ABSTRACT
A general report on the use of the Allium test as cytotoxicological and genotoxicological assay is proposed, with particular emphasis about the standardization of the test in several common applications. The intraspecific variation in Allium cepa has been overlooked, as in most investigations no mention is made about origin and denomination of the onion cultivar used. A standardization of the used material would allow a better generalization of the results, since we cannot be sure that all cultivars would give the same response. A more frequent use of transmission electron microscopy (TEM) investigation is proposed. Even if relatively time consuming and not available in all laboratories, it may help to better understand the mechanism of cytotoxicity, since many morphological characters may appear similar but be arisen from different processes observable only with TEM. About statistical testing, tests other than chi-squared may be used in case of a lower amount of data. The most commonly used statistical tests are the parametric tests ANOVA and Student’s t, and the non-parametric tests Kruskal–Wallis and Mann–Whitney U, for analysis of variance. Tests should be used also to assess the minimal sample dimension for obtaining significance, since data collection (microscope observation) appears to be one of the main bottle necks of the test. Also the use of the Allium test for testing liposomes and other nanovectors for drug delivery is proposed, in order to assess the cytotoxicity of these types of medium and the possible increase in cytotoxicity of the associated drug.
1. Introduction
The use of the Allium test (AT) to check the potential genotoxicity of several types of chemical, physical and biological agents has a long history in scientific literature, from the first investigation by Levan (Citation1938) to the more standardized method proposed by Fiskesjö (Citation1985) and later Rank (Citation2003). The plants used for the AT are individuals belonging to the species Allium cepa L. (family Amaryllidaceae or Alliaceae, depending on the chosen taxonomic treatment), commonly known as onion. The AT tests genotoxicity using chromosomes. Hence it does not deal with simple point mutations but instead genomic mutations that cause morphological changes on the chromosomes.
The use of a plant, i.e. an organism with relatively high level of complexity, provides data about potential damage to DNA in a multicellular context, a possibility that is not provided by cell cultures, even if the results may be of importance since the genomes of cultured cells may be of mammals, as in the case of mouse bone marrow cells (Nabeel et al. Citation2008; Algarni Citation2018) or even transformed lines of human cells (Li et al. Citation2015). Compared to the use of animals for testing, the AT is more cost effective (Vicentini et al. Citation2001; Teixeira et al. Citation2003; Tedesco and Laughinhouse, Citation2012) and potentially provides a large amount of data with very easy cultivation techniques and without the ethical concerns that affect the use of animals in testing and that necessitate complex breeding guidelines. Moreover, it was shown that comparing the effects of cytotoxicological agents on A. cepa and on animal cell lines, the results obtained were similar and comparable (Tedesco and Laughinghouse Citation2012).
Other plants have also been used for cytotoxicity testing, recent reports being of Vicia faba L. (Kursheed et al., Citation2018), maize (Bonea and Bonciu Citation2017), and Drimia indica (Roxb.) Jessop. (Daphedar and Taranath Citation2018). However, Allium cepa has as an advantage due to its large chromosomes, easily observed with a light microscope; also in relation to the features that may reveal an effect even at relatively low level of interaction of the tested substance with the genetic material, besides its long history of use as cytotoxicological test. As it is an in vivo test, the data can be used for assessment of genotoxicity on plants and even for eukaryotes in general, including humans.
A simple search on Google scholar on 21 June 2018 with the key “Allium test” produced 3170 hits, showing the wide use of the test in a variety of different investigations. A few examples are studies examining the effects of toxic plants (Khan et al. Citation2010; Pesnya et al. Citation2017), nanoparticles (Yekeen et al. Citation2017), synthetic plant hormones such as etephon (Yavuz et al., Citation2017), industrial waste (Firbas and Amon Citation2017; Dutta et al. Citation2018), aquatic environmental samples (Firbas Citation2015), fungal extracts (Dávila Giraldo et al. Citation2018), herbicides (de Souza et al. Citation2016), insecticides (Souza et al. Citation2017), fungicides (Dane and Dalgiç Citation2005), pesticides (Fatma et al. Citation2018), radioactivity (Evseeva et al., Citation2003) and heavy metals (Liu and Kottke Citation2003), and the mitigating effects of antioxidant or vitamins in case of treatment with genotoxic agents (Asita Okorie et al. Citation2017).
2. Features of the biological matrix: Allium cepa L. taxonomy and intraspecific variation
Genus Allium belongs to the family Alliaceae subf. Allioideae. It is a large genus, comprising more than 800 species (Li et al. Citation2010) and is widely distributed in the Northern Hemisphere. A phylogenetic analysis based on internal transcribed spacer (ITS) sequences showed that Allium cepa, part of section Cepa, was strictly related to A. vavilovii (Friesen et al. Citation2006), a position confirmed by chloroplast sequences (Li et al. Citation2010).
The ancestral karyotype of genus Allium seems to be x = 8 (Peruzzi et al. Citation2017). A. cepa has 2n = 16 chromosomes (data recorded from many locations in the database Index to Plant Chromosome Numbers (IPCN) http://www.tropicos.org/Project/IPCN). However, it is interesting to observe that the variety A. cepa var. viviparum Alef. has been reported to have 2n = 24, hence it would be a triploid (Puizina and Papea Citation1996).
Moreover, Kim et al. (Citation2015) observed large insertion in some accessions (and not in others!) of onion due to transposable elements. Other variation was detected on the base of volatile compounds emission (flavor) with an electronic nose system (Russo et al. Citation2013).
In fact, intraspecific variation in A. cepa may have been overlooked, as in most investigations no mention is made about the characterization of the onion cultivar used in cytotoxicological testing, apart from a few reference to cv. A. cepa (Stuttgarter Riesen). Hence, we may suppose that there is no general rule about the employed genetic material (cultivar, clone or variety).
3. Observed characters for assessing the cytotoxic/genotoxic effects
In the presence of certain external stimuli, the cellular progress can be blocked in one of the phases of the cell cycle or cell division, and their action is called mitoinhibition. Mitogens act to overcome intracellular braking mechanisms that block cell cycle progression, and their action is called mitostimulatory.
Any deviation from the orderly and directed progression of the cell cycle, and respectively, of mitosis and cytokinesis, is reflected in a state of cytotoxicity and genotoxicity. These are evaluated by the mitotic index (MI; a measurement to determine the percentage of cells undergoing mitosis), percentage of cells in each mitosis phase (prophase, metaphase, anaphase and telophase index), as well as a series of clastogenic, aneugenic and turbagenic changes.
Common clastogenic effects/aberrations include chromosome and/or chromatid fragments, interchromatid or subchromatid connections, nucleoplasmic bridges, heteromorphic chromosomes, dicentric or ring chromosomes, and micronuclei (MNs). Bimitosis and asynchrony of the cell cycle could also be added.
Interchromatid or subchromatid connections, known as chromosomal bridges, are chromosomal structural changes that may result from exchanges between homologous or non-homologous chromosomes, and may be the consequence of dicentric chromosome formation or poor activity of replication enzymes.
Nucleoplasmic bridges originate from dicentric chromosomes or occur as a result of a faulty longitudinal breakdown of sister chromatids during anaphase. The formation of ring chromosomes is explained by the production of two simultaneous breaks in the same chromosome and the subsequent union of non-centromeric fragments. Chromosome breakage, followed by rejoining of proximal chromatid breaks, leads to the formation of dicentric chromosomes. MNs, indicators of chromosomal genotoxicity and instability, are formed from one or more chromosomes.
Cell cycle asynchrony, including internuclear asynchrony, can be observed in large multinucleate cells in which nuclei coexist with early phases of mitosis, inter-chromosomal asynchrony manifested by an uneven chromosome condensation throughout the mitosis stages, and intra-chromosomal asynchrony which is manifested by a gradient condensation of chromatin. When the binucleate cell divides, the two nuclei enter mitosis synchronously, which is called bimitosis (Rieger et al. Citation1991). Turbagenic changes include laggards (delayed chromosome movement at poles), vagrants or forward chromosomes (precocious movement of chromosome to mitotic spindle poles), and star-like polar anaphase. It is believed that the formation of lagging chromosomes (laggards) is due to inhibition of tubulin polymerization or cytoskeletal proteins.
Aneugenic changes include sticky chromosomes, C-mitosis, and nuclear buds. According to Kurás (Citation2004), the change in the ratio between the amount of histones and other proteins that ensure the optimal organization of nuclear chromatin may increase its adhesiveness, often leading to the formation of atypical metaphases and anaphases, chromosomal bridges in anaphase and telophase, and finally inhibition of cytokinesis and the formation of binucleated cells. Nuclear buds are considered as markers of polyploidization events and gene amplification, and their formation leads to the expulsion of excess genetic material from aneuploid cells (Fernandes et al. Citation2007). Binucleated cells may be the consequence of an aberrant division of the spindle in early anaphase or the inhibition of cytokinesis after telophase. Giant cells may be polyploid cells that have occurred through endoreplication or endomitosis. C-mitosis, also called stathmokinesis (D’Amato Citation1950), is the mitosis in which the poisoning effect of colchicine blocks the progression of the metaphase cell in the anaphase, and consequently the cell becomes polyploid.
Allium cepa L. has a diploid (2n = 2x = 16) genome with monocentric chromosomes, with basic chromosome number x = 8. The chromosomes are relatively large and so appropriate for the detection of karyomorphological changes. The chromosomes are metacentric (1, 4, 7 couple), submetacentric (2, 3, 5, 8 couple), and subtelocentric (6 couple). Karyotypes can provide information about cytology, cytosystematics and cytogenetic relationships, evolutionary origins of species and, as with the AT, genomic aberration. A. cepa (Stuttgarter Riesen) is suitable for the detection of genotoxic agents effects that are manifested in the form of clastogenic and aneugenic effects (Firbas and Amon Citation2014).
A classification model for the A. cepa karyotype consists in a symmetric/asymmetric index S/AI = 1.7500. The monosomy karyotype without subtelocentric chromosomes has a symmetric/asymmetric index of S/AI = 1.6666 and the trisomy karyotype with a subtelocentric chromosome has a symmetric/asymmetric index of S/AI = 1.8235. All investigated ploidy series in A. cepa showed symmetric or between symmetric and asymmetric karyotypes (S/AI = 1.6666–2.8571). Investigated ploidy series in genus Allium L. showed a full symmetric to asymmetric karyotype (S/AI = 1.0000–3.5714). The symmetry/asymmetry index (S/AI) was applied to 579 taxa of genus Allium as an example. The formula includes chromosomal number, chromosomal type and centromeric position. The general formula is described with the different use of chromosome type by Eroğlu (Citation2015).
The observable characters to be recorded range from macromorphological effects (chlorosis, slow growth, reduced root production, as in ), if the plants are allowed to grow enough to produce a stem, to the mitotic index, including the presence of micronuclei, and the effects on chromosomes during mitosis.
The MI is defined as the number of cells undergoing mitosis divided by the total number of cells. This character can be measured with a variable number of repeats, each with a microscope observation of a given size measured on the slide at a given magnification. All stages of mitosis should be included in the count. Both higher and lower MI with respect to the control can be related to an alteration of mitosis mechanisms as a result of cytotoxic effects (Leme and Marin-Morales Citation2009).
The presence and number/frequency of MNs is another important character and consists in the presence, after mitosis, of a nucleus of reduced dimension in one of the daughter cells. Despite being a quantitative character (dimension of a nucleus) it is relatively easy to assess. The MNs may derive either from extended chromosome aberrations (CAs) or breakage or, possibly, from chromosome rearrangements after polyploidization events (Fernandes et al. Citation2007).
Another category of characters is CAs, i.e. modifications in chromosome number and/or structure. The cause of the aberrations may be DNA breaks, inhibition of DNA synthesis and repair or replication of mutated DNA. Change in chromosome number (aneuploidy for single chromosomes or polyploidy for genome duplication) may be due to alterations during replication or chromosome segregation. For this analysis it is necessary to subdivide mitosis in its phases (prophase, metaphase, anaphase, telophase), each with its specific CAs (Leme and Marin-Morales Citation2009). The CAs can be classified into clastogenic and aneugenic, depending on whether a break occurs at the chromosome level (DNA molecule) or if there are problems with the mitotic spindle and hence with the chromosome segregation as well as the inhibition of cytokinesis. The main CAs that characterize clastogenic action are chromosome bridges and chromosome breaks; laggard chromosomes, chromosome loss, C-metaphase, chromosome stickiness and multipolar divisions are induced by aneugenic agents (Sharma et al. Citation1990). The most important aberrations to be taken into consideration are bridges and breaks (clastogenic activity); and chromosome loss, delays, adherence, multi-polarity as result of aneugenic agents (Leme and Marin-Morales Citation2009).
The use of colchicine during chromosome preparation destroys the spindle microtubules, allowing a better observation of the metaphase plates (, )), but influencing the chromosome movement.
Figure 2. (a) A. cepa L. metaphase in preparation without colchicine. (b) A. cepa L. metaphase chromosome (2n = 16) pretreated with colchicine.
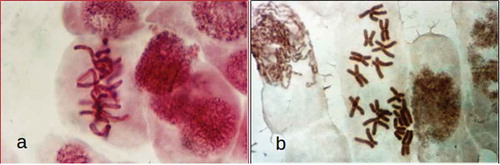
Nucleus modifications can also be recorded directly in the interphase cells: Nuclei can appear lobated, sometimes with small lobes or, in other cases with large bulges. Also two or more nuclei may be present and some cells may be of smaller size than the surrounding cells.
4. TEM ultrastructure of effects on Allium cepa cells in cytotoxicity tests
Ślusarczyk et al. (Citation2014, Citation2015) used the AT for studying antimitotic effect of selenium compounds. They compared the activity of two compounds (an organic and inorganic one) containing selenium in the oxidation state of + 4: Selol and sodium selenate (IV). Selol is a mixture of selenitetriglycerides, obtained by esterification of pre-oxidated triglycerides from sunflower oil with selenous acid (Fitak et al. Citation1999). Its anticancer and antimitotic properties have been studied for several years. At the first stage of the investigations, Ślusarczyk et al. (Citation2014) analyzed the structural changes in cells, their division activity (MI), and proportions of division phases (phase index). Incubation in both selenium compounds led to changes in chromosome structure, consisting in their strong condensation and contraction (cc-chromosomes) as in –) and –), which was accompanied by gradual reduction or complete inhibition of cell division. Treatment with Selol resulted in changes in chromosome structure, condensation and contraction, which blocked passage to subsequent division phases. High concentrations of Selol caused strong condensation of chromatin in interphase cells. Instead, in cells treated with sodium selenate (IV), characteristic “pseudoapoptotic” bodies appeared, and complete fragmentation of nuclei occurred. The effect of sodium selenate (IV) consisted in increased prophase index, and its high concentrations led to inhibition of divisions and arrest of cells at different stages of mitosis.
Figure 3. Changes in chromosome structure and ultrastructure in Allium test cells following incubation in Selol solutions at concentration of 100 μg ml−1 and 800 μg ml−1 Se for 48 h incubation periods. (Ślusarczyk et al. Citation2014, Citation2015). Squeezed preparation, stained with 2% aceto-orcein, observation under a light microscope; bar = 10 μm. (a) Chromatin de-condensation at telophase stage. (b) Change of shape of cell nuclei (contraction and condensation of chromatin) following 48 h of incubation. (c) Transition to apoptosis and formation of apoptotic bodies (arrows) after prolonged incubation in higher concentrations of Selol. (d) TEM image. Portion of a cell following incubation in Selol solution of 800 μg ml−1 Se for 48 h. Nucleus typically changed shape with condensed chromatin (arrow). Bars = 2 μm. d – deposits, m – mitochondria, N – cell nucleus, n – nucleolus, p – plastids, v – vacuole.
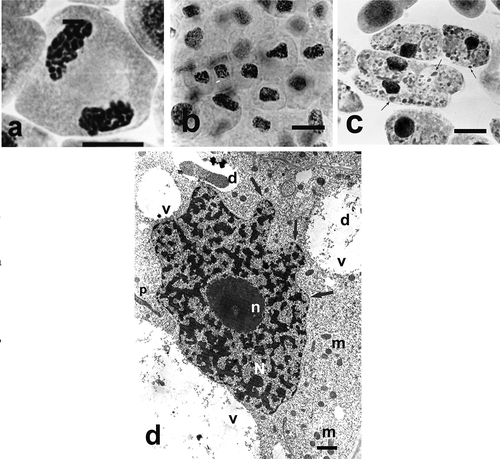
Analysis of ultrastructural changes in apical meristem cells of onion (Allium cepa L.) roots subject to treatment with both selenium compounds (Ślusarczyk et al. Citation2015) was a valuable addition to the above-described studies. It confirmed high toxicity of sodium selenate (IV), manifested by degradation of nucleus, cell organelles and cytoplasm. Treatment with sodium selenate (IV), in almost all tested concentrations and incubation times, caused drastic changes in cell ultrastructure, clearly showing its toxic effect. Prolonged incubation in the highest used concentration of sodium selenate (IV) (400 μg ml–1) resulted in total disintegration of cytoplasm and organelles within it, and the cells were filled with large vacuoles. Only the cell nucleus remained, extremely shrunk, without nucleolus and with perforated chromatin forming specific chromatin protrusions (, )). Treatment with Selol did not result in such dramatic changes in cell ultrastructure. They consisted in gradual increase of heterochromatinization of cell nuclei ()) and change of their shape correlated with increasing concentrations of Selol solutions and the incubation period.
Figure 4. Changes in chromosome structure and ultrastructure in Allium test cells following incubation in sodium selenate (IV) solutions at concentration of 100 μg Se ml−1 and 400 μg ml−1 Se after 48 h of incubation. (Ślusarczyk et al. Citation2014, Citation2015). Squeezed preparation, stained with 2% aceto-orcein, observation under a light microscope; bar = 20 μm. (a) Thickened and shortened chromosomes, forming atypical C-metaphases. (b) A cell with micronuclei. (c) Typical protrusions of chromatin (arrows) formed in prolonged incubation (48 h) in sodium selenate (IV). (d) A cell following 48 h of incubation in sodium selenate (IV) solution. Degraded cytoplasm and organelles, cell nucleus displaying chromatin leaking out (arrows). (e) Strongly degraded cell nucleus with chromatin leaking out (arrows). bars = 5 μm. cw – cell wall, lb – lipid body, N – cell nucleus.
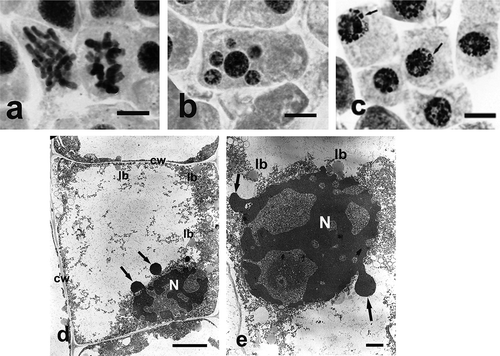
Observations in TEM allowed a more complete interpretation of the results of those studies. In the case of sodium selenate (IV), higher concentration and longer incubation time could trigger the process of programmed death in meristematic cells, the course of which resembles apoptosis process in animals, characterized by formation of apoptotic bodies. The process of apoptosis may develop according to different patterns in plant cells (Krishnamurthy et al. Citation2000; Papini et al. Citation2011). It is noteworthy that, as shown by earlier studies (Ślusarczyk et al. Citation2014), cells treated with Selol stop dividing but the structure of their interphase nuclei remains unchanged. Only highly concentrated solution (1600 μg Se ml–1) caused changes in cell nuclei structure. Additionally, no damage to outer or inner membranes of cell organelles was observed under treatment with Selol. This may mean that the effect of Selol is more specific than that of sodium selenate (IV), and it does not include general intoxication of cells
Studies performed using TEM revealed changes occurring in root apical meristem cells of onion (Allium cepa L.) subject to incubation in a range of concentrations of both selenium compounds. They also allowed interpretation of probable mechanism of action of both compounds, which is extremely important in assays of toxicity of potential drugs.
Thus the use of TEM images provided insights that can be considered very valuable in order to understand the mechanism of cytotoxicity of the tested compounds.
5. Statistical treatment of Allium test cytological data
Tedesco and Laughinghouse (Citation2012) proposed as a standard to use 50 ml plastic cups containing tap (unpolluted) or distilled water. The waiting time for roots collection cannot be standardized, since it may depend on temperature, unless growth chambers are used. However, it is possible to take into account the length of the roots and then to collect them (1.5–2 cm).
Tedesco and Laughinghouse (Citation2012) proposed as a standard experiment the use of five different groups of bulbs, one for the negative control, one for the positive control with a known genotoxic agent such as glyphosate, the aneugenic trifluralin (Fernandes et al. Citation2007) or methyl methanesulfonate (MMS), this last widely used as positive control, since it is clastogenic, and three different concentration of the agent to be tested. The number of cells analyzed for each bulb should be 500–1000 and better if more bulbs are used for each experimental condition.
The raw data should be a matrix containing columns with each observation (each observation should refer to a given square of known surface area) and rows with the character type. Possible character types may be: number of cells in mitosis divided by total cells (MI); nuclear alterations, such as MNs; double nuclei; lobated nuclei; and several CAs. All the parameters are calculated in relation to the total number of cells analyzed in each slide, for example, for the MI prophase, metaphase, anaphase and telophase cells should be summed and divided by the total number of cells.
Considering that DNA alterations can occur spontaneously or can be induced by a substance, then hypothesis-testing methods to detect if there is a significant difference in the evaluated effect between control and a given concentration are very suitable and they have been standardized in guidelines of OECD (Organisation for Economic Co-operation and Development) and ISO (International Organization for Standardization) (e.g. OECD Citation2006).
According to Barbério et al. (Citation2011) the most commonly used statistical hypothesis tests for analysis of variance are the parametric tests, i.e. ANOVA (analysis of variance) and Student’s t-test, and the non-parametric tests, i.e. Kruskal–Wallis and Mann–Whitney U; after analysis of variance, post-tests for multiple comparisons such as Tukey’s, Dunnett’s, Dunn’s should follow.
The use of suitable tests for analyzing variance and post-tests should be done according to normality and homogeneity of data. The Shapiro-Wilk's Kolmogorov–Smirnov’s, D’ Agostino Omnibus’ tests can be employed to assess normality and on the other hand the homogeneity of variances can be assessed by Levene’s, Bartlett’s, Cochran’s tests. To achieve normal distribution or homogeneous data it is possible to apply mathematical procedures to the dataset and thus change it, to analyze the data with parametric tests like ANOVA/Dunnett’s. It is known that parametric tests are more sensitive to significant differences between groups, thus the data transformation is important to use parametric tests afterwards. The significance level employed in the hypothesis tests is usually p < 0.05 (OECD Citation2006).
The most commonly used statistical test is the chi-squared test (Pearson Citation1900), with at least p < 0.05. The most frequently used open source software is R programming language (https://www.r-project.org/).
6. A. cepa as bioindicator of herbicidal effects
Pesticides are bioactive molecules that can form metabolites, and due to their electrophilic characteristics, they are able to react and to combine with biomolecules, e.g. DNA, and to induce changes in them (Rodrigues Citation2002). Herbicides are a group of pesticides used to combat and inhibit undesired weeds. They can be classified as selective and non-selective, according to the target weeds (Song Citation2014).
Among the different pesticide classes, data from 2015 show that the herbicides predominate, in the amount used (55.4%) and in sales (60.4%) around the world (Casida and Bryant Citation2017). Environmental contamination by pesticides is a very important problem, because of the risk to non-target organisms in different ecosystems; the use of herbicides has induced a lot of damage to the environment (Mahmood et al. Citation2014).
Higher plants can be used as bioindicators to evaluate the toxicity of pesticide compounds by mean of different biomarkers (De Souza et al. Citation2016). The use of higher plants as bioindicators is standardized by international programs such as the International Program on Plant Bioassays (IPPB) of the United Nations Environment Program (UNEP) (Ma Citation1999) and Geno-Tox of the US Environmental Protection Agency (USEPA) (Ma et al. Citation2005). The data obtained from these bioassays are recognized as effective to detect environmental genotoxicity by USEPA and the World Health Organization (WHO) (Palmieri et al. Citation2016).
The use of parameters related to germination and root growth to assess toxicity of several substances has been increasing, because data about germination can provide information about lethal effects of the compounds, and delay in germination or in root growth can provide information about sub-lethal effects (Sobrero and Ronco Citation2004). With root growth data of all treatments and negative control of a bioassay, the concentration at which 50% inhibition of root growth occurs when compared with negative control (IC50) can be estimated.
Root growth has been linked to increase in MI because some authors have related the growth of organs in plants with continuous cell division cycles (Silveira et al. Citation2017). MI has been described as a biomarker of cytotoxicity. Besides MI, the main biomarkers employed in A. cepa are CAs and MNs. MNs can be evaluated also in F1 root cells and daughter cells (Ma et al. Citation1995; Leme and Marin-Morales Citation2009). In meristematic cells, CAs and MNs have been used as biomarkers of genotoxicity and chromosomal instability events, which, if not repaired, may indicate a mutagenic effect. Therefore, in the F1 MNs can indicate mutagenic potential (Souza et al. Citation2017).
At the beginning of the twenty-first century, molecular cytogenetic approaches started to be used in genotoxicity evaluations. Methods such as TdT-mediated dUTP nick end labeling (TUNEL assay), single cell gel electrophoresis (comet assay), and fluorescent in situ hybridization (FISH) have been employed in genotoxicity studies showing reliable results (Silveira et al. Citation2017; de Souza et al., Citation2017b).
De Souza et al. (Citation2016) reviewed the action of at least 30 herbicides in A. cepa. In this review the authors found that the early studies were more descriptive and qualitative. But over the years there was the need to compare the treatments with negative control through statistical tools and thus the analyses became more quantitative. Since there is a consensus that all the DNA alterations can occur spontaneously or can be induced by a xenobiotic, the difference between normal and induced alterations can be answered only by statistical analyses.
The AT has been employed to evaluate the toxicity of many active ingredients of herbicides as well as commercial formulations. Wherever organisms are exposed to commercial formulations, the active ingredients and the excipients separately may produce different results. Studies evaluating the toxicity and genotoxicity of these complex mixtures are therefore important (Rossi et al. Citation2018). The bioindicator A. cepa was used to evaluate the genotoxicity of Roundup®, a non-selective herbicide widely used worldwide, and its active ingredient, glyphosate isopropylamine. The authors concluded that the Roundup® was able to induce chromosomal aberrations, but its active ingredient was not genotoxic. Thus, this effect may be due to some other ingredient in the commercial formulation (Rank et al. Citation1993).
The genotoxicity of a widely employed selective and synthetic herbicide, 2,4-D, has been evaluated since early studies with AT. At the beginning, the data were more descriptive (Croker Citation1953) and most studies investigated the active ingredient (Pavlica et al. Citation1991; Ateeq et al. Citation2002). However, de Souza et al. (Citation2017a) evaluated a commercial formulation of 2,4-D, DMA 806 BR®, at the field concentration and fractions. The authors inferred that the effects of the commercial formulation were very similar to those observed with the active ingredient in past studies, such as roots with tumors, reduced root growth, aneugenic chromosomal aberrations and binucleated cells.
In addition, de Souza et al. (Citation2017a) concluded that 2,4-D must have an effect like that of the herbicide trifluralin on the mitotic spindle and it can block cytokinesis because the phragmoplast is not formed. In addition, the commercial formulation of 2,4-D can induce cellular death, as observed in classic cytogenetic analyses, as well as in molecular approaches like the TUNEL test.
We can conclude that the AT is an effective bioassay to evaluate herbicidal effects and is on a par with molecular cytogenetic approaches.
7. Cyto-genotoxicity of fertilizers monitored by Allium root tip bioassay
The dietary requirements of the increased world population in the twentieth century would not have been met without a parallel growth in food production, and this was achieved due to fertilizers. Organic fertilizers were used since the end of the nineteenth century, but the introduction of mineral phosphate fertilizers took over in the beginning of the twentieth century and has been continuously increasing. The use of phosphates, together with development of improved crop varieties with higher yields, led to the “green revolution,” and the production of cereals more than doubled per unit area of agricultural land.
It was however soon realized that the application of fertilizers was causing contamination at both local and global scale, altering the physico-chemical and nutrient status of the soil, thereby effecting the flora. Fertilizers are reactive compounds that can form covalent bonds with different nucleophilic centers of cellular biomolecules, including DNA (Crosby Citation1982).
Mitotic activity and chromosomal behavior was observed in four different concentrations of magnesium sulfate at four different durations of treatment using Allium cepa as a biological system by Bhatta and Sakya (Citation2008). Mitoinhibitory effect of the compound was observed at higher concentration. The compound was capable of producing various chromosomal abnormalities indicating the cytostatic and clastogenic properties of magnesium sulfate.
Chaurasia and Sinha (Citation1987) observed both mitoinhibitory and clastogenic effects in Allium root tip meristem by treatment with urea. Urea, one of the most common fertilizers used to supplement soil with nitrogen, was found to cause chromosomal abnormalities such as breaks, clumping, pulverization and polyploidy in albino Swiss mice. The agrochemical could act as a mitoinhibitor in onion root-tip cells, where fragmentation of chromosomes was found to be the most common among the abnormalities induced, thereby clearly indicating the reliability of the AT.
Srivastava and Srivastava (Citation2009) observed decreased MI with increase in concentration of urea and also that frequency of CAs were directly proportional to the concentration. Breakdown of soil urea yields ammonium ions or ammonia, which further convert to nitrite and nitrate due to nitrification. These breakdown products have severe toxic effects on the crop standing in the soil, when present in high concentrations. Treatment of different concentrations of urea was given to Allium cepa bulbs for different durations and variation was observed in the percentage of MI and percentage of anomalous cells during the span of the experiment by Arora et al. (Citation2014). Two distinct types of abnormalities were observed: (i) mitotic abnormalities, i.e. abnormalities in dividing cells; and (ii) interphase nuclear abnormalities. The varying ratio of ammonium and nitrate with respect to time has been considered as a possible reason for such variations in nuclear behavior.
Urea exhibited a direct mutagenic effect on post-meiotic stages, whereas ammonium nitrate and calcium nitrate revealed an indirect mutagenic effect on meiotic and pre-meiotic stages in Drosophila melanogaster, as reported by El-Din et al. (Citation2009). This study showed that the tested nitrogenous fertilizers possesses mutagenic potentialities which are related to their nitrogen content, as also reported by Arora et al. (Citation2014) with AT.
Anitha and Mahalkshmi (Citation2013) investigated the genotoxic effect of seaweed liquid fertilizer at different concentrations. The low concentrations had no negative effect on the MI, though abnormalities in the nucleus and chromosome were seen. Higher concentration induced abnormalities like multiple vacuolation, condensation of cytoplasm and MN formation. The growth and development of the crop plants was also affected.
Antofie and Doroftei (Citation2013) observed a strong decrease in the MI which became more intense with concentration and time of exposure to sodium nitrate. The appearance of genotoxic effects such as chromosomal alterations, highly condensed chromatin expression easily identified during mitosis stages, sticky chromosomes and chromosomal bridges and laggards was also reported. Doroftei and Antofie (Citation2013) tested the effects of sulfates in Allium root tip cells. On treatment with sodium sulfate, a strong decrease in the MI was observed, which became more intense with the time of exposure. The appearance of different chromosomal complement alterations including the appearance of highly condensed chromatin was also reported.
The cytotoxic effects and the mutagen potential of Bionat, a foliar fertilizer used for higher plants, was analyzed using Allium cepa. The cytogenetic abnormalities were assessed by calculating the MI and by analyzing the chromosomal aberrations. The results indicated, on the one hand, that Bionat at a higher concentration than that usually used in agriculture significantly increased the abnormal cell frequency at all concentrations and treatment periods when compared with controls, and this increase was dose-dependent. It also significantly decreased the MI in all the treatments when compared to their controls (Doroftei and Trandafirescu Citation2013).
Cytological changes in plants associated with application of fertilizers in soil have not been studied in detail. Most initial studies on organic manures like compost, cow-dung and oil cakes, used in various agricultural practices were focused on the role of decaying organic substances in increasing the frequency of natural spontaneous mutations. The observations showed that fertilizers like ammonium sulfate, ammonium phosphate, ammonium nitrate and potassium chloride produced chromosome breakage and other abnormalities during cell division in Allium cepa (Abraham Citation1965). Studies using calcium salt in Allium cepa showed that calcium ion concentration in cells played important roles in bringing about chromosome breakage, stickiness and other abnormalities (Misra Citation1982).
Artificial fertilizers, a major source of environmental nitrates, may be composed of a variety of chemicals including ammonium, calcium, potassium and sodium nitrates and urea. Nitrites can react with amines and amides to form nitrosamines and nitrosamides. The precursors of these N-nitroso compounds are widely distributed in various environmental media. N-nitroso compounds are carcinogenic in a wide range of animal species; most are mutagenic in test systems and some have been shown to be teratogenic to animals. Hazards can result as malignancies in living populations or may represent a genetic load to future generations (Adel Citation2000; Xu et al. Citation2003).
The assessment of genotoxic effects of the two most commonly used nitrogen (N) fertilizers, ammonium nitrate and urea, used Allium cepa L. (Verma et al. Citation2016). Ammonium nitrate and urea were added to soil and roots from onion bulbs grown in the fertilized soil. The roots were tested during the first and second week after treatment. Cytological analysis revealed fewer dividing cells and different types of dividing cell and interphase nuclear anomalies. Decrease in MI and increase in anomalies in dividing cells were observed in both fertilizers but aberrations were significantly higher in ammonium nitrate treated root tip cells.
A significant decrease in MI and increase in abnormality percentage compared to the control was observed with increasing duration of the ammonium nitrate treatment (Verma and Srivastava Citation2017). Chromosomal aberrations like stickiness, fragmentation, precocious movement, bridges and disorientations were observed in varying frequencies. Interphase cell volume of cells of treated roots and their respective interphase nuclear volume were reduced as compared to control. The ratio between nuclear and cytoplasmic volume has been reported to be related to cell integrity.
The Allium cepa roots were placed in contact with rice crop water to check for possible chromosomal MI abnormalities by Wandscheer et al. (Citation2017). The data obtained indicates that the application of the tricyclazole fungicide leads to an increase in the genotoxic activity in the rice crop water, through the appearance of chromosomal abnormalities, without, however, causing significant effects on the MI.
Studies on the genotoxicity of agrochemicals should be prioritized, as the analysis of chromosomal alterations serves as a reliable test and can be used directly as an estimate of damage in plant systems exposed to possible mutagens or carcinogens. The evaluation of the effects that harmful agents might cause requires a system in active mitotic division so that the changes taking place in cell cycle can be determined. The Allium cepa test fulfils this requirement, and so it is widely used for this purpose (Silva and Fonseca Citation2003).
MI is an indicator of cell proliferation (Gadano et al. Citation2002) and can be measured by the Allium cepa test system. Cytotoxicity tests using in vivo plant test systems, such as Allium cepa, have been validated by several researchers who performed animal testing in vitro and the results obtained are similar (Vicentini et al. Citation2001; Teixeira et al. Citation2003), providing valuable information for human health.
8. Effects of insecticides
Insecticides are used in agricultural areas to avoid losses resulting from insects. Farmers have faced crop management problems owing to loss induced by various insect pests since ancient times. Firstly, they perceived the negative effects of some natural compounds on various insect species and then used these products to protect their crops from insect pests (Oberemok et al. Citation2015). Later, farmers used natural chemicals such as inorganic sulfur, arsenics, cryolite and borax for this purpose (Popov et al. Citation2003). Plant protection chemicals such as Paris green and DDT were extensively used in the nineteenth and twentieth centuries (Alyokhin Citation2009). In the second half of the twentieth century, organophosphate and carbamate insecticides were widely used. The properties of pyrethrins as insecticides were ascertained after synthesis of allethrin in 1949. At the present time, the most popular insecticides are neonicotinoids, which are administered to the target parts of plant (Goulson Citation2013), and investigations for the discovery of new insecticides continues (Oberemok et al. Citation2015).
However, potential harmful effects of insecticides should be considered. The main problem arising from use of insecticides in agriculture is the environmental contamination caused by these agents or their by-products which can have a negative influence on ecosystems and human health (Crosby Citation1982; Bolle et al. Citation2004).
In some studies, insecticides reduced root lengths in plants and showed a detrimental effect on meristematic cells, which were seen to be unstructured, with a fragile cellular envelope, increased cell volume and in several cases rupture of the cellular membrane (Karaismailoğlu Citation2016; Citation2017; Karaismailoğlu and İnceer Citation2017). In addition, insecticides induced decrease of mitotic activity (Chauhan et al. Citation1999; İnceer et al. Citation2009; Karaismailoğlu Citation2016; Citation2017; De Souza et al. Citation2017b; Karaismailoğlu and İnceer Citation2017). Also, they caused chromosomal abnormalities by interfering with the formation of spindle fibers. Some authors reported the presence of MNs as a consequence of the use of insecticides (Karaismailoğlu Citation2017; Karaismailoğlu and İnceer Citation2017). Insecticides were reported to be genotoxic in rodent bone marrow (Agarwal et al. Citation1994), in peripheral lymphocyte cultures (Surralles et al. Citation1990) and in aquatic organisms (Campana et al. Citation1999).
In conclusion, common application of insecticides for the control of insects in agriculture may endanger the genetic material of economically important plants as well as other higher plants. The insecticides have to be safe and effective at the same time. For this reason, it is important to examine the genotoxic influences of insecticides on plants and other systems before considering their applications for agricultural goals. The AT appears to be optimal, since it measures directly the effect of chemicals on plants.
9. Cyto-genotoxicity of fungicides monitored by Allium root tip bioassay
Fungicides are biocidal chemical compounds (or biological organisms) used to kill parasitic fungi or their spores. Fungi can cause serious damage in agricultural crops, resulting in critical losses of yield, quality, and profit. Fungicides are used in agriculture and also to fight fungal infections in animals. On the basis of their chemical nature, we have copper fungicides (Bordeaux mixture, Blitox, and Cuprax); sulfur fungicides (Microsul, Thiram, Mancozeb, and Nabam) and mercury fungicides (Mersil, Agallol, and Agrosan).
The effect of the fungicide flusilazole on somatic cells of Allium cepa was evaluated by Ozakca and Silah (Citation2013). MI and different types of chromosomal abnormalities such as bridges, stickiness and laggards were observed. The ratio of necrotic cells significantly increased by flusilazole pre-soaking and RAPD-PCR revealed that the group treated with higher doses was more different to the control than those treated with lower doses.
The genotoxic potential of carbendazim was investigated by Selvaraju et al. (Citation2015) using CA in Allium cepa L. root tip cells. All concentrations used caused several abnormalities in mitotic cell division and the MI in the onion root tip cells decreased as the concentrations of carbendazim increased. The total percentage of aberrations generally increased in a dose and time dependent manner.
Biologists have raised questions about the hazards that could result from the usage of fungicides (Tomkins and Grant Citation1976). Residues of most agrochemicals, including fungicides, have been reported to be genotoxic to plants (Mishra and Sinha Citation1979). The cytological effects of different agrochemicals on several plant species have been studied by many researchers (Njagi and Gopalan Citation1981; Adam et al. Citation1990).
Fungicides are known to induce mutation (Kumar et al. Citation1977; Sahu et al. Citation1981) and many fungicides with their metabolic derivatives have been reported to be both carcinogenic and mutagenic (Kumar and Banerjee Citation2001; Chandra et al. Citation2002). Higher plants have been used as test organisms for studying the effects of genotoxic substances in the environment. Several plant test systems in use are found to be sensitive and reliable, but the Allium cepa root assay has been the most commonly used.
Devi et al. (Citation1991) analyzed the long-term effects of fungicides on both mitotic and meiotic systems in Allium cepa and concluded that they can induce chromosomal aberrations. Rost (Citation1977) reported that one-hour incubation of root tips with ioxynil resulted in a 50% reduction in the number of dividing cells and further decline was observed during a 24-hour incubation. The fungicides vitavax 200 and dithane S-60 at the rate of 2 g kg–1 seeds caused highly significant reduction in MI (Al-Najjar and Atef Citation1980).
Regular application of fungicides to crop plants has been reported to be the possible cause of genetic damage which could lead to chromosomal aberrations that have been cited as reliable indicators of mutagenic activity (Stoltz et al. Citation1970).
Topas 100EC is a widely employed foliar fungicide for horticultural applications. Its effects on cell cycle stages of the root cells of Allium cepa were investigated by Miron et al. (Citation2017). MI was found to decrease while abnormality percentage increased in comparison to a control. The aberrations increased with the treatment duration and the concentration of fungicide. Chromosomal aberrations like stickiness, condensation of chromosomes, c-mitosis and delay in spindle formation were seen immediately after 6-h treatments, highlighting the sensitivity and precision of the AT. Bridges, stickiness, vagrant chromosomes, fragments, c-anaphase and multi-polarity CAs were observed in anaphase-telophase cells. The total CAs were more frequent with increase in the treatment time and also the concentrations of Topas.
Cytotoxic effect of the fungicide Calixin was studied in the mitotic system of root tip meristem of Allium cepa L. by Pulate and Tarar (Citation2014). Seeds of Allium cepa were treated with different concentrations of Calixin for different durations. A large number of mitotic abnormalities, including lagging early anaphase, chromosomal bridges, c-metaphase, sticky metaphase, multipolarity, fragment, and vagrant were observed. They however differed in their relative numbers at different concentrations and durations of treatment.
The effects of a systemic fungicide mefenoxam and copper hydroxide (the active ingredients of Ridomil Gold Plus 42, 5 WP fungicide) were investigated in root tip cells of Allium cepa L. (Sutan et al. Citation2015). A progressive concentration- and duration-dependent inhibition of the mitotic activity was observed in meristematic cells. The MI was minimum at the highest concentration of the fungicide tested. The genotoxicity was estimated on the basis of chromosomal aberration frequency and the highest percentage of abnormal cells were observed at the lowest concentration of Ridomil used. Occurrence of sticky chromosomes, laggard and multipolarity in large numbers was suggestive of abnormal DNA condensation, abnormal chromosome coiling and inactivation of the spindles. This indicated the aneugenic potential of the fungicide.
Generally, the concentration of fungicide tested induced a dose-dependent inhibition of MI, which could be due to intracellular stress, including DNA damage, preventing the cells from entering mitosis. Mitodepressive action may be due to a negative interference of the active substances contained by the fungicide tested, with specific proteins and enzymes that influence DNA polymerase (Hidalgo et al. Citation1989), DNA synthesis, microtubule formation, impaired nucleoprotein synthesis and reduced level of ATP provide energy for spindle elongation, microtubule dynamics and chromosomal movement (Majewska et al. Citation2003; Türkoğlu Citation2012). Mefenoxam is specially formulated to provide systemic control of plant diseases caused by members of the Oomycete class of fungi. It effectively controls root and stem diseases caused by Pythium and Phytophthora spp. as well as foliar diseases such as downy mildew.
Dithane M-45 is a very well-known multisite fungicide, which belongs to the ethylene bisdithiocarbamates group. The active ingredient of fungicide tested is 80% mancozeb, as a coordination product of zinc ion and manganese ethylene bisdithiocarbamate. Mancozeb is a broad spectrum contact fungicide with protectant activity against a wide range of plant diseases. Similar results were obtained when assessing the cyto-genotoxic potential of the fungicide Dithane M-45 in Tulipa praestans (Şuţan et al. Citation2015). Using a different formula of the pesticide Dithane, Asita Okorie and Makhalemele (Citation2009) found an inhibitory effect on mitotic division, which could be the consequence of different concentrations tested.
There are several studies aiming to explain and to understand the effects of fungicides in plant systems. Rayburn et al. (Citation1993) stated that amount of nuclear DNA is decreased by the fungicide captan and this fungicide has been seen to be mutagenic, carcinogenic and teratogenic effects on many organisms.
The extensive use of fungicides in plant protection against fungal disease generates long term residues in food and in the environment (Petit et al. Citation2008). Fungicides may also influence plant genetic systems due to their mutagenicity and carcinogenicity. Regular use of these chemicals can result in altered hereditary constitution of an organism (Wuu and Grant Citation1967, Citation1982).
The effects of benomyl, a systemic fungicide, were investigated in the mitotic cell division in onion (Allium cepa) root tip cells during germination (Dane and Dalgiç Citation2005). Different concentrations of benomyl solutions were used and all the concentrations caused several abnormalities in mitotic cell divisions. The mitotic frequency in the onion root tip cells decreased as the concentration of benomyl solution increased.
The genotoxic effects of fungicide Raxil and the active substance tebuconazole in both mitotic and meiotic cell divisions of Allium cepa L. were studied. All concentrations and treatment periods of Raxil induced a number of chromosomal aberrations in root tip cells and in anthers of Allium cepa L. Additionally Raxil decreased the frequency of MI and caused reduction in pollen fertility (Kaymak and Rasgele Citation2009).
The toxicity and genotoxicity of thiabendazole (TBZ) in its commercial formulation Foldan® was studied in A. cepa meristematic cells at concentrations ranging between 10 and 250 μg ml–1 by Andrioli and Mudry (Citation2011). They found that exposure to TBZ induced a significant increase in the frequency of anaphase-telophase chromosomal aberrations, MNs and binucleated cells at all the concentrations of TBZ assayed. The frequency of anaphase-telophase aberrations was observed to reach a plateau at the concentration which caused mitotic toxicity. An increase was also observed in the rate of metaphase and anaphase, which was, in turn, indicative of alterations in chromatid segregation. The study revealed that exposure to TBZ caused toxicity and genotoxicity, both being consistent with mechanisms that interfere with microtubule formation. Allium cepa root tips grown in soil samples treated with different concentrations of carbendazim showed decreased MI and various chromosomal abnormalities (Verma and Srivastava Citation2018). Carbendazim is a broad spectrum, systemic, pre-emergent, widely used fungicide. It is usually applied to control plant diseases including soil-borne diseases to ensure high yield of crops and post-harvest food storage. All the concentrations of CBZ caused different chromosomal abnormalities mostly during metaphase (stray, clumped, disoriented, laggard, sticky chromosomes and C-metaphase), anaphase (multiple bridges, disoriented, laggard, precocious, fragmented, and multipolar anaphase), and telophase (bridge, disoriented, and lateral telophase) stages and some binucleate cells. Similar results were obtained by Fatma et al. (Citation2018) when the fungicide mancozeb was tested using the Allium assay.
10. Heavy metals effects
The results of previous investigations indicate that 11 different metals (Al, Cr, Pb, Cu, Mg, Co, Zi, Mn, Ni, Cd, Hg) at excessive concentration can disturb cell division process and induce CA comprising c-mitosis and lagging chromosomes, anaphase bridges, and chromosome stickiness in the root tips of A. cepa (Liu et al. Citation1995).
During mitosis, metal ions can interfere with the proper positioning of nucleolar organizing regions on chromosomes. Under metal stress, an obviously toxic phenomenon appears in nucleoli of root tips of A. cepa. These metals can disturb the nucleolar cycle with the extrusion of silver-stained materials containing argyrophilic proteins from the nucleolus into the cytoplasm. Indirect immunofluorescence detects nucleolar material containing nucleophosmin, nucleolin and fibrillarin and their movement into the cytoplasm following metal stress. Western blotting reveals higher expression of these three major nucleoproteins in the treated roots, which is consistent with the results obtained by indirect immunofluorescence (Liu et al. Citation2016). However, the mechanism needs to be further studied.
Electron energy loss spectroscopy (EELS) and electron spectroscopic imaging (ESI) are good methods for identifying sites of localization of heavy metals at the sub-cellular level in cell organelles, cytoplasm or cell walls and clarifying the process involved in their uptake, transport and deposition or detoxification in plant cells. Evidence demonstrates that heavy metals such as Cr, Cu, Fe, Pb, Cd and Ni are localized in electron dense granules in root tip cell vacuoles, small cytoplasmic vesicles, nucleoli and cell walls (except for Cd) under heavy metal stress (e.g. Liu and Kottke Citation2003, Citation2004).
11. Toxic plants
The AT can be applied to nearly the entire range of agrochemicals, but it is especially relevant for testing extracts of plants containing toxic substances that may be dangerous to human and animal health, especially since some of these plants are grown in many households or live as spontaneous flora in the vicinity of human communities. From this point of view, there are many decorative plants, invasive plants or even medicinal plants that can become true poisons by consuming certain vegetative parts or by inhaling their pollen.
In the following paragraphs some of the most common plants, known to be potentially toxic to humans and animals are presented along with the suitability of AT for evaluating them.
Nerium oleander is one of the most poisonous plants grown in households. The plant grows in rich bushes and has very showy flowers. Leaves, seeds and pollen of its flowers are very toxic and can cause intestinal problems, heart complications, cerebral attacks, coma and even death (Khan et al. Citation2010). The mitodepressive action of glycosides from Nerium oleander L. on root tips of Allium cepa L. was observed in 1971 (Tarkowska Citation1971).
Grayanotoxin, also known as rhodotoxin, is a toxin derived from the leaves, twigs or flowers of plants belonging to genus Rhododendron, many species of which are common decorative plants. The toxic component can be found in very high concentrations in honey made by bees that feed on pollen of Rhododendron spp. The toxin is also present in a labrador tea, cigarettes and a variety of decoctions used in alternative medicine (Jansen et al. Citation2012).
Ambrosia spp. is invasive outside North America that occurs spontaneously on the edges of roads, as a ruderal species, and on the banks of the plain rivers. Ambrosia is the cause of many allergies because its pollen contains an enzyme that can affect the integrity of the nasal mucosa and the respiratory tract in general.
Different species of Ambrosia have some inhibitory effects on other plant crops, as revealed by their effect on seed germination and seedling growth of Zea mays (Bonea et al. Citation2018), length of the roots of Helianthus annuus (Buzhdygan and Baglei Citation2016) and the growth and root hair development of Triticum aestivum (Kong et al. Citation2007).
Although Nicotiana tabacum is less toxic to plants than some chemicals, industrial effluents, radiation, etc., tobacco leaf extract can induce a decrease in MI and increase of mitotic abnormalities in Allium cepa root tip cells treated with different concentrations (2, 3 and 4 g*l-1) of this extract (Banarjee and Sharma Citation1979). So tobacco fresh leaf should be regarded as a toxic and mutagenic agent. The cytotoxicity level can be determined by the decreased rate of MI (Kalpana et al. Citation2014).
Use of medicinal plants in pursuits of health is fashionable, but use of crude extracts can be toxic. For example, by using the AT, Saggoo et al. (Citation1991) concluded that the leaf homogenate of Tylophora indica L. is cytotoxic. For this reason, the authors recommended that high-dose use of this medicinal plant should be avoided.
12. Ionizing radiation and radioactive elements
AT is frequently used to evaluate cytotoxic and genotoxic effects, such as CAs, MNs and disturbance in the mitotic cycle. AT was also useful for assessing effects of water and soil samples from radiation polluted areas.
One of the earlier works by Sax (Citation1941) demonstrated sensitivity of Allium cepa to ionizing radiation and showed that X-rays are capable of inducing a large number of chromosomal mutations in the cells of root tips of Allium cepa L.
AT was used to evaluate genotoxicity of soil samples collected from four zones around Chernobyl Nuclear Power Plant with different level of radioactive contamination with radionuclides (cesium-137, strontium-90, kalium-40, thorium-232). Significant correlation of cesium-137 activity in soil samples with the level of chromosomal aberrations and MI was found. The authors concluded that AT was an effective, quick and inexpensive biological test for ecological and genetic risk assessment in the Chernobyl zones and may be a useful alternative to animal test for estimation of genotoxic effects of radioactively polluted soils (Kovalchuk et al. Citation1998).
Grodzinsky et al. (Citation2006) showed, by using AT and other plant systems, that the ionizing-radiation in the 30-km Chernobyl exclusion zone (contaminated by different radionuclides: caesium-137, caesium-134, iodine-131, strontium-90, cerium-144, ruthenium-106, etc.) increased levels of chromosomal aberrations and MNs. The level of genotoxic effects in AT is correlated with the density of radioactive contamination of the territory (Kovalchuk et al. Citation1998; Grodzinsky et al. Citation2006).
In laboratory experiments, alpha-radiation from plutonium-239 significantly increased the frequency of mitotic disturbances (lagging chromosomes and chromosome stickiness) as well as CAs (bridges and fragments), nuclear buds and MNs. Alpha-radiation from plutonium-239 showed more pronounced clastogenic activity due to the increase in CAs (total), fragments, and MNs in the root meristem of A. cepa (Pesnya and Romanovsky Citation2013). Plutonium-239 ionizing alpha-radiation also increased MI and root length. Stimulation of plant growth by low and moderate doses of ionizing radiation previously was described by different scientists and sometimes referred to “effect of radiation hormesis”. Stimulation of mitosis and growth of plant shoots by ionizing radiation have been shown against a background of increased levels of genetic disturbances. When MI is significantly higher than the control level it can be harmful for the cells, leading to a disordered cell proliferation and even to malignant transformation (Timofeeff-Ressovsky and Luchnik Citation1958; Marciulioniene et al. Citation2007; Shestopalova and Baeva Citation2007).
AT was recommended as an effective tool for genotoxicity testing in case of combined contamination of environmental compartments with radionuclides and chemical compounds (Oudalova et al. Citation2017).
In another investigation, the Allium root test (without ana-telophase or MN assay) was used to access toxicity of bottom sediments (containing caesium-137) in the Yenisei River and the effect of external gamma-radiation (absorbed dose was 0.015 Gy) under laboratory conditions. In experiments with sediment samples stimulation of root growth was found and a slight increase in root length was registered in an experiment with an external gamma-radiation source (Bolsunovsky et al. Citation2016). In another study it was shown that gamma-rays at higher doses (20, 40 and 60 Gy) decreased MI in Allium cepa root meristematic cells and increased the number of MNs, chromosomal aberration and abnormalities as well as levels of MDA and antioxidant enzymes. A positive correlation between chromosomal aberrations and antioxidant enzymes related defense mechanism of cell has been established (Kumar et al. Citation2011).
Evseeva et al. (Citation2003) used a modification of AT (Allium schoenoprasum L. meristematic root tip cells) to investigate the genotoxic effects of water samples contaminated by a combination of radioactive elements (radium-226, thorium-232, uranium-238, polonium-210, plumbum-210) and non-radioactive metal ions (Zn, Mn and Ca). A significant increase in the number of chromosomal aberrations was found. The MI was found to decrease in parallel with uranium-238 concentration increase, thus showing that MI is good toxicity indicator. The authors suggested the combined use of chemical composition determination and AT to identify the major sources of environmental risk (Evseeva et al. Citation2003).
AT was used for investigation of genotoxic effects of water samples contaminated with strontium-90 from the territory of the regional radioactive waste storage of I.I. Leypunsky Institute of Physics and Power Engineering (ROSATOM). A considerable increase in amount of chromosomal aberrations (bridges and fragments) was registered, while the level of mitotic abnormalities (lagging chromosomes) increased only slightly. To determine sensitivity of AT, the authors also carried out a laboratory experiment with different doses of gamma-radiation (0.1, 0.2, 0.9 and 2 Gy), and registered a stimulating effect of low doses (0.1–0.2 Gy) on cell proliferation, while at a higher dose (2 Gy), a depression of mitotic activity was observed (Sinovets et al. Citation2009).
Firbas and Amon (Citation2014) noted that both ana-telophase and metaphase assays are suitable for the detection of genotoxic effects of ionizing radiation.
Overall, AT is proved to be a very convenient, highly sensitive and informative cytogenetic tool for rapid screening of ionizing radiation and radionuclide pollution.
13. Conclusions and perspectives
13.1. Standardizing the starting material
Intraspecific variation in A. cepa has been until now overlooked, as in most investigations no mention is made about the characterization of the onion cultivar used in cytotoxicological testing, hence we may suppose that there is no rule about the choice of the material. A standardization of the material used in all studies would allow a better generalization of the results, since we cannot be absolutely sure that all cultivars will give the same answer if subjected to a given genotoxic agent.
A first recommendation is that data about the cultivars used are provided in studies; alternatively that the same cultivar is used in all investigations. The latter may not be an easy task, since in different countries the most common onion cultivars may be different. A quite well known cultivar, first produced in Italy but widely exported in the whole world is A. cepa “red Tropea onion” or “rossa di Tropea” and it may be a possible choice, since its three ecotypes were discriminated with an electronic-nose system (Russo et al. Citation2013).
13.2. TEM investigation
A more frequent use of TEM investigation, even if time consuming and not available in all labs, may help to better understand the mechanism of cytotoxicity, since many morphological characters used in data collection may be morphologically similar but arising from very different processes and may be also morphologically distinct at the ultrastructural level. Moreover, some data can be observed only with TEM.
13.3. Improving statistics
Tests other than chi-squared may be used in case of reduced amount of data. Tests should be used also to assess the minimal sample dimension for obtaining significance, since data collection (microscope observation) appears to be the bottle neck of the Allium cepa test.
13.4. Testing of nanovector toxicity
Lipid nanovectors, such as liposomes, lipid-based nanoparticles and lipid nanoemulsions, are used as drug delivery systems, thus opening interesting perspectives in the field of nano-medicine. Their lipidic structure guarantees biocompatibility and easy interaction with the cell membrane, which mainly consists of lipids and phospholipids (Xue et al. Citation2014). The use of cationic lipids included in lipid nanoformulations is very common. This gives advantages in terms of prolonged circulation time and improved encapsulation efficacy in case of negatively charged therapeutics, such as nucleic acids (Yi Xue et al. Citation2015). On the other hand, the employment of cationic lipids can lead to cytoplasm vacuolization, a reduction in the number of mitosis, cell shrinkage (Lappalainen et al. Citation1994) and, at high concentrations, cell lysis and necrotic death (Xue et al. Citation2014). In this context AT could represent an interesting tool to evaluate the toxicity of cationic lipid-based nano-systems, especially for the use of lipid formulations in the treatment of plants.
Disclosure statement
No potential conflict of interest was reported by the authors.
Additional information
Funding
References
- Abraham S. 1965. Studies on spontaneous and induced mutations in plants. Cytologia. 30:155–172.
- Adam ZM, Ebad FA, Abo-Fl-Kheir ZA, Shaikh E. 1990. Alterations in nucleic acids, protein content and mitotic division of Vicia faba root tip cells as affected by malathion and tamaron insecticides. Cytologia. 55:349–355.
- Adel EI 2000. The mutagenic effects of some nitrogenous fertilizers in Drosophila melanogaster. Ph.D. Thesis. Entomology Department, Faculty of Science, Cairo University.
- Agarwal DK, Chauhan LKS, Gupta SK, Sundararaman V. 1994. Cytogenetic effects of deltamethrin on rat bone marrow. Mutat Res. 311:133–138.
- Algarni AA. 2018. Genotoxic effects of acrylamide in mouse bone marrow cells. Caryologia. 71(2):160–165.
- Al-Najjar NR, Atef SS. 1980. Cytological effects of fungicides I. Mitotic effects of Vitavax-200 and Dithane S-60 on wheat and two related species. Cytologia. 45(1/2):163–168.
- Alyokhin A. 2009. Colorado potato beetle management on potatoes: current challenges and future prospects. Fruit Veg Cereal Sci Biotech. 3(1):10–19.
- Andrioli NB, Mudry MD. 2011. Cytological and cytogenetic effects induced by thiabendazole on Allium cepa root meristems. J Basic Appl Genet. 22(2):2.
- Anitha R, Mahalakshmi K. 2013. Genotoxic effect of sea weed liquid fertilizer on Allium cepa L. Applied Cell Biology. 2(2):93–96.
- Antofie -M-M, Doroftei E. 2013. Genotoxic effects of sodium nitrate in onion roots. Analele Universitatii Din Oradea, Fascicula Biologie. 20(2):71–79.
- Arora K, Singh N, Srivastava S, Srivastava A. 2014. Evaluation of genotoxic risks due to temporal changes in soil urea: using Allium cepa L. root tip bioassay. Cytologia. 79(1):85–93.
- Asita Okorie A, Makhalemele R. 2009. Genotoxic effects of dithane, malathion and garden ripcord on onion root tip cells. Afr J Food Agric Nutr Dev. 9(4):1191–1209.
- Asita Okorie A, Moramang S, Rants’o T, Magama S. 2017. Modulation of mutagen-induced genotoxicity by vitamin C and medicinal plants in Allium cepa L. Caryologia. 70(2):151–165.
- Ateeq B, Farah MA, Ali MN, Ahmad W. 2002. Clastogenicity of pentachlorophenol, 2,4-D and butachlor evaluated by Allium root tip test. Mut Res. 514:105–113.
- Banarjee A, Sharma A. 1979. Effects of tobacco extraction on Allium cepa chromosomes. Persp in Cytolog and Gen. Hindasia, New Delhi. 3(1):633–637.
- Barbério A, Voltolini JC, Mello MLS. 2011. Standardization of bulb and root sample sizes for the Allium cepa test. Ecotoxicology. 20:927–935.
- Bhatta P, Sakya SR. 2008. Study of mitotic activity and chromosomal behaviour in root meristem of Allium cepa L. treated with magnesium sulphate. Ecoprint. 15:83–88.
- Bolle P, Mastrangelo S, Tucci P, Evandri MG. 2004. Clastogenicity of atrazine assessed with the Allium cepa test. Environ Mol Mutagen. 43(2):137–141.
- Bolsunovsky AY, Trofimova EA, Zueva AV, Dementiev DV. 2016. The first results of using the Allium test in estimating the chemical and radiation toxicity of bottom sediments in the Yenisei River. Doklady Biol Sci. 469(1):192–195.
- Bonea D, Bonciu E. 2017. Cytogenetic effects induced by the fungicide Royal Flo to maize (Zea mays L.). Caryologia. 70(3):195–199.
- Bonea D, Bonciu E, Niculescu M, Olaru AL. 2018. The allelopathic, cytotoxic and genotoxic effect of Ambrosia artemisiifolia on the germination and root meristems of Zea mays. Caryologia. 71(1):24–28.
- Buzhdygan OY, Baglei OV. 2016. Developmental traits in grassland and agricultural plants under the influence of ragweed. Biol Syst. 8(2):202–207.
- Campana MA, Panzeri AM, Moreno VJ, Dulout FN. 1999. Genotoxic evaluation of the pyrethroid lambdacyhalothrin using the micronucleus test in erythrocytes of fish Cheirodon interruptus. Mut Res. 438:155–161.
- Casida JE, Bryant RJ. 2017. The ABCs of pesticide toxicology: amounts, biology, and chemistry. Toxicol Res. 6(6):755–763.
- Chandra BR, Saharan RP, Sareen PK. 2002. Clastogenic effects of trifluralin in Vicia faba. J Cytol Genet. 26:13–18.
- Chauhan LKS, Saxena PN, Gupta SK. 1999. Cytogenetic effects of cypermethrin and fenvalerate on the root meristem cells of Allium cepa. Environ Exp Bot. 42(3):181–189.
- Chaurasia OP, Sinha SP. 1987. Effects of urea on mitotic chromosomes of mice and onion. Cytologia. 52:877–882.
- Croker BH. 1953. Effects of 2,4-dichlorophenoxyacetic acid and 2,4,5-trichlorophenoxyacetic acid on mitosis in Allium cepa. Bot Gaz. 114:274:283.
- Crosby DG. 1982. Pesticides as environmental mutagens. In: Fleck RA, Hollander A, editors. Genetic toxicology: an agricultural perspective. London: Plenum Press. New York; p. 201–218.
- D’Amato F. 1950. The quantitative study of mitotic poisons by the Allium cepa test: data and problems. Protoplasma. 39(4):423–433.
- Dane F, Dalgiç O. 2005. The effects of fungicide benomyl (benlate) on growth and mitosis in onion (Allium cepa L.) root apical meristems. Acta Biol Hung. 56(1–2):119–128.
- Daphedar A, Taranath TC. 2018. Green synthesis of zinc nanoparticles using leaf extract of Albizia saman (Jacq.) Merr. and their effect on root meristems of Drimia indica (Roxb.) Jessop. Caryologia. 71(2):93–102.
- Dávila Giraldo LR, Méndez Arteaga JJ, Arango WM. 2018. Cytotoxic activity of ethanolic extracts of a selection of macromycetes. Caryologia. 71(2):166–173.
- De Souza CP, Guedes TA, Fontanetti CS. 2016. Evaluation of herbicides action on plant bioindicators by genetic biomarkers: a review. Environ Monit Assess. 188:694.
- de Souza CP, Souza RB, Palmieri MJ, Andrade-Vieira LF, Fontanetti CS. 2017a. Aneugenicity and DNA fragmentation in Allium cepa induced by a commercial formulation of 2,4-D herbicide. In: Dorta, DJ, editor. Abstract book of XX Brazilian congress of toxicology “21st century toxicology strategies for a safer and more sustainable world”. (Vol. 2) October 8-11; Goiânia. Brazil: Appl Res Toxicol; p. 65.
- de Souza RP, de Souza CP, Bueno OC, Fontanetti CS. 2017b. Genotoxicity evaluation of two metallic-insecticides using Allium cepa and Tradescantia pallida: a new alternative against leaf-cutting ants. Chemosphere. 168:1093–1099.
- Devi P, Kiranmai V, Padmavathi T. 1991. Pesticide induced cytological abnormalities in Allium cepa L. J Cytol Genet. 26:13–18.
- Doroftei E, Antofie -M-M. 2013. The cyto- and genotoxic effects induced by sulphates in Allium cepa L. An Univ Oradea Fasc Biol. 20(2):64–70.
- Doroftei E, Trandafirescu M. 2013. Cytotoxic effects and mutagen potential induced by the foliar fertiliser bionat on Allium cepa L. J Environ Prot Ecol. 14:1627–1636.
- Dutta J, Ahmad A, Singh J. 2018. Study of industrial effluents induced genotoxicity on Allium cepa L. Caryologia. 71(2):139–145.
- El-Din AE, Abd-El-Samie EM, Faheem HB, Ibrahim MT, Ramzy A, Salama MS. 2009. Mutagenic effect of three nitrogenous fertilizers on Drosophila melanogaster. Cytologia. 74(2):201–208.
- Eroğlu HE. 2015. Which chromosomes are subtelocentric or acrocentric? A new karyotype symmetry/asymmetry index. Caryologia. 68(3):239–245.
- Evseeva TI, Geras’kin SA, Shuktomova II. 2003. Genotoxicity and toxicity assay of water sampled from a radium production industry storage cell territory by means of Allium test. J Environ Radioact. 68:235–248.
- Fatma F, Verma S, Kamal A, Srivastava A. 2018. Monitoring of morphotoxic, cytotoxic and genotoxic potential of mancozeb using Allium assay. Chemosphere. 195:864–870.
- Fernandes TCC, Mazzeo EC, Marin-Morales MA. 2007. Mechanism of micronuclei formation in polyploidizated cells of Allium cepa exposed to trifluralin herbicide, Pest. Biochem Physiol. 88:252–259.
- Firbas P. 2015. A survey of Allium cepa L. chromosome damage in Slovenian environmental water, soil, and rainfall samples. Int J Curr Res Biosci Plant Biol. 2(1):62–83.
- Firbas P, Amon T. 2014. Chromosome damage studies in the onion plant Allium cepa L. Caryologia. 67(1):25–35.
- Firbas P, Amon T. 2017. Combined of chemical analysis, fish micronuclei and onion chromosome damage for assessing cleaning effect in the WWTP central Domžale-Kamnik and quality of Kamniška Bistrica River. Cepal Review. 121:2825–2842.
- Fiskesjö G. 1985. The Allium test as a standard in environmental monitoring. Hereditas. 102(1):99–112.
- Fitak B, Grabowski M, Suchocki P 1999. Polski Patent. Pol. Pl 176530 (Cl. A61K31/095), 30 June 1999, Appl. 304046, 29 June 1994; p. 6.
- Friesen N, Fritsch RM, Blattner FR. 2006. Phylogeny and new intrageneric classification of Allium (Alliaceae) based on nuclear ribosomal DNA ITS Sequences. Aliso. 22(1):372–395.
- Gadano A, Gurni A, López P, Ferraro G, Carballo M. 2002. In vitro genotoxic evaluation of the medicinal plant Chenopodium ambrosioides L. J Ethonopharmacol. 81:11–16.
- Goulson D. 2013. Review: an overview of the environmental risks posed by neonicotinoid insecticides. J Appl Ecol. 50(4):977–987.
- Grodzinsky DM, Konoplya EF, Vakulovsky SM 2006. International conference «Chernobyl 20 years later, Minsk, 19-21 April 2006»: 89–96. (in Russian)
- Hidalgo A, Gonzalez-Reyes JA, Navas P, Garcia-Herdugo G. 1989. Abnormal mitosis and growth inhibition in Allium cepa roots induced by propham and chlorpropham. Cytobios. 57:7–14.
- İnceer H, Hayirlioglu-Ayaz S, Ozcan M. 2009. Genotoxic effects of the insecticide cypermethrin on the root meristem cells of sunflowers (Helianthus annuus L.). Bull Environ Contam Toxicol. 83(5):652–656.
- Jansen SA, Kleerekooper I, Hofman ZLM, Kappen IFPM, Stary-Weinzinger A, Van der Heyden MAG. 2012. Grayanotoxin poisoning: ‘Mad honey disease’ and beyond. Cardiovasc Toxicol. 12(3):208–215.
- Kalpana B, Lakshmi S, Karthikeyan S. 2014. Effect of fresh leaf extract of tobacco on the somatic chromosome of Allium cepa Linn. Int J Cur Tr Res. 3(2):109–112.
- Karaismailoğlu MC. 2016. The evaluation of the genotoxic and cytotoxic effects of pyriproxyfen insecticide on Allium cepa somatic chromosomes with mitotic activity, chromosome abnormality and micronucleus frequency. Turk J Life Sci. 1(2):65–69.
- Karaismailoğlu MC. 2017. Assessments on the potential genotoxic effects of fipronil insecticide on Allium cepa somatic cells. Caryologia. 70(4):378–384.
- Karaismailoğlu MC, İnceer H. 2017. Evaluation of potential genotoxic and cytotoxic effects of deltamethrin insecticide on somatic chromosomes of Helianthus annuus L. Caryologia. 70(4):295–301.
- Kaymak F, Goc Rasgele P. 2009. Genotoxic effects of raxil on root tips and anthers of Allium cepa L. Caryologia. 62(1):1–9.
- Khan I, Kant C, Sanwaria A, Meena L. 2010. Acute cardiac toxicity of Nerium oleander/indicum poisoning (Kaner) poisoning. Heart Views. 11(3):115–116.
- Kim S, Park YI, Yang TJ. 2015. Characterization of three active transposable elements recently inserted in three independent DFR-A alleles and one high-copy DNA transposon isolated from the Pink allele of the ANS gene in onion (Allium cepa L.). Mol Genet Genom. 290:1027–1103.
- Kong CH, Wang P, Xu XH. 2007. Allelopathic interference of Ambrosia trifida with Wheat (Triticum aestivum). Agric Ecosyst Environ. 119(3):416–420.
- Kovalchuk O, Kovalchuk I, Arkhıpov A, Telyuk P, Hohn B, Kovalchuk L. 1998. The Allium cepa chromosome aberration test reliably measures genotoxicity of soils of inhabited areas in the Ukraine contaminated by the chernobyl accident. Mutat Res. 415:47–57.
- Krishnamurthy KV, Krishnaraj R, Chozhavendan R, Samuel CF. 2000. The programme of cell death in plants and animals – a comparison. Curr Sci. 79(9):1169–1181.
- Kumar CV, Ramiah NJ, Subadra J. 1977. Cytological effects of fungicide Nystatin in Allium cepa. Indian J Exp Biol. 15:1071–1072.
- Kumar DS, Chakrabarty D, Verma AK, Banerji BK. 2011. Gamma ray induced chromosomal aberrations and enzyme related defence mechanism in Allium cepa L. Caryologia. 64(4):388–397.
- Kumar S, Banerjee MK. 2001. Evidence for three paracentric inversions between Welsh and Common onion. J Cytol Genet. 34:173–182.
- Kurás L. 2004. Characterization of protein–DNA association in vivo by chromatin immunoprecipitation. In: Dickson RC, Mendenhall MD, editors. Signal transduction protocols, methods in molecular biology (Vol. 284). Totowa: Humana Press Inc.; p. 147–162.
- Kursheed S, Raina A, Amin Laskar R, Khan S. 2018. Effect of gamma radiation and EMS on mutation rate, their effectiveness and efficiency in faba bean (Vicia faba L.). Caryologia.
- Lappalainen K, Jääskeläinen I, Syrjänen K, Urtti A, Syrjänen S. 1994. Comparison of cell proliferation and toxicity assays using two cationic liposomes. Pharmaceutical Research. 11(8):1127–1131.
- Leme DM, Marin-Morales MA. 2009. Allium cepa test in environmental monitoring: A review on its application. Mutat Res. 682:71–81.
- Levan A. 1938. The effect of colchicine on root mitosis in Allium. Hereditas. 24:471–486.
- Li D, Huang Q, Lu M, Zhang L, Yang Z, Zong M, Tao L. 2015. The organophosphate insecticide chlorpyrifos confers its genotoxic effects by inducing DNA damage and cell apoptosis. Chemosphere. 135:387–393.
- Li -Q-Q, Zhou S-D, He X-J, Yu Y, Zhang Y-C, Wei X-Q. 2010. Phylogeny and biogeography of Allium (Amaryllidaceae: allieae) based on nuclear ribosomal internal transcribed spacer and chloroplast rps16 sequences, focusing on the inclusion of species endemic to China. Ann Bot. 106:709–733.
- Liu D, Jiang W, Wang W, Zhai L. 1995. Evaluation of metal ion toxicity on root tip cells by the Allium test. Israel J Plant Sci. 43:125–133.
- Liu D, Kottke I. 2003. Subcellular localization of chromium and nickel in root cells of Allium cepa by EELS and ESI. Cell Biol Toxicol. 19:299–311.
- Liu DH, Kottke I. 2004. Subcellular localization of cadmium in the root cells of Allium cepa by electron energy loss spectroscopy and cytochemistry. J Biosci. 29:329–335.
- Liu X, Shi Q, Zou J, Wang J, Wu H, Wang J, Jiang W, Liu D. 2016. Chromosome and nucleolus morphological characteristics in root tip cells of plants under metal stress. Fresenius Environ Bull. 25:2419–2426.
- Ma TH. 1999. The international program on plant Bioassays and the report of the follow-up study after the hands-on workshop in China. Mutat Res. 426:103–106.
- Ma TH, Cabrera GL, Owens E. 2005. Genotoxic agents detected by plant bioassays. Rev Environ Health. 20(1):1–14.
- Ma TH, Xu Z, Xu C, Mc Connell H, Rabago EV, Arreola GA, Zhang H. 1995. The improved Allium/Vicia root tip micronucleus assay for clastogenicity of environmental pollutants. Mutat Res. 334:185–195.
- Mahmood Q, Bilal M, Jan S. 2014. Herbicides, pesticides, and plant tolerance: an overview. In: Ahmad P, Rasool S, editors. Emerging technologies and management of crop stress tolerance. 1st. ed. San Diego: Academic Press; p. 423–448.
- Majewska A, Wolska E, Sliwinska E, Furmanowa M, Urbanska N, Pietrosuk A, Zobel A, Kuras M. 2003. Antimitotic effect, G2/M accumulation, chromosomal and ultrastructure changes in meristematic cells of Allium cepa L. root tips treated with the extract from Rhadiola rosea roots. Caryologia. 56(3):337–351.
- Marciulioniene D, Luksiene B, Kiponas D, Maksimov G, Darginaviciene J, Gaveliene V. 2007. Effects of 137Cs and 90Sr on the plant Lepidium sativum L. growth peculiarities. Ecologija. 53(1):65–70.
- Miron L, Beleniuc G, Doroftei E 2017. Cytogenetic effects of the commercial fungicide Topas 100EC on meristematic root cells of Allium cepa L. 17th International Multidisciplinary Scientific GeoConference SGEM 2017, Vienna GREEN Conference Proceedings. https://www.sgemviennagreen.org
- Mishra GM, Sinha SP. 1979. Effects of Malathion on mitotically dividing onion (Allium cepa) root tip cells. Indian Jour Expl Biol. 17:716–717.
- Misra MP. 1982. Effect of calcium salts on Allium cepa chromosomes. Cytologia. 47:47–51.
- Nabeel M, Abderrahman S, Papini A. 2008. Cytogenetic effect of Arum maculatum extract on the bone marrow cells of mice. Caryologia. 61(4):383–387.
- Njagi GDE, Gopalan HNB. 1981. Mutagenicity testing of herbicides, fungicides and insecticides. Chromosome aberrations in V. faba. Cytologia. 46:169–172.
- Oberemok VV, Laikova KV, Gninenko YI, Zaitsev AS, Nyadar PM, Adeyemi TA. 2015. A short history of insecticides. J Plant Prot Res. 55(3):221–226.
- OECD (Organisation for Economic Co-operation and Development). 2006. Current approaches in the statistical analysis of ecotoxicity data: a guidance to application. (Vol. 33). Paris, France: OECD Publishing; p. 1–147.
- Oudalova AA, Geras’kin SA, Dikareva NS, Pyatkova SV. 2017. Allium-test as a tool for toxicity testing of environmental radioactive-chemical mixtures. J Physics: Conf Ser. 784(1):1–5.
- Ozakca DU, Silah H. 2013. Genotoxicity effects of Flusilazole on the somatic cells of Allium cepa. Pestic Biochem Physiol. 107(1):38–43.
- Palmieri, M. J., Andrade-Vieira, L. F., Trento, M. V. C., Eleutério, M. W. F., Luber, J., Davide, L. C., et al. 2016. Cytogenotoxic effects of spent pot liner (SPL) and its main components on human leukocytes and meristematic cells of Allium cepa. Water Air Soil Pollut. 227:1-10.
- Papini A, Mosti S, Milocani E, Tani G, Di Falco P, Brighigna L. 2011. Megasporogenesis and programmed cell death in Tillandsia (Bromeliaceae). Protoplasma. 248:651–662.
- Pavlica M, Papes D, Nagy B. 1991. 2,4- Dichlorophenoxyacetic acid causes chromatin and chromosome abnormalities in plant cells and mutation in cultured mammalian cells. Mutat Res. 263(77):81.
- Pearson K. 1900. On the criterion that a given system of deviations from the probable in the case of a correlated system of variables is such that it can be reasonably supposed to have arisen from random sampling. Philosophical Magazine Series 5. 50:157–175.
- Peruzzi L, Carta A, Altinordu F. 2017. Chromosome diversity and evolution in Allium (Allioideae, Amaryllidaceae). Plant Biosyst. 151:212–220.
- Pesnya DS, Romanovsky AV. 2013. Comparison of cytotoxic and genotoxic effects of plutonium-239 alpha particles and mobile phone GSM 900 radiation in the Allium cepa test. Mutat Res. 750:27–33.
- Pesnya DS, Romanovsky AV, Serov DA, Poddubnaya NY. 2017. Genotoxic effects of Heracleum sosnowskyi in the Allium cepa test. Caryologia. 70(1):55–61.
- Petit AN, Fontaine F, Clément C, Vaillant-Gaveau N. 2008. Photosynthesis limitations of grapevine after treatment with the fungicide fludioxonil. J Agric Food Chem. 56(15):6761–6767.
- Popov S, Dorozhkina LA, Kalinin VA 2003. Fundamentals of chemical plant protection. Art-Lion, Moskva, pp. 208.
- Puizina J, Papea D. 1996. Cytogenetical evidences for hybrid structure and origin of diploid and triploid shallots (Allium cepa var. viviparum, Liliaceae) from Dalmatia (Croatia). Pl Syst Evol. 199:203–215.
- Pulate PV, Tarar JL. 2014. Cytogenetic effects of tilt on root tip meristem of onion Allium cepa L. IJPAES. 4(2):53–57.
- Rank J. 2003. The method of Allium anaphase-telophase chromosome aberration assay. Ekologija. 1:38–42.
- Rank J, Jensen AG, Skov B, Pedersen LH, Jensen K. 1993. Genotoxicity testing of the herbicide Roundup and its active ingredient glyphosate isopropylamine using the mouse bone marrow micronucleus test, Salmonella mutagenicity test, and Allium anaphase-telophase test. Mutat Res. 300:29–36.
- Rayburn LA, Wayne L, Pedersen L. 1993. The fungicide Dithane M-45 reduce nuclear DNA content in maize seedling. Pestic Sci. 37:79–82.
- Rieger R, Michaelis A, Green MM. 1991. Glossary of genetics – classical and molecular. 5th ed. Berlin Heidelberg: Springer-Verlag; p. 50.
- Rodrigues GS. 2002. Pesticide mutagenesis. In: Pimentel D, editor. Encyclopedia of pest management. New York: Marcel Dekker; p. 595–597.
- Rossi LF, Luacesa JP, Palermoc AM, Merania MS, Mudry MD. 2018. Cytogenetic damage in peripheral blood cultures of Chaetophractus villosus exposed in vivo to a glyphosate formulation (Roundup). Ecotoxicol Environ Saf. 157:121–127.
- Rost TL. 1977. Responses of the plant cell cycle to stress. In: Rost TL, Gifford EM, editors. Mechanisms and control of cell division. Dowden Hutchinson and Ross. Stroudsbourg, PA, USA; p. 111–143.
- Russo M, Di Sanzo R, Cefaly V, Carabetta S, Serra D, Fuda S. 2013. Non-destructive flavour evaluation of red onion (Allium cepa L.) ecotypes: an electronic-nose-based approach. Food Chem. 141:896–899.
- Saggoo MIS, Kumari S, Bindu. 1991. Cytological effects of indian medicinal plants I. Mitotic effects of leaf homogenate of Tylophora indica L. on Allium cepa. Cytologia. 56(4):633–637.
- Sahu RK, Behera BN, Shanna CBSR. 1981. Effects of a fungicide, Dexon and its derivative on root meristems. Nucleus. 24:60–65.
- Sax K. 1941. The behavior of X-ray induced chromosomal aberrations in Allium root tip cells. Genetics. 26(4):418–425.
- Selvaraju S, Vasanth M, Rajarajan R, Muralidharan R, Raghupathy V. 2015. Genotoxic effects of Carbendezim (fungicide) on the root apical meristems of Allium cepa L. Res J Pharmacognosy Phytochmistry. 7(1):29–33.
- Sharma CBSR, Panneerselvan N, Ma T-H. 1990. Genetic toxicology of pesticides in higher plant systems. Crit Rev Plant Sci. 9(5):409–442.
- Shestopalova NG, Baeva EY. 2007. Radioadaptive response of leaf meristem cells of inbred and heterosis Helianthus annuus L. plants during ontogenesis. Tsitol Genet. 41(6):44–49.
- Silva J, Fonseca MB. 2003. Estudos toxicológicos no ambiente e na saúde Humana. In: Silva J, Erdtmann B, Henriques JAP, editors. Organisation. Genética Toxicológica. Porto Alegre: Alcance; p. 69–84
- Silveira GL, Lima MGF, Dos Reis GB, Palmieri MJ, Andrade-Vieira LF. 2017. Toxic effects of environmental pollutants: comparative investigation using Allium cepa L. and Lactuca sativa L. Chemosphere. 178:359–367.
- Sinovets S, Pyatkova SV, Koz’min GV. 2009. Ecological bases of allium-test application for ecological monitoring. Izvestiya Vysshikh Uchebnykh Zavedenij. Yadernaya Ehnergetika. 1:32–38. in Russian.
- Ślusarczyk J, Dudek M, Wierzbicka M, Suchocki P, Kuraś M. 2014. Antimitotic effect of Selol and sodium selenate (IV) on Allium test cells. Caryologia. 67(3):250–259.
- Ślusarczyk J, Wierzbicka M, Suchocki P, Kuraś M. 2015. Ultrastructural changes in onion (Allium cepa L.) root tip meristem cells treated with Selol and sodium selenate (IV). Caryologia. 68(4):306–316.
- Sobrero MC, Ronco A. 2004. Ensayo de toxicidad aguda con semillas de lechuga (Lactuca sativa L.). In: Castillo G, editor. Ensayos toxicológicos y métodos de evaluación de calidad de aguas. Estandarización, intercalibración, resultados y aplicaciones. Canadá: IDRC/IMTA; p. 71–79.
- Song Y. 2014. Insight into the mode of action of 2,4‐dichlorophenoxyacetic acid (2,4‐D) as an herbicide. J Integr Plant Biol. 56:106–113.
- Souza RB, De Souza CP, Bueno OC, Fontanetti CS. 2017. Genotoxicity evaluation of two metallic-insecticides using Allium cepa and Tradescantia pallida: a new alternative against leaf-cutting ants. Chemosphere. 168:1093–1099.
- Srivastava S, Srivastava A. 2009. Cytostatic and genotoxic effects produced by nitrogen fertilizer breakdown revealed by root mitosis bioassays. Nucleus. 52:79–92.
- Stoltz DR, Khera KS, Bendall R, Gunner SW. 1970. Cytogenetic studies with cyclamat and related compounds. Science. 167:1501–1502.
- Surralles J, Carboneil E, Puig M, Xamena N, Creus A, Marcos R. 1990. Induction of mitotic micronuclei by fenvalerate in cultured human lymphocytes. Toxicol Lett. 54:151–155.
- Şuţan NA, Mayasari S, Popescu A, Toma AM, Deliu I 2015. Evaluation of genotoxic and antimicrobial effect induced by fungicide Dithane M-45. Studia Universitatis Babeş-Bolyai Biologia, LX, Sp. Iss.: 5–18.
- Sutan NA, Popescu A, Mihaescu C, Soare LC, Marinescu MV. 2015. Evaluation of cytotoxic and genotoxic potential of the fungicide ridomil in Allium cepa L. Analele Stiintifice ale Universitatii, Al. I. Cuza Iasi s. II a. Biologie Vegetala. 60(1):5–12.
- Tarkowska JA. 1971. Antimitotic action of glycosides of Nerium oleander L. Hereditas. 67(2):205–211.
- Tedesco SB, Laughinghouse IVHD. 2012. Bioindicator of genotoxicity: the Allium cepa test. In: Srivastava J, editor. Environmental contamination. Croatia: InTech; p. 137–156.
- Teixeira RO, Camparoto ML, Mantovani MS, Vicentini VEP. 2003. Assesment of two medicinal plants Psidium guajava L. and Achillea millefolium L., in in vitro and in vivo assays. Genet Mol Biol. 26(4):551–555.
- Timofeeff-Ressovsky NW, Luchnik NV. 1958. Radiation stimulation of plants and its possible theoretical interpretation. Radiobiologiya. s2.0-84871715658:258–266. in Russian.
- Tomkins DJ, Grant WF. 1976. Monitoring natural vegetation for herbicide-induced chromosomal aberrations. Mutat Res./Fundam Mol Mech Mutagen. 36:73–83.
- Türkoğlu S. 2012. Determination of genotoxic effects of chlorfenvinphos and fenbuconazole in Allium cepa root cells by mitotic activity, chromosome aberration, DNA content, and comet assay. Pesticide Biochem Physiol. 103(3):224–230.
- Verma S, Arora K, Srivastava A. 2016. . Monitoring of genotoxic risks of nitrogen fertilizers by Allium cepa L. mitosis bioassay. Caryologia. 69(4):343–350.
- Verma S, Srivastava A. 2017. Cytomorphologic parameters in monitoring cytogenotoxic effects of fertilizer in Allium cepa L. Environ Monit Assess. 189:159.
- Verma S, Srivastava A. 2018. Cyto-genotoxic consequences of carbendazim treatment monitored by cytogenetical analysis using Allium root tip bioassay. Environ Monit Assess. 190:238.
- Vicentini VEP, Camparoto ML, Teixeira RO, Mantovani MS. 2001. Averrhoa carambola L., Syzygium cumini (L.) Skeels and Cissus sicyoides L.: medicinal herbal tea effects on vegetal and test systems. Acta Scientiarum. 23(2):593–598.
- Wandscheer ACD, Marchesan E, Tedesco SB, Frescura VDS, Soares CF, Londero1 GP, Teló GM, Hansel DSS. 2017. Cytogenotoxicity of rice crop water after application of the tricyclazole fungicide. Anais Da Academia Brasileira De Ciências. 89(2):1251–1258.
- Wuu KD, Grant WF. 1967. Chromosomal aberrations induced by pesticides in meiotic cells of barley. Cytologia. 32:31–41.
- Wuu KD, Grant WF. 1982. Chromosome aberration assays in Allium. A report of the U.S. Environmental protetion agency gene-tox program. Mutat Res. 99:273–291.
- Xu ZX, Tan JJ, Chen FL, Si JH, Xu BN, Li GM, Wu XL. 2003. Farm compost polluted water may induce pharyngoesophageal, gastric and liver carcinoma in the chicken. Zhonghua Zhong Liu Za Zhi. 25:344–347.
- Xue HY, Guo P, Wen WC, Wong HL. 2015. Lipid-based nanocarriers for RNA delivery. Current Pharmaceutical Design. 21(22):3140–3147.
- Xue HY, Liu S, Wong HL. 2014. Nanotoxicity: a key obstacle to clinical translation of siRNA-based nanomedicine. Nanomedicine. 9(2):295–312.
- Yavuz Kocaman A, Kılıç E. 2017. Evaluation of the genotoxicity of commercial formulations of ethephon and ethephon+cyclanilide on Allium cepa L. root meristematic cells. Caryologia. 70:3,229–237.
- Yekeen TA, Azeez MA, Lateef A, Asafa TB, Oladipo IC, Badmus JA, Adejumo SA, Ajibola AA. 2017. Cytogenotoxicity potentials of cocoa pod and bean-mediated green synthesized silver nanoparticles on Allium cepa cells. Caryologia. 70(4):366–377.