Abstract
Decreasing carbon (C) footprints by reducing nitrogen (N) and water inputs has been speculated to have negative impacts on wheat grain yield and flour processing quality. The objective of this study was to determine the impact of N and water stress on winter wheat grain yield, protein composition, and dough quality. Wheat fertilized at two N rates (unfertilized and recommended) was grown under water-stressed and well-watered environments. Nitrogen and water stress were measured using the 13C isotopic approach. Research showed that (1) N fertilizer and the water-management environment produced similar impacts on wheat quality and yield loss due to N stress and yield loss due to water stress (YLWS); (2) N fertilizer increased flour protein, dough stability, and relative concentration of glutenin (%Glu), unextractable polymeric protein (UPP), and relative amount of high-molecular-weight glutenin subunits (HMW-GS/LMW-GS); (3) the well-watered environment reduced protein contents when N mineralization was low, whereas it did not influence protein content when mineralization was high; and (4) the %Glu was negatively correlated with yield loss due to N stress (YLNS) and positively correlated with stability. This study showed that a clear understanding of the complex relationship between soil variability and climatic conditions should make it possible to develop adaptive management practices, increase profitability, and improve quality.
Introduction
The most common factor used to add a premium or discount to the hard red winter wheat (HRWW) selling price is protein. In the northern Great Plains, USA, discount of –$0.03 for each 10 g kg−1 less than 120 g kg−1 and premiums of +$0.015 for each 10 greater than 120 g kg−1 are common (South Dakota Wheat Growers Citation2008). However, millers can also impose regional modifications to the selling price. These discounts may be based on perceptions of previous detailed chemical and quality analysis conducted by the buyer. For example, a buyer might discount the regional purchasing price based on perceived risk of high ash or low dough stability. Once a regional discount is imposed it might take many years to remove. Minimizing the risk for an imposed regional discount requires the development of nitrogen (N)–management practices that fully consider how water and N stress impact yields as well as dough and bread quality.
In the U.S. Northern Great Plains, wide seasonal variations in rainfall and temperature have direct impacts on yield and bread-making quality. Nitrogen and water management impacts the ability of the plant to respond to this variability. In a dry-land environment, the overapplication of N fertilizer can stimulate vegetative growth, which can result in increased water stress at grain filling. In addition, water and N management can influence the metabolic activity within the plant and the protein composition within the kernel (French and Schultz Citation1984; Jamieson, Stone, and Semenov Citation2001; Klupacs et al. Citation2010; Nicolas, Gleadow, and Dalling Citation1985). Prior to adopting N- and water-management practices that will reduce carbon (C) footprints, a clear understanding of the ramification of N and water stress on protein composition and dough quality is needed.
Wheat quality is impacted by management, environment, and genetic interactions by many factors including their impact on the length of the grain-filling periods (Kraljevic-Balallic et al. Citation2001). Conditions that shorten the grain-filling periods directly impact the types and amount of proteins transported to the kernel. For example, gliadin accumulates earlier in grain filling than glutenin (Gupta et al. Citation1996; Zhao et al. Citation2009), and therefore environmental conditions, such as temperature, nutrient deficiencies, and water stress, that shorten the grain-filling period tend to increase the relative concentration of gliadins (%Gli) and decrease the relative concentration of glutenin (%Glu) in the kernel (Johansson, Prieto-Linde, and Svensson Citation2004). Gliadins are a mixture of monomeric polypeptides that contribute to dough viscosity and extensibility (Payne Citation1987; Payne et al. Citation1982), while glutenins form large polymeric structures that impact dough strength and elasticity. After the reduction of disulfide bonds, glutenins can be divided into high- (HMW-GS; 80,000–120,000 Daltons) and low-molecular-weight-glutenin subunit (LMW-GS; 10,000–70,000 Daltons) (Wall Citation1979; Weegels, Hamer, and Scholfield Citation1996). The HMW-GS influences gluten strength and elasticity, whereas LMW-GS influences dough strength (Bietz and Wall Citation1972).
In the region, research is being conducted to reduce N and water inputs. Much of this research is focused on developing new N and water recommendations based on yields and protein concentration. In most cases protein composition and dough quality are not considered in these considerations. To develop a mechanistic understanding on how stress and management interact to influence yield and bread-making quality, the research needs to be expanded to include the impact of N and water stress on protein composition and flour quality. The objective of this study was to determine the impact of N and water stress on winter wheat grain yield, protein composition, and dough quality.
Materials and Methods
Plant Materials and Cultural Practices
Field study was conducted on a Lowry silt loam (coarse-silty, mixed mesic typic haplustolls) at the Dakota Lake Research Farm (99° 59ʹ W latitude and 44° 17ʹ N longitude) in South Dakota, USA, in two consecutive years (2007 and 2008). Growing degree days (GDDs, base 0 °C) were summed from planting to crop maturity and were 1,891 in the first year and 2,262 in the second year. During the 2007 and 2008 growing seasons, precipitation was 457 and 450 mm, respectively. These totals were supplemented with additional water by placing a line source irrigation system in the center of the experimental area. Plots 2.3 m from the line source were identified as well watered and plots 16.0 m from the line source were identified as water stressed. In the well-watered treatment, tensiometer readings at depth of 45 and 90 cm were used for irrigation planning. In the first year, the irrigation plus natural rainfall amounts in the well-watered and water-stressed plots were 687 and 519 mm, respectively, whereas in 2008 irrigation plus natural rainfall amounts in the well-watered and water-stressed treatments were 609 and 528 mm, respectively.
The N rates in 2007 were 0 and 200 kg N ha−1, whereas in 2008 the N rates were 0 and 160 kg N ha−1. Nitrogen fertilizer [urea and ammonium nitrate (UAN); 28–0–0] was applied at Feekes 3.0. Each treatment was replicated four times. Winter wheat was planted at 4.44 × 106 seeds ha−1 (145 kg ha−1) on 21 September 2006 and 8 September 2007, respectively. The grain yield was measured following crop maturity with a plot combine with a 1.52-m header. The grain yields were adjusted to 13.5% moisture, whereas protein values were adjusted to the 12% moisture basis.
Quality Analyses
For quality analysis, bulk wheat samples were cleaned by hand and tested with an electric dockage tester (Carter Day International, Minneapolis, Minn., USA). The grain samples were milled according to the American Association of Cereal Chemists (AACC)–approved method 26-50.01 (AACC International Citation2011a). The milled samples were weighed and sieved through an ASTM E-11 No. 62 sieve (0.30 mm) (Fisher Scientific, Pittsburgh, Penn., USA) and then shaken on a mechanical shaker for 4 min to recover white flour.
Grain protein contents, flour protein contents, and moisture contents were determined with a near-infrared analyzer (model 6500, Foss NIR Systems, Laurel, Md., USA) according to AACC-approved methods 39-10.01 (AACC International Citation2011b) and 39-11.01 (AACC International Citation2011c). The flour-mixing characteristics were analyzed by Farinograph (C. W. Brabender Instruments, South Hackensack, N.J., USA) with a 50-g bowl following AACC-approved method 54-21.01 (AACC International Citation2011d). The water absorption, peak time, stability, mixing tolerance index (MTI), and breakdown time were calculated (AACC Citation1984).
Protein Fractionation and Characterization
The wheat flour samples for size-exclusion high-performance liquid chromatography (SE-HPLC) were prepared according to Singh, Donovan, and MacRitchie (Citation1990) and Sissons et al. (Citation2005). The flour samples (500 mg) were extracted with a buffer containing 5 mg mL−1 sodium dodecyl sulfate (SDS) and 0.05 M sodium phosphate at pH 6.9 for 20 min with continuous mixing. The extract was centrifuged (10,000g) for 3 min, and the supernatant [sodium dodecyl sulfate (SDS)–soluble fraction] was removed. The residue fraction was further extracted with the same buffer by sonication for 3 min, which solubilized the large polymeric glutenins, and were then centrifuged (10,000g) for 3 min. The fractions were injected into a Phenomenex BIOSEP-SEC 4000 column (300 mm × 7.8 mm, Phenomenex, Torrance, Calif., USA) to run for 10 min at 2 ml min−1 in a 1:1 mixture of deionized water containing 0.5 mg ml−1 trifluoroacetic acid (TFA) and acetonitrile containing 0.5 mg ml−1 TFA using a Waters HPLC system (Waters Corporation, Milford, Mass., USA). Proteins were detected by ultraviolet (UV) absorbance at 210 nm. The relative amounts of albumins + globulins, glutenins, gliadins, and unextractable polymeric proteins (UPP) were calculated based on total SE-HPLC area of extractable and unextractable proteins.
Samples for reverse-phased-HPLC (RP-HPLC) were prepared according to Fu and Sapirstein (Citation1996). The flour samples (100 mg) were initially extracted in 50% (v/v) 1-propanol for 15 min at room temperature with intermittent vortexing followed by centrifugation (10,000g) for 3 min. The residue was washed with 500 μl of 50% 1-propanol to remove any remaining soluble protein. Glutenin was extracted from the residue in 0.08 M Tris-hydrochloric acid (HCl) containing 50% 1-propanol at pH 8.0 and containing 1% (w/v) freshly added dithiothreitol after a brief initial vortexing. The proteins were extracted for 30 min at 60 °C. The extract was then alkylated in 0.08 M Tris-HCl containing 50% 1-propanol, pH 8.0, and containing 4% (v/v) freshly mixed 4-vinylpyridine. The extract was incubated for 15 min at 60 °C and then centrifuged (10,000g) for 3 min. The samples were injected into a Vydac 218 TP54 column (C18 5 um, 250 mm × 2.6 mm, Grace, Deerfield, Ill., USA). Protein separation was carried out using a flow rate of 0.2 ml min−1 at 60 °C using a Waters HPLC system (Waters Corporation, Millford, Mass., USA). The eluents were purified water and acetonitrile, each containing 0.1% (v/v) TFA. The measured RP-HPLC fractions were HMW-GS and LMW-GS. Each analysis was conducted in duplicate.
Yield Loss Calculation
The grain samples were dried, ground on a cyclone mill, and analyzed on an isotope ratio mass spectrometer (Europa Scientific Ltd., Westchester, UK) for total N, total C, delta 15N (δ15N), and delta 13C (δ13C). The δ13C values were used to calculate the 13C isotopic discrimination value with the following equation:
Table 1 Influences of year, water-stress environment, and N rate on grain yield (kg ha−1), grain protein (g kg−1), and yield loss due to nitrogen stress (YLNS) and water stress (YLWS)
Statistical Analyses
Experimental design was a randomized split block with four replications. The statistical analysis approach followed the method reported by Stroup (Stroup Citation1989). In this analysis, a first-order autoregressive model under PROC MIXED in SAS 9.1 was used to determine the treatment differences for the individual years (SAS Institute Inc. Citation2008). Block was random factor whereas N and water were fixed factors.
Results and Discussion
Climate and Soil Effects
The well-watered and water-stressed environments were designed to simulate moisture differences between the summit/shoulder and footslope/toeslope areas. In addition to the simulated water environments, temperatures were warmer in 2008 than 2007. Interactions between the moisture and temperature regimes resulted in four environmental conditions.
Greater N mineralization in 2007 (192 kg N ha−1) than 2008 (99 kg N ha−1) produced an apparent N response differences across years. In year 1, the greatest yields were in the fertilized well-watered environment, and lowest yields were in the unfertilized water-stressed environment (). In addition, the well-watered environment had lower YLNS and YLWS. The impact of water environment on YLNS was attributed to a synergistic relationship between N and water. The net result in year 1 was similar protein contents in both water environments. Slightly different results were observed in the second year when N fertilizer and water environment had a synergistic impact on grain yield and an antagonistic impact on protein (). The antagonistic impact on protein content was attributed to protein dilution.
The apparent differences between year 1 and year 2 in the relationship between N rate and protein could be explained by building a model that considered N mineralization and the N fertilizer rate (). Differential N mineralization may have also impacted dough quality (). In the first year, peak time was generally increased by N, but it was not impacted by water environment. Nitrogen addition also increased stability and breakdown and reduced MTI. The decrease in MTI with N was attributed to the impact of protein on dough strength; that is, greater MTI values mean lower stability. Others have had similar findings (Al-Eid Citation2006; Ma et al. Citation2009).
Table 2 Influence of year, water-stress environment, and N rate on protein (g kg−1) and selected dough quality parameters
Figure 1. Relationship between N additions (N fertilizer + mineralized N) and protein content over 2 years.
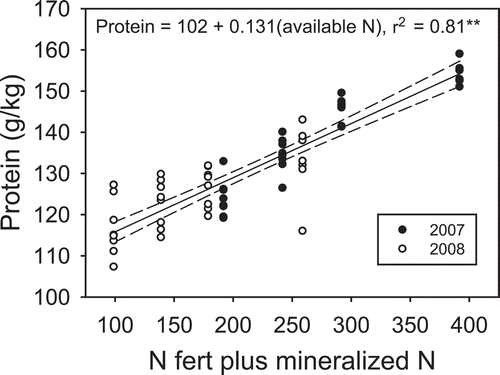
In year 2, yields and YLWS were much greater than year 1 (). These results were attributed to greater temperatures in 2008 than 2007. Associated with greater yields was an N fertilizer–induced increase in peak time. Plants growing in the water-stressed environment had greater protein contents, dough stability, and breakdown times than those growing in the well-watered environment. A comparison across environments showed that environment had an impact on the relationship between protein content and stability (). However, this apparent difference could be explained by considering water stress [; stability = –11.17 + 1.34 (protein) + 0.0033 (YLWS), R2= 0.42**]. These finding shows that a single model could be used to explain the impacts of water and N stress on the wheat quality.
Effects of N and Water Stress on Protein Characterization
The imposed N and water treatments on winter wheat impacted protein composition, which in turn impacted dough quality. In the first year of study, N and water produced synergic impacts on protein composition (). The addition of both N and water enhanced the %Glu and UPP contained in the kernel. Opposite impacts were observed for the Gli/Glu ratio. For the HMW-GS/LMW-GS ratio in-season N increases the ratio, whereas water did not influence the ratio. In the second year of study, different results were observed. As in the first year, %Glu was increased by N and water. However, combining both the water and N treatment decreased the differences. Opposite impacts were observed for %Gli. On the HMW-GS/LMW-GS ratio N fertilizer increased the ratio, whereas water addition did not impact the ratio. Nitrogen had a similar impact on the HMW-GS/LMW-GS ratio, but water stress did not affect the HMW-GS/LMW-GS ratio in both years. Contrasts between the 2 years were attributed to (1) greater N mineralization rates in the first year than the second year of study and (2) water enhancing the uptake of soil and fertilizer N in the first year of study (Kharel et al. Citation2011). Others (Gupta, Batey, and MacRitchie Citation1992; Jia et al. Citation1996; Johansson, Prieto-Linde, and Svensson Citation2004; Triboi et al. Citation2000) have produced similar and different results. For example, Johansson, Prieto-Linde, and Svensson (Citation2004) reported% Glu increased with N application, while Triboi et al. (Citation2000) reported that N increased the Gli/Glu ratio. Differences between the studies may be related to the impact of N on the length of the grain-filling period and water and N stress. Jiang et al. (Citation2009) reported that water stress after anthesis increased the accumulation of HMW-GS during the early grain-filling stage. Konopka et al. (Citation2007) reported that wheat grains obtained under stress conditions were less abundant in LMW- and HMW-GS.
Table 3 Influences of water-stress environment and N rate on protein characterization in 2007 and 2008
The protein fraction analysis across years showed that %Glu and HMW-GS/LMW-GS ratios were negatively correlated with YLNS and positively correlated with stability whereas the Gli/Glu ratio was negatively correlated with stability and positively correlated with YLNS ( and ). These results show that the protein composition was influenced by N stress, and that across the 2 years, glutamine was greatest when N stress was the lowest. These results are attributed to gliadin accumulating earlier in the kernel than glutenin and stress reducing the length of the grain-filling period (Panozzo and Eagles Citation2000). For example, Panozzo and Eagles (Citation2000) reported that high temperatures (>30 °C) increased the %Gli concentrations. The protein composition impacted dough stability because gliadins are a mixture of monomeric polypeptides that contribute to dough viscosity and extensibility (Payne Citation1987), whereas glutenins are large polymeric structures that impact dough strength and elasticity. Dough stability was not correlated with %Glu, the Gli/Glu ratio, or the HMW-GS/LMW-GS ratio. However, Wang and Yu (Citation2009) reported that when N was added, the grain protein and flour protein contents and the %Glu increased whereas the %Gli and the Gli/Glu ratio decreased, which induced the increase of stability.
Figure 4. Relationships between protein fraction [percentage of glutenin (A), gliadin/glutenin ratio (B), and HMW-GS/LMW-GS ratio (C)] and stability in 2007 and 2008.
![Figure 4. Relationships between protein fraction [percentage of glutenin (A), gliadin/glutenin ratio (B), and HMW-GS/LMW-GS ratio (C)] and stability in 2007 and 2008.](/cms/asset/75e9b0bf-90b2-4f7f-980c-78c15ee12c13/lcss_a_909833_f0004_b.gif)
The composition of the glutenin protein was impacted by stress. Across the 2 years the HMW/LMW-GS ratio decreased with increasing N stress (), which in turn impacted stability (). There results agree with the findings by both Bayoumi and Demardash (Citation2008) and Luo et al. (Citation2000).
Conclusions
This experiment produced four uniquely different environments. In year 1, temperatures were lower and N mineralization was greater than year 2. This environment resulted in greater N mineralization, lower yields, lower yield losses due to water stress, and synergistic relationships between water and N use. For both years, N fertilizer generally improved dough quality, and the relative glutenin amount and HMW-GS/LMW-GS ratio were negatively correlated with and positively correlated with stability. A single model could be used to explain protein accumulation and the relationship between protein content and stability.
This article had three major conclusions. The first conclusion was that soil and climate variability could impact yields, protein composition, and dough quality. To quantify these impacts, N mineralization was measured. The second conclusion was that by understanding complex relationships between climatic conditions and soil variability it should be possible to develop adaptive management practices to increase profitability and reduce greenhouse gas footprints. The third conclusion was that routine measurements of dough quality and protein composition should be integrated into management studies.
Funding
Support was provided by South Dakota Wheat Commission, the SD Drought Tolerance Center, South Dakota 2010 grant program, South Dakota Experiment Station, and USDA-NIFA-AFRI.
References
- AACC. 1984. Interpretation of farinogram. In Farinograph handbook, ed. B. L. D’Appolonia and W. H. Kunerth, 31–32. St. Paul, Minn.: American Association of Cereal Chemists.
- AACC International. 2011a. Method 26-50.01: Brabender Quadrumat Jr. (quadruplex) method. In Approved methods of analysis, 11th ed. St. Paul, Minn.: AACC International.
- AACC International. 2011b. Method 39-10.01: Near-infrared reflectance method for protein determination in small grains. In Approved methods of analysis, 11th ed. St. Paul, Minn.: AACC International.
- AACC International. 2011c. Method 39-11.01: Near-infrared reflectance method for protein determination in wheat flour. In Approved methods of analysis, 11th ed. St. Paul, Minn.: AACC International.
- AACC International. 2011d. Method 54-21.01: Farinograph method for flour. In Approved methods of analysis, 11th ed. St. Paul, Minn.: AACC International.
- Al-Eid, S. M. 2006. Effect of nitrogen and manure fertilizer on grain quality, baking, and rheological properties of wheat grown in sandy soil. Journal of the Science of Food and Agriculture 86:205–211.
- Bayoumi, T. Y., and I. S. E. Demardash. 2008. Influence of nitrogen application on grain yield and end use quality in segregating generations of bread wheat (Triticum aestivum L.). African Journal of Biochemistry Research 2:132–140.
- Bietz, J. A., and J. S. Wall. 1972. Wheat gluten subunits: Molecular weights determined by sodium dodecyl sulfate polyacrylaminde gel electrophoresis. Cereal Chemistry 49:416–30.
- Clay, D. E., S. A. Clay, D. J. Lyon, and J. M. Blumenthal. 2005. 13C discrimination in corn grain can be used to separate and quantify yield losses due to water and nitrogen stresses. Weed Science 53:23–29.
- Clay, D. E., R. E. Engel, D. S. Long, and Z. Liu. 2001. Nitrogen and water stress interact to influence carbon-13 discrimination in wheat. Soil Science Society of America Journal 65:1823–1828.
- French, R. J., and J. E. Schultz. 1984. Water use efficiency of wheat in a Mediterranean-type environment, II: Some limitations to efficiency. Australian Journal of Agricultural Research 35:765–775.
- Fu, B. X., and H. D. Sapirstein. 1996. Procedure for isolating monomeric proteins and polymeric glutenin of wheat flour. Cereal Chemistry 73:143–452.
- Gupta, R. B., I. L. Batey, and F. MacRitchie. 1992. Relationships between protein composition and functional properties of wheat flours. Cereal Chemistry 69:125–131.
- Gupta, R. B., S. Masci, D. Lafiandra, H. S. Bariana, and F. MacRitchie. 1996. Accumulation of protein subunits and their polymers in developing grains of hexaploid wheats. Journal of Experimental Botany 47:1377–1385.
- Jamieson, P. D., P. J. Stone, and M. A. Semenov. 2001. Towards modeling quality in wheat from grain nitrogen concentration to protein composition. Aspects of Applied Biology 64:111–126.
- Jia, Y. Q., V. Masbou, T. Aussenac, J. L. Fabre, and P Debaeke. 1996. Effects of nitrogen fertilization on maturation conditions on protein aggregates and on the breadmaking quality of a common wheat cultivar. Cereal Chemistry 73:123–130.
- Jiang, D., H. Yue, B. Wollenweber, W. Tan, H. Mu, Y. Bo, T. Dai, Q, Jing, and W. Cao. 2009. Effect of post-anthesis drought and water logging on accumulation of high molecular weight subunits and glutenin macropolymers content in wheat grain. Journal of Agronomy and Crop Science 195:89–97.
- Johansson, E., M. L. Prieto-Linde, and G. Svensson. 2004. Influence of nitrogen application rate and timing on grain protein composition and gluten strength in Swedish wheat cultivars. Journal of Plant Nutrition and Soil Science 167:345–350.
- Kharel, T. P., D. E. Clay, S. A. Clay, D. Beck, C. Reese, G. Carlson, and H. Park. 2011. Nitrogen and water stress affect winter wheat yield and dough quality. Agronomy Journal 103:1389–1396.
- Klupacs, H., A. Tarnawa, I. Balla, and M. Jolankai. 2010. Impact of water availability on winter wheat (Triticum aestivum L.) yield characteristics. Agrokémia és Talajtan 59:151–156.
- Konopka, I., M. Tanska, A. Pszczołkowska, G. Fordonski, W. Kozirok, and J. Olszewski. 2007. The effect of water stress on wheat kernel size, color, and protein composition. Polish Journal of Natural Sciences 22:157–171.
- Kraljevic-Balallic, M., A. J. Worland, E. Porceddu, and M. Kuburovic. 2001. Variability and gene effect in wheat. In Genetics and breeding of small grains, ed. S. Ouarrie, 9–49. Belgrade, Serbia: Agricultural Research Institute Serbia.
- Luo, C., G. Branlard, W. B. Griffin, and D. L. McNeil. 2000. The effect of nitrogen and sulphur fertilization and their interaction with genotype on wheat glutenins and quality parameters. Journal of Cereal Science 31:185–194.
- Ma, D., T. Guo, Z. Wang, C. Wang, Y. Zhu, and Y. Wang. 2009. Influence of nitrogen fertilizer application rate on winter wheat (Triticumaestivum L.) flour quality and Chinese noodle quality. Journal of the Science of Food and Agriculture 89:1213–1220.
- Nicolas, M. E., R. M. Gleadow, and M. J. Dalling. 1985. Effect of post-anthesis drought on cell division and starch accumulation in developing wheat grains. Annals of Botany 55:433–444.
- Panozzo, J. F., and H. A. Eagles. 2000. Cultivar and environmental effects on quality characteristics in wheat, II: Protein. Australian Journal of Agricultural Research 51:629–636.
- Payne, P. I. 1987. Genetics of wheat storage proteins and the effect of allelic variation on bread making quality. Annual Review of Plant Physiology 8:141–153.
- Payne, P. I., L. M. Holt, G. J. Lawrence, and C. N. Law. 1982. The genetics of gliadin and glutenin, the major storage proteins of the wheat endosperm. Plant Foods for Human Nutrition 31:229–241.
- SAS Institute Inc. 2008. SAS 9.1 documentation. Cary, N.C.: SAS Institute. Available at http://support.sas.com/documentation/onlinedoc/91pdf/index_913.html.
- Singh, N. K., R. Donovan, and F MacRitchie. 1990. Use of sonication and size-exclusion high performance liquid chromatography in the study of wheat flour proteins, I: Dissolution of total proteins in the absence of reducing agents. Cereal Chemistry 67:150–161.
- Sissons, M. J., N. Ames, R. A. Hare, and J. M. Clarke. 2005. Relationship between glutenin subunit composition and gluten strength measurements in durum wheat. Journal of the Science of Food and Agriculture 85:2445–2452.
- South Dakota Wheat Growers. 2008. South Dakota Wheat Growers cash grain prices. Availabel at http://www.sdwg.com/UserFiles/TempFTP/Grain%20Prices/Prices.htm.
- Stroup, W. W. 1989. Use of mixed model procedure to analyze spatially correlated data: Sn example applied to a line-source sprinkler irrigation experiment. In Applications of mixed models in agriculture and related disciplines (Southern Cooperative Ser. Bull. No. 343), 104–122. Baton Rouge: Louisiana Agricultural Experiment Station.
- Triboi, E., A. Abad, A. Michelena, J. Loveras, J. L. Ollier, and C. Daniel. 2000. Environmental effects on the quality of two wheat genotypes, 1: Quantitative and qualitative variation of storage proteins. European Journal of Agronomy 13: 47–64.
- Wall, J. S. 1979. The role of wheat protein in determining baking quality. In Recent advances in the biochemistry of cereals, ed. D. L. Laidmond, and R. G. Wyn Jones, 275–311. London: Academic Press.
- Wang, X. Y., and Z. W. Yu. 2009. Effect of interactions between water management and nitrogen fertilizer on wheat processing quality and their relationship to protein fractions. Journal of Triticeae Crops 29:518–523.
- Weegels, P. L., R. J. Hamer, and J. D. Scholfield. 1996. Critical review: Functional properties of wheat glutenin. Journal of Cereal Science 23:1–18.
- Zhao, C. X., M. R. He, Z. L. Wang, Y. E. Wang, and Q. Lin. 2009. Effects of different water availability at post-anthesis stageon grain nutrition and quality in strong-gluten winter wheat. Comptes Rendus Biologies 332:759–764.