was the highest (98.6%). On the other hand, the lowest similarity was between aquatic morphotype M2 and the salt-affected wetland morphotype M3 (73.4%); however both are tetraploid.
Figure 6. Cluster analysis with UPGMA method of seven related morphotypes (M1–M7) of Ludwigia using RAPD PCR markers based on the Jaccard similarity matrix.
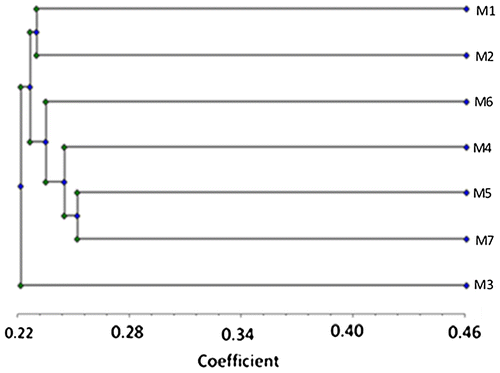
Table 8. Jaccard’s similarity coefficient among the studied L. stolonifera morphotypes (M1–M7) based on RAPD-PCR analysis.
Discussion
The phenoplasticity observed on Ludwigia stolonifera populations in Egypt was found to be correlated with habitat diversity. It was found that L. stolonifera expanded its ecological range in Egypt from fresh water into marine habitats and salt affected wetlands (Amer et al. Citation2016). Many reports found that this eco-plasticity enabled the plant adaption to different habitats by increasing its fitness (Zardini et al. Citation1991; Alpert et al. Citation2000; Bedoya and Madriñán Citation2015). In the current study, cytological tools were used to evaluate the polymorphism among the studied L. stolonifera morphotypes at the DNA level; a trend proposed earlier by Dewey and Dover (Citation1986). This is important for precise identification of L. stolonifera and the correlation between the species genetic fitness and its expansion to new habitat.
Ludwigia stolonifera was reported by Raven and Tai (Citation1979) and Zardini et al. (Citation1991), as tetraploid (2n=32). The chromosome counting of the seven studied morphotypes (Figure ) revealed variations at the polyploidy level and presence of three tetraploid (2n=32) morphotypes (M1, M2 & M3) and four triploid (2n=24) morphotypes (M4, M5, M6 & M7). Raven and Tai (Citation1979) postulated that the fluctuations between diploid and polyploid levels were the characteristic modes of differentiation between individuals within genus Ludwigia.
Despite the report of Wagner et al. Citation2007, who mentioned the presence of triploid (L. x taiwanensis C. I Peng, 2n=24) in sect. Jussiaea which was treated as a persistent natural hybrid, this work is the first to record 2n=24 in L. stolonifera. Nesom and Kartesz (Citation2000) reported that Ludwigia are able to produce hybrids with intermediate morphology when coexisting in the same geographical region “sympatrically”. Seven species out of nine (in sect. Jussiaea) are outcrossing as a group (Raven and Tai Citation1979). However, the origin of the triploid morphotypes by hybridization could not be interpreted due to several points: (1) if we postulated that triploid morphotypes (M4–M7; 2n=24) have been derived from hybridization between L. stolonifera (2n=32) and other diploid species (2n=16), this would not be confidentially supported due to the absence of L. erecta (2n=16) from natural habitats in Egypt c. 50 years ago (Boulos Citation2009). L. erecta is a diploid species naturalized in tropical Africa, outside its ecological range (Wagner et al. Citation2007). L. erecta is an autogamous species (Ramamoorthy Citation1979; Ramamoorthy and Zardini Citation1987). Also, no diploid specimens have been recorded in the field by the authors (personal field experience). In addition, the diploid L. peploides (2n=16) colonized the Mediterranean sea except for the southern part where Egypt is located (Dandelot et al. Citation2005). (2) Peng (Citation1990) found that the natural triploid hybrid in sect. Jussiaea is morphologically intermediate between parents, especially in reproductive features such as the colour of petals; however the studied triploid and tetraploid have the same yellow colour of petals. (3) Peng (Citation1990) also documented that the natural triploids are sterile and their flowers never mature as ripe fruit; however mature fruit and successful seed production was observed in the monitored triploid and tetraploid. The observed differences in ecological preference between triploidy and tetraploidy may have accompanied the change in chromosome number or may have been subsequently acquired. Lewis (Citation1953) suggested a direct relationship between chromosome number and ecological tolerance, clarifying that a change in basic chromosome number by increase or decrease has been associated with changes in ecological adaptation. In general, the occurrence of chromosome races or variants within species are widespread (Semple Citation1974; Murray Citation1976; Ainsworth et al. Citation1983; Parker et al. Citation1991; Ebert et al. Citation1996). The most likely postulation of this study for the origin of the triploidy is that the triploid was derived from the tetraploid in Egypt by certain errors in meiosis, leading to the disappearance of one complete set of chromosomes (x=8); this postulation is supported by Raven and Tai (Citation1979).
Amer et al. (Citation2016) indicated that plants in morphotypes M2, M4, M5 and M6 were characterized by the presence of hermaphrodite flowers together with withered and bagged female flowers located in the same plant. This may indicate that Ludwigia plants may depend on the balance between the number of X chromosomes and the number of autosomes (X/A balance); a feature that determines the final sex of the plant (Navajas-Pérez et al. Citation2005). The case we found in Ludwigia seemed likely to resemble, to a great extent, the case scenario of Rumex plant where sex was controlled by the balance between multiple sex determining chromosomes (Navajas-Pérez et al. Citation2005). No reports could be found for the presence of protandry, protogyny or male sterility in Ludwigia (Raven and Tai Citation1979). However, male sterile flowers were observed in the studied morphotypes M2, M4, M5 and M6 (Table ).
However, some tetraploid and triploid morphotypes develop in similar ecological conditions; the tetraploid morphotype (M2) was dominant in fresh water and the triploid morphotype (M4) can survive in fresh to brackish water (Table ). This result was supported by Hamed (Citation2016), in water analysis where Cl– ion concentrations were 57.38 and 324.66 mg l–1 for M2 and M4, respectively. In addition, NO3– ion concentrations were 0.13 and 25.57 mg l–1 for M2 and M4, respectively.
The tetraploid morphotypes (M1 and M2) showed higher seed productivity (60–80 seeds/fruit) than the triploids (M4, M5 and M6) with 35–45 seeds/fruit (Table ). The disappearance of this set seemed to affect seed productivity from 60–80 seeds/fruit in the tetraploid (M1 & M2) to 35–45 seeds/fruit in the triploid. On the other hand, the low seed productivity (35–45 seeds/fruit) of tetraploid morphotype M3 may be attributed to the exceptional habitat of this morphotype (salt affected wetland, Table ); also this was supported by Hamed (Citation2016) who claimed that the Cl– ion concentration in M3 was 360.36 mg l–1 while in tetraploid morphotypes (M1 & M2) it ranges between 25.56 and 57.38 mg l–1. However, the triploid M7 produced 60–80 seeds/fruit, similar to the tetraploids. The expansion of the morphotype (M7) from fresh to saline water (Lake Manzala average annual salinity 32.29 ± 5.9 g l–1, Table ) might be guarded by the presence of satellite DNA in the second chromosome pair (Figure ). The same scenario was mentioned before in other plants (Hall et al. Citation2003; Mravinac et al. Citation2005; Ugarković and Plohl Citation2002) where satellite DNAs could establish the adaptability of the organism (Pezer et al. Citation2012). Satellite DNAs are usually influenced by gene conversion and unequal crossing over, which result in rapid spread of mutations and modifications among the genome (Dewey and Dover Citation1986; Garrido-Ramos Citation2015). To date, there is no record indicating the presence of satellite DNA in Ludwigia (http://w3lamc.umbr.cas.cz/PlantSat; Macas et al. Citation2011). Thus, the studied triploid M7 produced 60–80 seeds/fruit as the tetraploids (2n=32, as M1 & M2; Table ) and the genetic similarity outlined in Table supports that this morphotype may had been derived from M1, as the similarity between them is 90.6%.
The studied chromosome sets (Figure ) showed notable differences among the studied morphotypes (M1–M7), with respect to their relative chromosome lengths (Table and Figure ) and centromere position (Table and Figure ). L. stolonifera morphotypes were clearly heterogeneous, with respect to relative lengths, and proportions of the proximal, distal regions and sizes; this agrees with (Kurbayashi and Raven Citation1962). A similar observation was reported by Sharma and Sarkar Citation1956 within L. peploides. Raven and Tai (Citation1979) also reported that the family of genus Ludwigia (Onagraceae) is characterized by chromosome-morphological diversity, which support the present results.
However, little is known about how the species can expand its ecological range. Ecological range expansion may increase individual fitness, population growth rate and propagule pressure on adjacent habitats (Matesanz et al. Citation2015). The notable morphological and karyotyping diversity of the studied morphotypes, especially those outside the freshwater habitat, may be attributed to the plasticity of this species to habitat diversity. This concept was supported by Matesanz et al. (Citation2015), who claimed that a number of ecological and evolutionary processes can lead to changes in a species ecological range. Expansion can occur after a species is introduced to a new region if its populations in that region evolve broader individual properties of adaptive plasticity to contrasting conditions (Matesanz et al. Citation2015).
The data retrieved from the molecular markers (Table ) showed the close similarity between the studied L. stolonifera morphotypes. This postulation is supported by the presence of 60.29% monomorphic bands (41 out of 68; Table ). In general, no prior information is available to the authors in relation to the molecular structure of Ludwigia stolonifera. This is the first time to establish a relation between morphotypes using RAPD-PCR markers, thus indicating its efficiency to determine genetic affinity and clarify the interrelationships. The cladistic relationship (Figure ) ordered the seven morphotypes under investigation into three main clusters. The RAPD generated the polymorphic loci out grouped the terrestrial morphotype (M3) in one cluster and the other (aquatic) morphotypes were grouped in other clusters.
The UPGMA tree produced by the RAPD data (Table and Figure ) showed that the common ancestor of morphotypes was 0.24 Nei unit distant (Nei Citation1972). The analysis revealed that the salt-affected wetland morphotype M3 was the most divergent group, distinguished by absence of several DNA bands, developed in other aquatic morphotypes, among these bands at 1800 and 1500 bp for OP-M13 & OP-P2. This morphotype also showed the lowest similarity (73.4%), with the aquatic morphotype M2; however both are tetraploid.
The grouping of the two tetraploid fresh morphotypes M1 & M2 in one cluster seems to be in correlation with their habitat types; also the morphological characters clearly reflect the specific similarity between them especially in bracteoles position on fruit, number of seeds/fruit and density of vesicles (Table , Amer et al. Citation2016). Table shows that the percentage of similarity between the aquatic tetraploid morphotypes (M1 & M2) was high (95.4%).
However, the sex alteration in M2 (presence of female flowers with hermaphrodite; Amer et al. Citation2016), can be correlated with the difference in the total genome length (Table ) between M1 & M2 (13.45 ± 0.46) for the former and (20.79 ± 0.66) for the latter, in line with the absence of several bands developed using OP-M13 & OP-A1 and r 38 & r 1302–1 primer pairs for M2.
The UPGMA tree (Figure ) also grouped the triploid morphotypes in one cluster. However, the morphological variation among them may be incongruent with their variation in karyotype features (Tables ), as they differ considerably in their total genome length, mean relative length, mean centromeric index and presence or absence of satellite chromosome, confirmed by the retrieved molecular data in several points as follows: (1) the only triploid marine morphotype (M7) was distinguished by the presence of a unique band at 2000 bp using the OP-M13 & OP-P2 primer pair. (2) M6 can be separated from the closely related triploid M4 & M5 by the absence of several bands developed by OP-M13 & OP-A and r 38 & r 86 and others, and also by presence or absence of other bands in r 1302–1 & OP-A1. These data are supported by the difference in water salinity (0.320 ± 20 g l–1 for M4; 0.95 ± 20 g l–1 for M6; Table ).
The evidence indicated by karyotype features and molecular diversity between the studied L. stolonifera morphotypes collected from different habitats may reflect the influence of habitat on genetic diversity of the studied L. stolonifera morphotypes and correlate with the expansion behaviour of L. stolonifera in Egypt. Jaccard’s coefficient based on RAPD data, outlined in Table , showed that the percentage of similarity between the studied morphotypes (M1–M7) reflected the possible origin of these morphotypes and confirmed that the interspecific plasticity is genetically based and supported by environmental factors.
Disclosure statement
No potential conflict of interest was reported by the authors.
References
- Abd El-Rasheed ME. 2011. Ecological studies on Lake El-Manzalah with special reference to their water quality and sediment productivity [ M Sc. Thesis]. Egypt: Fac. Sci., Al Azhar Univ.
- Ainsworth CC, Parker JS, Horton DM. 1983. Chromosome variation and evolution in Scilla autumnalis. In: Brandham PE, Bennett MD, editors. Kew chromosome conference II. London: Allen and Unwin; p. 261–268.
- Alpert P, Bone E, Holzapfel C. 2000. Invasiveness, invisibility and the role of environmental stress in the spread of non-native plants. Perspect Plant Ecol Evol Syst. 3(1):52–66.10.1078/1433-8319-00004
- Amer WM, Hamdy RS, Hamed AB. 2016. Macro- and micro-morphological variations of Ludwigia stolonifera (Guill. & Perr.) P.H. Raven in Egypt and its taxonomic assessment. Egypt Journal of Botany, 6 th International conference 11-12 May, Menoufia University. p. 317–354.
- Barik A, Banerjee TC. 2003. Characterization and identification of triterpenes in the weed, Ludwigia adscendens (L.) (Myrtales: Onagraceae) leaves. National Symposium on Biological sciences health and Environmental Aspects, Allied publishers Pvt. Ltd, New Delhi, p. 358–360.
- Bedoya AM, Madriñán S. 2015. Evolution of the aquatic habit in Ludwigia (Onagraceae): Morpho-anatomical adaptive strategies in the Neotropics. Aquat Bot. 120:352–362.10.1016/j.aquabot.2014.10.005
- Belaj A, Satovicg Z, Ciprianil G, Baldoni L, Testolin R, Rallo L, Trujilloi I. 2003. Comparative study of the discriminating capacity of RAPD, AFLP, SSR markers, their effectiveness in establishing genetic relationship in Olive. Theor Appl Genet. 107(4):736–744.10.1007/s00122-003-1301-5
- Boulos L. 2000. Flora of Egypt vol 2: Geraniaceae-Boraginaceae. Cairo, Egypt: Al Hadara Publishing; p. 352.
- Boulos L. 2009. Flora of Egypt checklist revised annotated edition. Cairo, Egypt: Al Hadara Publishing; p. 410.
- Dandelot DS, Verlaque W, Dutartre A, Cazaubon A. 2005. Ecological, dynamic, taxonomic problems due to Ludwigia (Onagraceae) in France. Hydrobiologia. 551:131–136.10.1007/s10750-005-4455-0
- Dar A, Mudigunda S, Mittal P, Arumugam N. 2017. Comparative assessment of genetic diversity in Sesamum indicum L. using RAPD and SSR markers. Biotech. 3(1):7–10.
- Dewey J, Dover M. 1986. New York pesticide recommendations. Ithaca: Cornell University; p. 47-66, 485.
- Dutartre A, Haury J, Dandelot S, Coudreuse J, Ruaux B, Lambert E, Le Goffe P, Menozzi MJ. 2007. Les jussies: caractérisation des relations entre sites, populations et activités humaines. Implications pour la gestion programme de recherche INVABIO, rapport final; p. 128.
- Ebert I, Greilhuber J, Speta F. 1996. Chromosome banding and genome size diferentiation in Prospero (Hyacinthaceae). Plant Syst Evol. 203:143–177.10.1007/BF00985242
- Egyptian Environmental Affairs Agency, Ministry of Environment. 2001. Annual report. Cairo; p. 192. http://www.eeaa.gov.eg/en-us/mediacenter/reports/annualreports.aspx
- Egyptian Environmental Affairs Agency. Ministry of Environment. 2015. Annual report. Cairo; p. 175. http://www.eeaa.gov.eg/en-us/mediacenter/reports/annualreports.aspx
- El-Fellaly S, Saleh EMA. 2004. Egypt’s experience with regard to water demand management in agriculture. Alexandria, Egypt: Eight international water technology conference; p. 5–25.
- Elifantz H, Tel-or E. 2002. Heavy metal biosorption by plant biomass of the macrophyte Ludwigia stolonifera. Water Air Soil Pollut. 141:207–218.10.1023/A:1021343804220
- El-Sadek LM, Ayyad MA. 2000. Genetic diversity as a basis component of biodiversity: case studies in Egypt. In: Nordenstam, B, El-Ghazaly, G, Kassas, M, Laurent, T, editors. Plant systematics for the 21st century. Portland: Portland press; p. 239–250.
- Firoj A, Selim MST, Shilpi JA. 2005. Antibacterial activity Ludwigia ascendens. Fitoterapia. 76(5):473–475.
- Fischer MR, Husi D, Prati M, Peintinger MV, Kleunen, Schmid B. 2000. RAPD variation among and within small and large populations of the rare clonal plant Ranunculus reptans (Ranunculaceae). Am J Bot. 87(8):1128–1137.10.2307/2656649
- Frankel OH, Brown, AHD, Burdon JJ. 1995. The conservation of plant biodiversity. Cambrige: Cambrige Univ. press.
- Fukui K, Nakayama S. 1996. Plant chromosomes: laboratory methods. Boca Raton: CRC Press; p. 241–255.
- Garrido-Ramos MA. 2015. Satellite DNA in plants: more than just rubbish. Cytogenet Genome Res. 146:153–170.10.1159/000437008
- Ghani A. 2003. Medicinal plants of Bangladesh with chemical constituents and uses, thoroughly revised and enlarged. 2nd ed. Dhaka: Asiatic Society of Bangladesh.
- Hall SE, Kettler G, Preuss L. 2003. Centromere satellites from Arabidopsis populations: maintenance of conserved and variable domains. Genome Res. 13:195–205.10.1101/gr.593403
- Hamed AB. 2016. Taxonomic study of genus Ludwigia (Family: Onagraceae) in Egypt [ Ph.D Thesis]. Egypt: Cairo University; p. 136.
- Hassan NA, Abd-El Gawad MH. 2013. Morphological karyotype analysis of eleven pomegranate cultivars. American-Eurasian J Agric & Environ Sci. 13(11):1562–1567.
- Jain S. 1978. Adaptive strategies: polymorphism, plasticity and homeostasis. In: Solbring OT, Jain S, Johnson, GB, Raven PH, editors. Topics in plant population biology. New York, NY: Columbia Univ. press; p. 160–187.
- Karam MA. 1994. Genetic diversity of some medicago species in the western Mediterranean region of Egypt [ Ph.D. Thesis]. Alexandria, Egypt: Alexandria Univ.
- Klinbunga S, Ampayup P, Tassanakajon A, Jarayabhand P, Yoosukh W. 2000. Development of species-specific markers of the tropical oyster (Crassostrea belcheri) in Thailand. Mar Biotechnol. 2:476–484.
- Kurbayashi ML, Raven PH. 1962. A comparative study of mitosis in the Onagraceae. Am J Bot. 9:1003–1026.10.2307/2439214
- Larson R. 1999. Native plants could help improve water quality. Aces news:217–233.
- Lewis H. 1953. Chromosome phylogeny and habitat preference of Clarkia. Evolution. 7(2):102–109.10.1111/evo.1953.7.issue-2
- Macas J, Kejnovský E, Neumann P, Novák P, Koblížková A, Vyskot B. 2011. Next generation sequencing-based analysis of repetitive DNA in the model dioecious plant Silene latifolia. PLoS One. 6(11):1–12.
- Matesanz S, Horgan-Kobelski, Sultan S. 2015. Evidence for rapid ecological range expansion in a newly invasive plant. AOB plants. 7:1–12.
- Mravinac B, Plohl M, Ugarković D. 2005. Preservation, high sequence conservation of satellite DNAs suggest functional constraints. J Mol Evol. 61(4):542–550.10.1007/s00239-004-0342-y
- Murray BG. 1976. The cytology of the genus Briza II. Chiasma frequency, polyploidy and interchange heterozygosity. Chromosoma. 57:81–93.10.1007/BF00292951
- Navajas-Pérez R, de la Herrán R, González GL, Jamilena M, Lozano R, Rejón CR, Rejón MR, Garrido-Ramos MA. 2005. The evolution of reproductive systems and sex-determining mechanisms within Rumex (Polygonaceae) inferred from nuclear and chloroplastidial sequence data. Mol Biol Evol. 22(9):1929–1939.10.1093/molbev/msi186
- Nei M. 1972. Genetic distance between populations. Amer Nat. 106:283–292.
- Nesom GL, Kartesz JT. 2000. Observations on the Ludwigia uruguayensis complex (Onagraceae) in the United States. Castanea. 65:123–125.
- Pallotta MA, Warner P, Fox RL, Kuchel H, Jefferies SJ, Langridge P. 2003. Marker assisted wheat breeding in the southern region of Australia. Proceedings of the 10th International Wheat Genetics Symposium; Sep 1–6, Paestum, Italy. p. 789–791.
- Parker JS, Lozano R, Taylor S, Ruiz Rejôn M. 1991. Chromosome structure of populations of Scilla autumnalis in the Iberian Peninsula. Heredity. 67:287–297.10.1038/hdy.1991.92
- Peng CI. 1990. Ludwigia x taiwanensis (Onagraceae), a new species from Taiwan, and its origin. Bot Bull Acad Sinica. 31:343–349.
- Pezer Z, Brajkovic′ J, Feliciello I, Ugarkovc′ D. 2012. Satellite DNA-mediated effects on genome regulation. Genome Dyn. 7:153–169.10.1159/000337116
- Pham TD, Mulatu G, Tri MB, Tuyen CB, Arnulf M, Anders SC. 2011. Comparative analysis of genetic diversity of sesame (Sesamum indicum L) from Vietnam and Cambodia using agro-morphological and molecular markers. Hereditas. 148:28–35.10.1111/more.2011.148.issue-1
- Pigliucci M. 2001. Phenotypic plasticity: beyond nature and nurture. Baltimore, MD: Johns Hopkins Univ; p. 328.
- Ramamoorthy TP, Zardini EM. 1987. The systematics and evolution of Ludwigia sect. Myrtocarpus sensu lato (Onagraceae). Systematic botany monographs. Ann Mo Bot Gard. 19:1–120.
- Ramamoorthy TP. 1979. A sectional revision of Ludwigia sect. Myrtocarpus sensu lato (Onagraceae). Ann Mo Bot Gard. 66:893–896.10.2307/2398928
- Raven PH, Tai W. 1979. Observations of chromosomes in Ludwigia (Onagraceae). Ann Mo Bot Gard. 66:862–879.10.2307/2398926
- Rohlf FJ. 1998. NTSYSpc numerical taxonomy and multivariate analysis system version 2.0 user guide. Setauket, New York: Applied Biostatistics Inc.; p. 37.
- Rohlf FJ. 2009. NTSYS–PC: numerical taxonomy system. version 2.21. Setauket, NY, USA: Exeter software.
- Semple JC. 1974. The geographic distribution of B-chromosomes of Xanthisma texanum DC. (Asteraceae). I. Survey of the range. Am J Bot. 61:995–1001.10.2307/2441990
- Serag MS, Khedr AA. 1996. The shoreline and aquatic vegetation of El-Salam canal. Egypt J Environ sci. 11:141–163.
- Serag MS, Khedr AA. 2001. Vegetation-environment relationships along El-Salam Canal. Egypt. J Environ. 12:219–232.
- Sharma AK, Sarkar SK. 1956. Cytology of the two species of Onagraceae with special reference to the structural hybridity of Clarika. Phyton (Buenos Aires). 7:69–76.
- Sultan SE. 1995. Phenotypic plasticity and plant adaptation. Acta Bot Neerl. 44:363–383.10.1111/plb.1995.44.issue-4
- Sytsma KJ, Litt A, Zjhra ML, Pires JC, Nepokroeff M, Conti E, Walker J, Wilson PG. 2004. Clades, clocks, and continents: historical and biogeographical analysis of Myrtaceae, Vochysiaceae, and relatives in the southern hemisphere. Int J Plant Sci. 165(4 supplments):85–105.10.1086/421066
- Täckholm V. 1974. Student’s flora of Egypt. 2nd ed. Egypt: Cairo University Press; p. 888.
- Tarinezhad A, Sabouri A, Mohammadi SA. 2005. Statistical software NTSYS pc application in plant breeding. The 7th Conference of Iran Statistics. Tehran, Iran: Allame Tabatabaei University; p. 10.
- Ugarković D, Plohl M. 2002. Variation in satellite DNA profiles-causes, effects. Eur Mol Biol Organ J. 21(22):5955–5959.10.1093/emboj/cdf612
- Wagner WL, Hoch PC, Raven PH. 2007. Revised classification of the Onagraceae. systematic botany monographs. Ann Mo Bot Gard. 83:1–240.
- Zardini EM, Peng CI, Hoch PC. 1991. Chromosome Numbers in Ludwigia sect. Oligospermum, sect. Oocarpon (Onagraceae). Taxon. 40(2):221–230.10.2307/1222976
- Zardini EM, Raven PH. 1992. A new section of Ludwigia (Onagraceae) with a key to the sections of the genus. Syst Botany. 17:481–485.10.2307/2419486