ABSTRACT
Interaction among plant nutrients can yield antagonistic or synergistic outcomes that influence nutrient use efficiency. To provide insight on this phenomenon, peer-reviewed articles were selected that quantified the interaction effects of nutrients on crop yield levels. In total 94 articles were selected that described 117 interactions between all macro- and micronutrients for different agricultural crops. In 43 cases the interaction was synergistic, in 17 cases the interaction was antagonistic, and in 35 cases the interaction was zero-interaction; the other 23 cases were non-significant (16) or showed a negative response (7). Generally: (a) when the availability of two nutrients is characterized as deficient, a large increase in yield can be expected by diminishing these deficiencies: (b) for most macronutrients the mutual interactions on yield levels are synergistic; and (c) antagonistic effects on yield are often found for divalent cations. Knowledge of nutrient interactions can guide fertilizer design and optimization of fertilization strategies for high yields and high nutrient use efficiencies.
Introduction
The increase in global food demand will require an increased use of natural resources such as water, land, and nutrients to produce crops (Tilman et al. Citation2011). Three major pathways have been identified to meet this growth: decreasing the loss of agro-ecological production capacity, decreasing the demand of food per capita, and increasing the production of food (Dogliotti, Giller, and Van Ittersum Citation2014). The growth of the global food production is projected to require increased use of chemical fertilizers, but since the current environmental impact of agriculture and fertilizer use has reached its planetary boundaries (Steffen et al. Citation2015), the nutrient use efficiency of fertilizers should be increased dramatically. The current yield trends are insufficient to meet forecasted food demands (Ray et al. Citation2013), which implies a daunting challenge to limiting the use of fertilizers and increasing yields. This problem reveals the great potential to increase the nutrient use efficiency, and consequently, yield levels by considering all essential plant nutrients (macronutrients N, P, K, Ca, Mg, and S and micronutrients Cl, Fe, B, Mn, Zn, Cu, Mo, and Ni) in fertilizer products and fertilization strategies (Bindraban et al. Citation2015; Dimkpa and Bindraban Citation2016).
One of the causes of the current stagnating yield levels is the deficiency or imbalance of nutrients (Lobell, Cassman, and Field Citation2009). This problem suggests the need for a great potential to increase the nutrient use efficiency, and consequently, yield levels by considering all essential plant nutrients (macronutrients N, P, K, Ca, Mg, and S and micronutrients Cl, Fe, B, Mn, Zn, Cu, Mo, and Ni) in fertilizer products and fertilization strategies. To achieve these benefits, it will be imperative to apply balanced amounts of the most limiting nutrients to obtain the highest yield while minimizing nutrient losses, that is, when fertilization is fine-tuned to local soil chemical conditions and crop requirements (Roy et al. Citation2006).
In designing fertilizers with the right nutrient composition, antagonistic (negative) nutrient interactions should be minimized, whereas synergistic (positive) nutrient interactions should be maximized for optimal nutrient use efficiency. These actions require proper knowledge of possible positive and negative interactions between nutrients. The objective of this review is to provide an overview of the effects of these interactions on fertilizer use efficiencies and crop yields. To this end, the occurrence of antagonism and synergism in crops was assessed by posing a number of questions: (1) what is the quantitative nature of nutrient antagonism and synergism, (2) is there a relationship between the uptake of micro- and macronutrients, (3) which mechanisms are responsible for the interactions, and (4) what is the influence of nutrient transporters, reductase enzymes, and phytosiderophore production.
Material and methods
We reviewed the scientific literature on the effects of nutrients and their interactions on the yield of crops. The keywords were obtained from well-known references on mineral nutrition of plants: specifically ‘fertilizer technology’ (Chien, Prochnow, and Cantarella Citation2009; Mortvedt, Cox, and Shuman Citation1991), “fertilization,” “interactions between plant nutrients” (Tisdale, Nelson, and Beaton Citation1985), “micronutrients” (Alloway 2008; Welch Citation1995), “principles of ion uptake by plants” (Epstein and Bloom Citation2005; Mengel et al. Citation2001; White Citation2012), and “specific fertilization of various important crop types” (Fageria, Baligar, and Jones Citation2011).
To compile a dataset of quantitative effects of interactions, we searched for peer-reviewed literature investigating the effects of interactions between nutrients on yield using Scopus (Elsevier). The search-query was: ((TITLE-ABS-KEY ((nitrogen AND magnesium) OR (potassium AND iron) OR (calcium AND iron) OR (magnesium AND calcium) OR (zinc AND calcium) OR (iron AND phosphorus) OR (manganese AND potassium) OR (copper AND phosphorus) OR (nitrogen AND potassium)) AND TITLE-ABS-KEY ((potassium AND phosphorus) OR (calcium AND potassium) OR (magnesium AND phosphorus) OR (zinc AND phosphorus) OR (nitrogen AND borate) OR (potassium AND borate) OR (calcium AND manganese)))) AND (TITLE-ABS-KEY (nutrient OR fertilizer)) AND (TITLE-ABS-KEY (antagonism OR interaction OR synergism)) AND (TITLE-ABS-KEY (plant OR crop OR root OR leaves)) AND NOT (TITLE-ABS-KEY (trees OR cadmium OR toxic OR lead OR moss OR forest)) AND (LIMIT-TO (DOCTYPE, “ar”)). This search produced a total of 349 publications (accessed Nov 6, 2014). The papers found using this method were selected. The references in the papers and references to the selected papers were used for additional publications.
The papers were screened on interaction between nutrients as reflected in the yield. To address the research questions as listed above, several exclusion criteria were used. The experimental papers that did not include original data or a statistical evaluation of the interaction were excluded. Because the main focus for this review was on the positive effects of fertilization, a large number of studies about the toxic effects of copper, zinc, and boron were excluded. Papers describing the variation of nutrient supply by different soils and possible effects on nutrient interactions were also not considered. In total 94 papers were selected to compile the dataset on synergistic and antagonistic effects.
Approach
Interactions between nutrients occur when the supply of one nutrient affects the uptake, distribution, or function of another nutrient. Depending on the nutrient supply, the interaction can modify plant growth and yield. Interactions can be assessed by examining the relationship between nutrient supply and nutrient concentrations in plants and by examining the relationship between nutrient supply and plant growth (Robson and Pitman Citation1983). It has resulted in many possible relations between the supply of a nutrient and the effects on plants (Landon Citation1991; Robson and Pitman Citation1983) that have been discussed in detail in various reviews and textbooks (Alloway 2008; Fageria et al. Citation2013; Pan Citation2012; Tisdale, Nelson, and Beaton Citation1985; White Citation2012; Wilkinson, Grunes, and Sumner Citation2000; Zhang, Shen, and Zhu Citation2006). Knowledge about these interactions is important to understand nutrient uptake processes. In many publications, the effects on concentrations, or accumulation in plants, is used as the main parameter to assess nutrient interactions (Gunes, Alpaslan, and Inal Citation1998).
Nutrient interaction in crops is probably one of the most important factors affecting yields of annual crops (Fageria Citation2014). On the one hand, nutrient interaction at the root uptake level may be studied deterministically based on well-conditioned experiments, and on the other hand, it can be determined agronomically by studying nutrient availability and fertilizer effects on crop yield. The deterministic approach eliminates external influences such as other limiting nutrients, water limitation or water excess, temperature and pH, but these results may often not be transferred to field conditions. In contrast, agronomic studies have the disadvantage that external influences are uncontrolled and that the results can be valid only for the prevailing conditions because of a host of confounding variables. According to Fageria (Citation2014): “Interactions occur when the supply of one nutrient affects the absorption and utilization of another nutrient. […] Nutrient interactions affect plant growth and development only when the supply of a determined nutrient is too low compared to the applied ones. In other words, yield decrease occurs only when the supply of some nutrients falls below the critical level. If the soil or growth medium has sufficient supply of other essential nutrients compared to the added one, plant growth will not be affected adversely, even though the uptake of some nutrients may decrease. Hence, plant growth or yield is considered a better criterion for evaluating nutrient interactions in crop plants.” The rationale for performing this review is to improve fertilization toward balanced fertilizer application and enhanced fertilizer use efficiency. Therefore, we have selected the crop yield approach in this review while not further considering nutrient uptake.
The effect of interactions between nutrients on yield of crops has been reviewed before for specific nutrients: for N (Aulakh and Malhi Citation2005; Fageria Citation2014), for K (Daliparthy, Barker, and Mondal Citation1994; Dibb and Thompson Citation1985), for P (Sumner and Farina Citation1986), and specific for N-K interactions (Zhang et al. Citation2010), but not for the entire array of all essential nutrients.
Synergism, zero-interaction, and antagonism
Pan (Citation2012) described theoretical studies (Rubio, Zhu, and Lynch Citation2003; Wallace Citation1990; Zinn, Witholt, and Egli Citation2004) to classify nutrient interactions on the basis of quantitative descriptions of data and models. We adopted their methodology that is most common in the literature studying this phenomenon. Yield will be used as the main parameter to assess nutrient interactions, which can either be synergism, antagonism, or zero-interaction. Synergism refers to the response that is greater than expected from the individual responses (Aulakh and Malhi Citation2005; Fageria Citation2014, Citation2001; Roy et al. Citation2006; Tisdale, Nelson, and Beaton Citation1985; Wallace Citation1990; Wilkinson, Grunes, and Sumner Citation2000). The yield expected (yab) on the basis of the individual responses (ya and yb) for the situation of zero-interaction follows from
where y0 is the yield in the reference or control treatment (). The calculation of the expected yield (yab) as a product of the individual responses according to Eq. (1) is based on Wallace (Citation1990) and, to our knowledge, is an operational definition and is not based upon a plant physiological process. By using relative yields, it is possible to compare between different experiments, crops, fertilizers, and to account for variations in the control treatments. Wallace (Citation1990) has suggested that many interactions can be described according to Eq. (1). Therefore, we also used this criterion for zero-interaction. Antagonism refers to the yield in response of two nutrients in which the combined effect is less than expected from the individual responses (expected from individual responses: zero interaction, according to Eq. (1)) (Aulakh and Malhi Citation2005; Fageria Citation2001; Sumner and Farina Citation1986), or in other words, that the actual relative yield is less than the product of the individual yield effects. Thus, even if the yield in a plot treated with nutrient a and b is higher than the plots treated with a or b, the effect is synergistic only when the yield response exceeds the expected yield on the basis of the individual responses, that is, the actual relative yield is greater than the product of the individual yield effects (Aulakh and Malhi Citation2005).
Table 1. Definition of synergistic, antagonistic, zero-interaction, and Liebig-synergism.
Results and discussion
In total 116 interactions between nutrients on crop yield have been identified in the 94 publications. Details are presented in Appendix 1, and the major findings regarding nutrient interactions are discussed below. It is expected that the use of the categories synergism and antagonism is useful to describe the interaction between a pair of nutrients because it gives an indication of the yield response that might be expected from the use of fertilizers containing these nutrients.
An example of synergism is given in _see below: Fageria and Oliveira (Citation2014) found large increase in rice grain yield by using K and P. The observed effect (relative yield) of the combination of both nutrients is compared to the calculated effect obtained as the product of the individual effects (i.e., 1.1 × 1.2), and appears greater (1.6) than the product of the individual effects according to Eq. (1), indicating synergism.
Table 2. Example of synergism. The yielda is given as function of the application of nutrients.
Synergism was identified in 23 cases, especially between macronutrients (). The Liebig-synergism, in which a deficiency is so severe that it first has to be resolved to obtain synergism with other nutrients, was coincidently identified also in 20 cases. presents an example of the Liebig-synergism. This interaction appears to be antagonistic at zero Cu application, as the addition of N did not raise yield, but rather suppressed it, suggesting Cu deficiency to be the prime factor limiting yield. When both nutrients were supplied simultaneously, the yield increase was much higher than the yield due to the individual effects.
Table 3. Example of a specific type of synergism: Liebig-synergism. The yielda is given as a function of the application of nutrients.
Figure 1. Interactions between nutrients. Number in squares refer to the number of studies of each type of interaction a. synergistic or Liebig-synergistic (totaling 43 cases). b. zero-interaction (totaling 35 cases). c. antagonistic (totaling 17 cases).
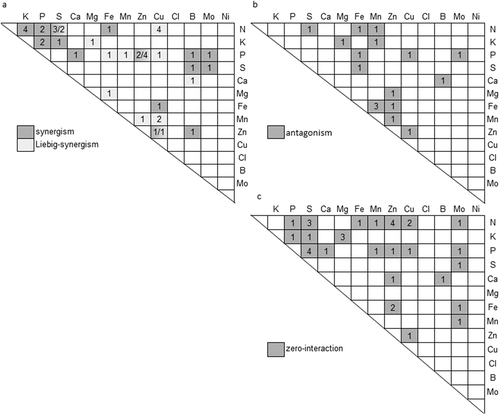
The distinction between synergism and the Liebig-synergism is essential for a fertilization strategy. In the 23 studies that show a synergistic interaction, the quotient of the actual and the predicted yield increase varies between 1 and 3 (Appendix 1). When severely limiting factors occur, and are corrected, resulting in Liebig-synergism, the response in yield is difficult to predict (Wallace Citation1990). In the 20 studies that show a Liebig-synergistic interaction, the quotient of the actual and the predicted yield increase varied between 1.5 and 35, demonstrating the variation involved in Liebig-synergism, as a result of the negligible response by a second nutrient that cannot overcome the severely limiting prime nutrient.
Synergistic interactions are well known for N x K and N x P interactions (Aulakh and Malhi Citation2005). They are not only important for the yield but also help to explain their combined effect on root growth, and the relevance for synchronized applications of, for example, N and K, during the growing season (Aulakh and Malhi Citation2005), ultimately resulting in improved nutrient use efficiency (NUE) (kg product per kg applied N, corrected for control in which no fertilizer is used) (). Some micronutrients reveal synergism with macronutrients (). The addition of Zn resulted in a higher yield of wheat on a calcareous soil, and the increase due to Zn was largest at the highest addition of macronutrients () (Sakal, Singh, and Sinha Citation1988).
Table 5. Improved nitrogen use efficiency due to interaction with other nutrients (partly based on Aulakh and Malhi Citation2005).
Table 6. Grain yield of wheat (t ha−1) as influenced by NPK and Zn applications (Sakal, Singh, and Sinha Citation1988).
Some synergistic responses to nutrients may result from soil reactions such as the acidifying or reducing effects of the fertilizer nutrient. NH4 fertilizers increase yield of barley and oats under Mn deficiency (Petrie and Jackson Citation1984) due to the reduction of the unavailable Mn(IV) to available Mn(II) in soil (Husted et al. Citation2005). Also, thiosulfate can reduce Mn(IV) in soil and increase the Mn uptake by Mn-deficient plants (Husted et al. Citation2005).
Zero-interaction was identified in 35 cases (). An example for zero-interaction is given in . Either K or P individually improve the yield of soybean. The interaction effect in this case is predictable, as it follows from the individual effects: the relative yield increase is (about) equal to the product of the relative yield increase for the individual effects (1.5 ≈ 1.16 × 1.30) (Aulakh and Malhi Citation2005; Fageria Citation2001; Sumner and Farina Citation1986). It is noteworthy that zero-interaction does not imply no effect of fertilizer on yield, but rather the lack of an additive effect.
Table 7. Example of a zero-interaction. The yielda is given as function of the application of nutrients.
Antagonism was identified in 17 cases. An example of antagonism is given in . Either Zn or Mg has strong positive effects on the growth of wheat, while the combined effect is less than the product of the individual effects. The quotient of the predicted and the actual yield varies between 0.3 and 0.9. Such interactions appear also, for example, between N and minerals that improve the symbiotic N fixation in plants (e.g., Mo, Ca, or Cu) (Robson and Pitman Citation1983): they both improve the N deficiency. Antagonisms are detected mainly between cations: K, Cu, Fe, Mn, and Zn (), as will be discussed later in the section about preferential transport of nutrients. Two exceptions that do not involve these cations are the antagonisms for Mo x P and N x S. The interaction between Mo x P, as determined by Vistoso, Alfaro, and Mora (Citation2012) showed that both the addition of P or Mo increased the Mo accumulation by white clover. The antagonism for N x S was specific for the third grass cut, whereas the N x S interaction in the first grass cut was synergistic (Kowalenko Citation2004). According to Aulakh and Malhi (Citation2005), such seasonal variations have also been observed for the N x P interaction in pumpkin and N x K in rice, and they have suggested synergic interactions in high yielding seasons and antagonisms at lower yielding seasons. Knowledge of these antagonisms is relevant to estimate the amount of fertilizers to be used. Therefore, it is relevant to note that most of the antagonisms involve interactions between micronutrients and rarely between macronutrients ().
Table 8. Example of a negative interaction: Antagonism. The yield* is given as function of the application of nutrients.
For some combinations of nutrients, various types of interactions were found, such as for Zn x P (synergism, Liebig-synergism, zero-interaction) (). also shows that for a large number of nutrient combinations, no interactions have been reported. Remarkably, interactions of nutrients with Ca, Mg, and S are rare, as was previously noted by Aulakh and Malhi (Citation2005). No studies were found that show interactions for the cations: K x Zn, K x Cu, Fe x Ca, Mg x Ca, Mg x Cu, Ca x Cu, and Mg x Mn (). Also no interactions were found for the micronutrients Cl and Ni.
Table 9. Number of interactions assessed in this review.
Besides interactions between nutrients that affect yield, nutrients also can have an effect on the content or uptake of other nutrients. For example, the effect of K x Mg interaction often has been studied because nutrient content is relevant for animal feed. Optimal growth of ryegrass is reached at a Mg content of 1 g kg−1 dw (dry weight) in ryegrass (Smith, Cornforth, and Henderson Citation1985), but lactating cows require animal feed with a Mg content of 1.6–2.4 g kg−1 dw (Suttle and Underwood Citation2010). Supply of K had a negative effect on the Mg content of crops, but the K content of crops was not affected (Bedi and Sekhon Citation1977; Bolton and Penny Citation1968; Ohno and Grunes Citation1985; Ologunde and Sorensen Citation1982), or even increased (Narwal, Kumar, and Singh Citation1985). High ryegrass yields, in combination with high Mg content in grass (2.5 g Mg kg−1 dw), are obtained in practice by balancing limited levels of K with increased levels of Mg (Reijneveld et al. Citation2014).
Summarizing, our systematic assessment shows that with the exception of a limited number of studies for N x S and Mg x K, interactions between macronutrients are synergistic or zero-interaction. On the other hand, rather unpredictable large yield responses result from resolving nutrient deficiencies, Liebig-type synergisms, which in most cases involve Fe, Cu, Mn, and Zn. For these cations, synergisms among each other is rare. In most cases, antagonism does involve one of the cations Ca, Mg, Fe, Mn, Zn, or Cu. shows that only a limited number of studies have reported interactions for each combination of nutrients. Such a small number of studies does not allow disentangling the variations due to crop species, and other variables such as soil conditions. Additionally, in some studies, a negative effect of the application of nutrients on yield was found (). As this cannot be the intention of the use of fertilizers, these studies were assigned to a separate category (see Appendix 1). However, they can provide information about specific nutrient interactions and are, therefore, discussed after the following section. Finally, in some studies, no significant effect of nutrients on yield was obtained, and therefore, no interaction effects can be derived ().
Relationship between uptake of micronutrients and application of macronutrients
Various authors have investigated if an increase in yield, especially via N fertilization, will lead to a dilution of nutrients in crops (Rengel, Batten, and Crowley Citation1999), and a possible decrease of nutrient content in food and crops has been discussed in various publications (Fan et al. Citation2008). However, in field experiments (), the increased yield via N fertilizer does not lead to a significant change of the nutrient content in the grain of corn, wheat, and rice, likely due to the adequate soil supply of these nutrients (Mg, Zn, Cu, Fe and Mn in ). The Zn content in the grain decreased in three studies, whereas it increased in two. The use of N fertilizer increased the S content in sorghum (by 9%) (Kaufman et al. Citation2013), wheat (McGrath Citation1985), and rice (Marr, Batten, and Lewin Citation1999), increasing the total accumulation by crops per unit area. Similarly, an increase in yield via various fertilizers does not lead to large increases or decreases in nutrient concentrations in potato (White et al. Citation2009) or corn (Heckman et al. Citation2003). The content of nutrients in brown rice was not affected by S treatment for S-deficient soils (Juliano et al. Citation1987). A decrease in Zn content of wheat grain was found, however, in a field without Zn deficiency, as a function of P fertilizer input (Zhang et al. Citation2012), whereas an increase in the Zn content of corn occurred if Zn was deficient (Friesen, Juo, and Miller Citation1980), revealing the complexity of the edaphic and biological system (Alloway 2008).
Table 10. Effects of macronutrient fertilizer on yield and content of micronutrients in the graina. In case of a significant effect of the fertilizer on the nutrient concentration, the nutrient concentration at the lowest and highest fertilizer input is given; otherwise the average content is given.
Assuming a rather constant micronutrient content, it is possible to estimate the crop nutrient accumulation from the yield. The nutrient contents in , however, are for the grain and not for the whole crop. Other references, as given in , provided the nutrient accumulation by crops, which, when no crop residues are left behind, is similar to the nutrient removal from the field. In , the fertilization rates used in various studies are presented, together with the recommended fertilization rates under deficiency. Comparison of with shows that the fertilization rates of micronutrients are rather high compared to the annual nutrient removal, as these fertilization rates are part of a strategy in which fertilization is performed only when there are indications for deficiency, based on low concentrations in soil or crop, or visual symptoms. Such a high dose is assumed to suffice for several years. Only boron is used annually, or more frequently, as it is easily leached in areas of high rainfall (Alloway 2008). The strategy to create a large stock in the soil, which in case of Cu, Mn, and Zn, is likely to be effective, as the nutrients are hardly lost by leaching and are applied only if they favorably affect yield. An annual-application approach is also applied, for example, in Australia with single superphosphate that contains about 600 mg Zn kg−1 fertilizer from rock phosphate and suffices to sustain wheat grain production (Brennan Citation2001). The use of compound NPK fertilizers containing 1% Zn is promoted in Turkey (Cakmak Citation2008), and many fertilizer blends are sold that contain micronutrients.
Table 11. Annual nutrient uptake (kg/ha/year) for some crops.
Table 12. Fertilization rates of micronutrients (kg/ha) for deficient situations for different countries.
Studies about the effects of an annual fertilization with micronutrients to compensate for the removal by crop are rare. In a study in which the effects of P x Zn interactions on corn have been investigated over a period of 25 years in a calcareous soil (Mallarino and Webb Citation1995), there was no induction of Zn deficiency by long-term high P fertilization, with zero-interaction between P and Zn. Brennan (Citation2001) studied the effectiveness of Zn applications on the yield of wheat in sandy acidic soils. Zinc applications performed in 1983 were still effective in 1996, although the Zn application was 50% less effective in terms of yield response after 13 years as compared to Zn applied in 1996 (Brennan Citation2001). The total Zn removal from the field by wheat grain in 13 years was 7% of the applied 3 kg Zn ha−1.
Summarizing, fertilizer application recommendations that considered a balanced application of nutrients have been reported, but without consideration of antagonism and synergism. The micronutrients content in plants remain relatively constant with increasing yield and increased application of macronutrients if sufficient micronutrients are available to the crop.
Specific mechanisms for antagonistic or synergistic responses to nutrients
In most cases, the authors of the studies listed in Appendix 1 did not assign specific mechanisms for the nutrient interactions. According to Pan (Citation2012), non-specific interactions are typical for interactions with N (e.g., N x P, N x S), as they influence all stages of plant growth, and too many associated processes. Specific nutrient interactions have been classified as soil, rhizosphere, and plant processes (Zhang, Shen, and Zhu Citation2006), with the rhizosphere processes classified as cation-cation, cation-anion, or anion-anion interactions (Pan Citation2012). The antagonisms () between the cations, Cu, Fe, Mg, Mn, and Zn suggest that with cation-cation interactions, similar uptake mechanisms and competition between the cations might explain these effects. There are also some cation-anion interactions that might be specific, notably P x Zn interaction. One mechanism is that the control of P uptake by a plant is lost under Zn deficiency, as the expression of high-affinity P transporter proteins is linked to the Zn status of plants, suggesting in turn that complex plant-specific genetic and membrane transport processes are relevant for the P x Zn interaction (Bouain et al. Citation2014; Huang et al. Citation2000). Below we attempt to relate the important nutrient interactions in to specific mechanisms.
Preferential transport of nutrients
Nutrient uptake occurs by proteins embedded in root membranes that catalyze the transport of nutrients across the membrane. Similar cations and similar anions compete for binding to specific carrier proteins, whereas the uptake of cations versus anions occurs through different transport proteins (). Some of the identified plasma membrane transporters seem to be specific for nutrients, and others are less specific. The molecular mechanism of Mg uptake is understood poorly, and, therefore, no plasma membrane transporters for Mg are listed in .
Table 13. Plasma membrane transporters for nutrients.
The transport proteins are unable to differentiate effectively between similar ions such as: potassium (K+) and rubidium (Rb+) (White Citation2012), sulfate (SO42-) and selenate (SeO42-) (White et al. Citation2007, Citation2004), sulfate (SO42-) and molybdate (MoO42-) (Fitzpatrick, Tyerman, and Kaiser Citation2008; Shinmachi et al. Citation2010), phosphate (PO43-) and arsenate (AsO43-) (White Citation2012), suggesting that the selectivity of some transport proteins in the plasma membrane of root cells is based partly on the physicochemical similarities between ions (White Citation2012). Indeed, some of the plasma membrane transporters families in are able to transport various nutrients, an action that might specifically explain the antagonism on yield () between nutrients.
Chaudhry et al. (Citation1973) reported an antagonistic response on rice yield of the Cu x Zn interaction, and a negative effect of Zn on Cu uptake by rice plants occurred if Cu in the plant was deficient. As Cu and Zn have two plasma membrane transporters in common (P1B-Zn-ATPases and/or ZIP), these findings suggest that competition at the common plasma membrane transporters might be relevant in case of an antagonism. In contrast, additive (Agarwala et al. Citation1995) and synergistic responses (Chaudhry and Loneragan Citation1970; Khurana and Chatterjee Citation2000) were reported for Cu x Zn in combination with a positive effect of Zn on Cu uptake and vice versa, in case of deficient and adequate Cu and Zn levels in the growing medium. The zero-interaction and synergistic crop responses show that competition of Cu and Zn at the common plasma membrane transporter is outweighed by other processes if Cu and Zn levels in the growing medium are deficient or adequate.
The crop yields suggest that competition on plasma membrane is not likely to affect a major interaction between Mo x S (), for which we found one study showing synergism (Sims, Leggett, and Pal Citation1979), one zero-interaction (Olsen and Watanabe Citation1979), and another no effect (Dhankar et al. Citation1996). None of the studies showed antagonism for Mo x S, probably because the nutrients contents were not deficient.
Ammonium has a negative effect on K uptake via K-channels (Hoopen et al. Citation2010), but K does not have the same effect on ammonium uptake via ammonium transporters (White Citation2012). While a positive N x K interaction is reported in many experiments, the interaction is found to be very complex, depending on the form of N and with contradictory findings (Zhang et al. Citation2010), and therefore cannot simply be explained by competition at the plasma membrane level.
The antagonism between Mn x Fe was noticed in three studies (Bansal, Chahal, and Nayyar Citation1999; Ghasemi-Fasaei and Ronaghi Citation2008; Kobraee and Shamsi Citation2011), and these two nutrients have one plasma membrane transporter in common (NRAMP). In two of these studies, there is a negative effect of Fe on Mn uptake (Bansal, Chahal, and Nayyar Citation1999; Ghasemi-Fasaei and Ronaghi Citation2008), and in only one study (Kobraee and Shamsi Citation2011), there is a negative effect of Mn on Fe uptake. In a hydroponic study, a strong effect of solution Fe concentration on Mn accumulation by soybean occurred, but no effect of solution Mn concentration on Fe uptake occurred (Heenan and Campbell Citation1983). Besides affecting Mn uptake at the common plasma membrane transporter, Fe has several other plasma membrane transporters, but other mechanisms also play a role in the antagonism between Mn and Fe.
Effect of nutrients on root reductase activity and phytosiderophore production
The activity of the reductase enzymes and phytosiderophore (“plant iron carrier”: class of chelating compounds, common in grasses, that sequester iron) might be the mechanism that explains the synergistic interactions between Fe x N, Fe x P, P x Zn, Cu x Fe; Cu x P; and B x Zn (). The positive influence of macronutrients on root reductase activity and phytosiderophore production might help to explain the positive responses of crops to macronutrients even under Fe or Zn deficiencies.
Iron deficiency by plants is caused by its low availability in soils, especially alkaline and calcareous soils, where the availability of other metallic micronutrients, specifically Zn, is also low. To mobilize Fe in the soil for uptake, grasses produce phytosiderophores (plant iron carriers) (strategy II), and other plants (dicots and non-grass monocots) produce reductases capable of reduction of Fe(III) to Fe(II) that can then be taken up by plants (strategy I). The release of phytosiderophores also can be induced by Zn deficiency (Aciksoz et al. Citation2011), and they also can mobilize other metals (Zn, Cu) (Römheld Citation1991). Various Fe-reducing substances, phenolics and carboxylates, can be produced by plants to increase the Fe availability (White Citation2012). Root ferric chelate reductase for instance is determined by Fe-deficiency but also can be regulated by Cu status in strawberry plants (Mukherjee et al. Citation2006; Pestana et al. Citation2013). Iron(III)-phytosiderophores are taken up by roots via yellow stripe1 (YS1) transporters (Schulz Citation2010), and plants such as peanut that cannot produce phytosiderophores can take up iron(III)-phytosiderophores. Corn in combination with peanut induces the production of phytosiderophores by corn and explains the positive effect of intercropping corn with peanut (Xiong et al. Citation2013). Nitrogen and S fertilization also may catalyze higher production of phytosiderophores and have been reported in soil and field experiments to increase Zn and Fe content in wheat (Kutman, Yildiz, and Cakmak Citation2011; Shi et al. Citation2010), Fe content in a Fe-deficient wheat plant in hydroponic experiments (Aciksoz et al. Citation2011) (Zuchi, Cesco, and Astolfi Citation2012), and Fe content in a pot experiment using brown rice in soil (Wu, Lu, and Hu Citation2014).
Antagonisms between Fe x Mn and Fe x Zn were found (), which also might be related to ferric-chelate reductase activity, as it has been shown that the ferric-chelate reductase activity of various plants, for example, alfalfa (Barton et al. Citation2000), sugar beet (Chang et al. Citation2003), cucumber (Lucena et al. Citation2003), and cowpea and bean (Dimkpa et al. Citation2015, Citation2008), can be inhibited by the high availability of metals.
Summarizing, although it has been a subject for research only recently (Keuskamp et al. Citation2015), the effects of nutrients on the reductase enzymes or phytosiderophore production might be a mechanism for interactions between macronutrients and Fe, Zn, and Cu.
Management strategies
Identifying synergisms is the most obvious management strategy to improve the response to fertilizers. Liebig-type synergisms are stimulated by solving nutrient deficiencies. The example in shows that nutrient deficiencies are not always better identified by looking only at the response to a deficient nutrient, but by including a combination of nutrients. Synergisms only can be identified using different fertilizers, combinations, and reference treatments. It is also expected that the improvement of various agronomic factors (e.g., water, weed, tillage, cultivar) will improve the responses to fertilizers (Ghosh et al. Citation2002).
Crop and plant ecophysiological processes inherit various opportunities to overcome nutrient antagonisms while stimulating synergisms due to 1) different nutrient requirements during the season, 2) different uptake routes for nutrients (leaf, roots), and 3) effects of different plants (intercropping). According to Aulakh and Malhi (Citation2005), the synergism between N x K can be exploited by a combined application throughout the season. This combined application corresponds with the enhancement of the N x S synergism for teff by a split application of combined N and S (Habtegebrial and Singh Citation2006), and with high yields in tomato using split application of NK fertilizers within the growing season on sandy soils (Locascio et al. Citation1997).
While many synergisms have been identified between nutrients applied via the soil, only a few synergistic responses have been reported using other application methods. Synergism between a nutrient application via soil N fertilization and foliar Fe fertilization was reported for soybean (Caliskan et al. Citation2008), and synergism between foliar fertilizers Mn x Mo was found on canola (Brennan and Bolland Citation2011). Besides soil (possible for all nutrients) and foliar fertilization (possible for B, Ca, Cu, Fe, Mn, Mo, N, Ni, S, Zn (Fageria et al. Citation2009)), nutrients can be supplied with the seed (e.g., Mo) (Draycott and Christenson Citation2003; Fageria et al. Citation2009) or by irrigation (which is mainly though the soil). A combination of seed, soil, or foliar applications of fertilizers might initiate an effective strategy to obtain a more efficient use of fertilizers and overcome antagonisms while enhancing synergism.
Nutrient combinations that show an antagonistic response when applied together might be delivered via different routes, whereas a synergistic response can be exploited best when nutrients are added together. An example of synergism is the combination of Fe foliar fertilizer with urea, which stimulates the uptake of Fe via wheat leaf penetration (Aciksoz et al. Citation2014) similar to the synergism between urea and Zn or Mn in foliar fertilization (Yassen, Abou El-Nour, and Shedeed Citation2010). Foliar fertilization of S alone did not increase soybean grain yield, whereas soybean responded positively if S was applied in a mixture with NPK (Garcia and Hanway Citation1976) suggesting a synergism in which nutrients have to be applied together. However, either soil or foliar applications of Mn were not effective to correct Fe-induced Mn deficiency in bean (Moosavi and Ronaghi Citation2010), and soil application of Mn was not effective to correct Mn deficiency due to soil or foliar application of Fe in chickpea (Ghasemi-Fasaei et al. Citation2005) or wheat (Ghasemi-Fasaei and Ronaghi Citation2008). These complex responses reveal that preventing antagonism and creating synergism is not straightforward and calls for an in-depth understanding of plant physiological processes (Fageria et al. Citation2009; Pandey, Krishnapriya, and Bindraban Citation2014).
Synergisms may be stimulated using intercropping. The synergism between N x P on corn was increased by intercropping with Gliricidia sepium, a leguminous tree (Akinnifesi et al. Citation2007). As mentioned earlier, the increased production of phytosiderophores as a response to fertilization of one crop and the improved Fe nutrition by a second crop, might be a mechanism for synergistic responses due to intercropping (Xiong et al. Citation2013). Manganese deficiency of berseem clover in alkaline soils in NW India could be remedied by mixed cropping with other fodder crops including oat, ryegrass, or mustard, resulting in a higher yield of berseem clover (Arneja and Sadana Citation2012); and mixed cropping with barley, oat, and wheat increased the Fe content in peanut plants under peanut Fe-deficiency (Zuo and Zhang Citation2008). These studies show that deficiencies can be alleviated by intercropping, and by doing so, these crops may show a higher response to fertilizers, although this possibility still has to be verified.
Conclusions
There is a need to increase the fertilizer use efficiency, that is, to obtain more yield per unit of fertilizer applied, to contribute to sustainable agriculture. Interactions among nutrients on nutrient uptake and accumulation and crop yield, including zero-interaction, synergism, or antagonism, reinforce balancing the composition, amount, timing, and mode of delivery of fertilizers to plants and soil, thereby aiming to overcome antagonism and stimulate synergism.
This review provides an overview on synergistic and antagonistic nutrient interactions on crop yield and sets the stage for delineating fertilizer strategies in terms of fertilizer design and delivery. A total of 117 interactions between nutrients on crop yield have been identified in 94 publications: 43 synergistic (of which 21 were of special type Liebig- synergistic), 17 antagonistic, and 35 resulted in zero-interaction. In some studies, no significant yield responses were found to assess interaction (16), and seven negative results were reported that were difficult to explain.
Because nutrient interactions could be studied for a limited number of crops, nutrients, soil types, and climates, only general, rather than conclusive, findings can be reported, and these results do not allow for extrapolation to other production conditions:
– In cases where the availability of two nutrients can be characterized as deficient, increasing the availability of both nutrients often results in a large increase in yield. Identification of deficiencies and the use of optimal ratios between nutrients are therefore important in developing efficient fertilizer application schemes.
– Most macronutrients have synergistic interactions. Synergistic interactions between nutrients result in actual relative yields that are a factor 1–3 greater than yield predicted on the basis of individual nutrients. As macronutrients form the basis for fertilizer applications, it is worthwhile to take these synergistic interactions into account.
– Antagonisms and negative effects of nutrients often are related to the divalent cations that probably share similar uptake mechanisms. Strategies to overcome these problems might be to differentiate the fertilization of these nutrients between soil and foliar applications. In some instances, this combined avenue has appeared effective, such as foliar fertilization of micronutrients in combination with urea. This differentiation however has rarely been studied for interacting nutrients.
Knowledge of nutrient interactions can guide fertilization trials and optimization of fertilization strategies for high yields and high nutrient use efficiencies. Stimulating reductase activity or phytosiderophore production, intercropping, and the use of micronutrients can be strategies to decrease deficiencies and increase responses to macronutrients, whereas a balanced fertilization of macronutrients can exploit the synergisms. Although the available data do not allow to disentangle the impact of confounding variables on reported yield responses to nutrient applications systematically, the information reveals several generic principles that can be accounted for as initial steps toward more balanced application of nutrients, and to explore the option of delivery of the nutrients to the plant through roots and leaves.
Acknowledgments
The authors acknowledge Prof. Dr. E. Hoffland and Dr. Ir. C.L. van Beek for their valuable comments on the subject. This work was supported by funding from the Virtual Fertilizer Research Center, Washington, D.C., USA.
References
- Abbasi, M. K., M. M. Tahir, W. Azam, Z. Abbas, and N. Rahim. 2012. Soybean yield and chemical composition in response to phosphorus-potassium nutrition in Kashmir. Agronomy Journal 104 (5):1476–84. doi:10.2134/agronj2011.0379.
- Aciksoz, S. B., L. Ozturk, O. O. Gokmen, V. Römheld, and I. Cakmak. 2011. Effect of nitrogen on root release of phytosiderophores and root uptake of Fe(III)-phytosiderophore in Fe-deficient wheat plants. Physiologia Plantarum 142 (3):287–96.
- Aciksoz, S. B., L. Ozturk, A. Yazici, and I. Cakmak. 2014. Inclusion of urea in a 59FeEDTA solution stimulated leaf penetration and translocation of 59Fe within wheat plants. Physiologia Plantarum 151 (3):348–57.
- Agarwala, S. C., and S. C. Mehrotra. 1984. Iron-magnesium antagonism in growth and metabolism of radish. Plant and Soil 80 (3):355–61.
- Agarwala, S. C., B. D. Nautiyal, C. Chatterjee, and N. Nautiyal. 1995. Variations in copper and zinc supply influence growth and activities of some enzymes in maize. Soil Science and Plant Nutrition 41 (2):329–35.
- Akinnifesi, F. K., W. Makumba, G. Sileshi, O. C. Ajayi, and D. Mweta. 2007. Synergistic effect of inorganic N and P fertilizers and organic inputs from Gliricidia sepium on productivity of intercropped maize in Southern Malawi. Plant and Soil 294 (1–2):203–17.
- Aktas, M., and F. Vanegmond. 1979. Effect of nitrate nutrition on iron utilization by an fe-efficient and an fe-inefficient soybean cultivar. Plant and Soil 51 (2):257–74.
- Alloway, B. J., and I. Cakmak. 2008. Zinc deficiency in wheat in Turkey. In Micronutrient deficiencies in global crop production, 181–200. Springer Netherlands.
- Antil, R. S., D. S. Yadav, Y. K. Yadav, and M. Singh. 1988. Nitrogen–Copper relationship in Raya (Brassica juncea Coss). Journal of the Indian Society of Soil Science 36:704–08.
- Arneja, S., and U. S. Sadana. 2012. Mixed cropping effects on yield, manganese influx, and manganese depletion in the rhizosphere of fodder crops grown in manganese-deficient soil. Communications in Soil Science and Plant Analysis 43 (3):533–40.
- Aulakh, M. S., and S. S. Malhi. 2005. Interactions of nitrogen with other nutrients and water: Effect on crop yield and quality, nutrient use efficiency, carbon sequestration, and environmental pollution. Advances in Agronomy 86:341–409.
- Awan, Z. I., and M. K. Abbasi. 2000. Interactive effect of phosphorus and copper on maize growth. Pakistan Journal of Agriculture Research 16 (2):105–08.
- Bailey, J. S. 1991. A re-examination of phosphorus-lime interactions in perennial ryegrass. Plant and Soil 135 (2):185–96.
- Balyan, D. V., and J. S. Dhankar. 1978. Effect of N, P and zinc on the yield of cauliflower. Indian Journal of Agricultural Science 58:947–48.
- Bansal, R. L., D. S. Chahal, and V. K. Nayyar. 1999. Effect of iron-manganese interaction on the yield and content of Fe and Mn in maize (zea mays L.). Acta Agronomica Hungarica 47 (1):19–25.
- Barben, S. A., B. G. Hopkins, V. D. Jolley, B. L. Webb, B. A. Nichols, and E. A. Buxton. 2011. Zinc, manganese and phosphorus interrelationships and their effects on iron and copper in chelator-buffered solution grown russet burbank potato. Journal of Plant Nutrition 34 (8):1144–63.
- Barton, L. L., G. V. Johnson, A. G. O’Nan, and B. M. Wagener. 2000. Inhibition of ferric chelate reductase in alfalfa roots by cobalt, nickel, chromium, and copper. Journal of Plant Nutrition 23 (11–12):1833–45.
- Bedi, A. S., and G. S. Sekhon. 1977. Effect of potassium and magnesium application to soils on the dry-matter yield and cation composition of maize. Journal of Agriculture Science., Cambridge. 88:753–58.
- Bindraban, P. S., C. O. Dimkpa, L. Nagarajan, A. Roy, and R. Rabbinge. 2015. Revisiting fertilisers and fertilisation strategies for improved nutrient uptake by plants. Biology and Fertility of Soils 51 (8):897–911.
- BMLFUW. 2006. Guidelines for appropriate fertilisation. Insttuctions for interpretation of soil investigations in agriculture. “Richtlinien für die Sachgerechte Düngung. Anleitung zur Interpretation von Bodenuntersuchungsergebnissen in der Landwirtschaft”. Wien: Bundesministerium für Land- und Forstwirtschaft.
- Bolton, J., and A. Penny. 1968. The effects of potassium and magnesium fertilizers on yield and composition of successive crops of ryegrass, clover, sugar beat, potatoes, kale and barley on sandy soil at Woburn. Journal of Agricultural Science 78:303–11.
- Bouain, N., Z. Shahzad, A. Rouached, G. A. Khan, P. Berthomieu, C. Abdelly, Y. Poirier, and H. Rouached. 2014. Phosphate and zinc transport and signalling in plants: Toward a better understanding of their homeostasis interaction. Journal of Experimental Botany 65 (20):5725–41.
- Brennan, R. F. 2001. Residual value of zinc fertiliser for production of wheat. Australian Journal of Experimental Agriculture 41 (4):541–47.
- Brennan, R. F., and M. D. A. Bolland. 2009. Comparing the nitrogen and potassium requirements of canola and wheat for yield and grain quality. Journal of Plant Nutrition 32 (12):2008–26.
- Brennan, R. F., and M. D. A. Bolland. 2011. Foliar spray experiments identify no boron deficiency but molybdenum and manganese deficiency for canola grain production on acidified sandy gravel soils in southwestern australia. Journal of Plant Nutrition 34 (8):1186–97.
- Bruns, H. A., and M. W. Ebelhar. 2006. Nutrient uptake of maize affected by nitrogen and potassium fertility in a humid subtropical environment. Communications in Soil Science and Plant Analysis 37 (1–2):275–93.
- Buah, S. S. J., J. M. Kombiok, and L. N. Abatania. 2012. Grain sorghum response to NPK fertilizer in the Guinea Savanna of Ghana. Journal of Crop Improvement 26 (1):101–15.
- Cakmak, I. 2008. Zinc deficiency in wheat in Turkey. In Micronutrient deficiencies in Global Crop Production, Ed. B. J. Alloway. Spinger Science + Business Media.
- Caliskan, S., I. Ozkaya, M. E. Caliskan, and M. Arslan. 2008. The effects of nitrogen and iron fertilization on growth, yield and fertilizer use efficiency of soybean in a Mediterranean-type soil. Field Crops Research 108 (2):126–32.
- Chang, Y. C., M. Zouari, Y. Gogorcena, J. J. Lucena, and J. Abadia. 2003. Effects of cadmium and lead on ferric chelate reductase activities in sugar beet roots. Plant Physiology and Biochemistry 41 (11–12):999–1005.
- Chaudhry, F. M., and J. F. Loneragan. 1970. Effects of nitrogen, copper and zinc fertilizers on the copper and zinc nutrition of wheat plants. Australian Journal of Agricultural Research 21:865–79.
- Chaudhry, F. M., M. Sharif, A. Latif, and R. H. Qureshi. 1973. Zinc-copper antagonism in nutrition of rice (oryza-sativa l). Plant and Soil 38 (3):573–80.
- Cheshire, M. V., P. C. Dekock, and R. H. E. Inkson. 1967. Factors affecting copper content of oats grown in peat. Journal of the Science of Food and Agriculture 18 (4):156–60.
- Chien, S. H., L. I. Prochnow, and H. Cantarella. 2009. Recent developments of fertilizer production and use to improve nutrient efficiency and minimize environmental impacts. Advances in Agronomy 102:267–322.
- Ciampitti, I. A., and T. J. Vyn. 2013. Maize nutrient accumulation and partitioning in response to plant density and nitrogen rate: II. Calcium, magnesium, and micronutrients. Agronomy Journal 105 (6):1645–57.
- Daliparthy, J., A. V. Barker, and S. S. Mondal. 1994. Potassium fractions with other nutrients in crops. A review focussing on the tropics. Journal of Plant Nutrition 17 (11):1859–86.
- Das, K., R. Dang, T. N. Shivananda, and P. Sur. 2005. Interaction between phosphorus and zinc on the biomass yield and yield attributes of the medicinal plant stevia (Stevia rebaudiana). TheScientificWorldJournal 5:390–95.
- Dekock, P. C., M. V. Cheshire, and A. Hall. 1971. Comparison of the effect of phosphorus and nitrogen on copper-deficient and-sufficient oats. Journal of the Science of Food and Agriculture 22 (9):437–40.
- Dhankar, J. S., V. Kumar, P. S. Sangwan, and S. P. S. Karwasra. 1996. Effects of sulphur and molybdenum application on dry matter yield, and on concentration and total content of molybdenum in a raya crop (Brassica juncea Coss). Agrochimica 40 (5–6):247–56.
- Dias, M. A., and M. M. Oliveira. 1996. Effects of copper and nitrogen supply in rice. Agrochimica 40 (1):1–8.
- Dibb, D. W., and W. R. Thompson. 1985. Interaction of potassium with other nutrients. In Potassium in agriculture: Proceedings of an international symposium held 7-10 July in Atlanta, Georgia, ed. R. D. Munson, 515–33. Madison: Soil Science Society of America.
- Dimkpa, C. O., and P. S. Bindraban. 2016. Micronutrients fortification for efficient agronomic production. Agronomy for Sustainable Development 36:1–26.
- Dimkpa, C. O., J. E. McLean, D. W. Britt, and A. J. Anderson. 2015. Nano-CuO and interaction with nano-ZnO or soil bacterium provide evidence for the interference of nanoparticles in metal nutrition of plants. Ecotoxicology 24 (1):119–29.
- Dimkpa, C. O., A. Svatoš, D. Merten, G. Büchel, and E. Kothe. 2008. Hydroxamate siderophores produced by Streptomyces acidiscabies E13 bind nickel and promote growth in cowpea (Vigna unguiculata L.) under nickel stress. Canadian Journal of Microbiology 54 (3):163–72.
- Dogliotti, S., K. E. Giller, and M. K. Van Ittersum. 2014. Achieving global food security whilst reconciling demands on the environment: Report of the First International Conference on Global Food Security. Food Security 6 (2):299–302.
- Draycott, A. P., and D. R. Christenson. 2003. Nutrients for sugar beet production: Soil-plant relationships. Wallingford: CABI.
- Dwivedi, B. S., A. K. Shukla, V. K. Singh, and R. L. Yadav. 2003. Improving nitrogen and phosphorus use efficiencies through inclusion of forage cowpea in the rice-wheat systems in the Indo-Gangetic Plains of India. Field Crops Research 80 (3):167–93.
- Epstein, E., and A. J. Bloom. 2005. Mineral nutrition of plants: Principles and perspectives. Sunderland, MA: Sinauer.
- Evers, M. A. A., A. Postma, T. Van Dijk, W. Vergeer, and C. Wierda. 2000. Practice guide to fertilizer use “Praktijkgids bemesting”. Wageningen: Nutrienten Management Instituut.
- Fageria, N. K. 2014. Nitrogen management in crop production. Boca Raton, FL: CRC Press.
- Fageria, N. K., V. C. Baligar, and C. A. Jones. 2011. Growth and mineral nutrition of field crops. Boca Raton, FL: CRC.
- Fageria, N. K., V. C. Baligar, A. Moreira, and L. A. C. Moraes. 2013. Soil phosphorous influence on growth and nutrition of tropical legume cover crops in acidic soil. Communications in Soil Science and Plant Analysis 44 (22):3340–64.
- Fageria, N. K., M. P. B. Filho, A. Moreira, and C. M. Guimarães. 2009. Foliar fertilization of crop plants. Journal of Plant Nutrition 32 (6):1044–64.
- Fageria, N. K., A. Moreira, and A. M. Coelho. 2012. Nutrient uptake in dry bean genotypes. Communications in Soil Science and Plant Analysis 43 (17):2289–302.
- Fageria, N. K., and J. P. Oliveira. 2014. Nitrogen, phosphorus and potassium interactions in upland rice. Journal of Plant Nutrition 37 (10):1586–600.
- Fageria, V. D. 2001. Nutrient interactions in crop plants. Journal of Plant Nutrition 24 (8):1269–90.
- Fan, M. S., F. J. Zhao, S. J. Fairweather-Tait, P. R. Poulton, S. J. Dunham, and S. P. McGrath. 2008. Evidence of decreasing mineral density in wheat grain over the last 160 years. Journal of Trace Elements in Medicine and Biology 22 (4):315–24.
- Feil, B., S. B. Moser, S. Jampatong, and P. Stamp. 2005. Mineral composition of the grains of tropical maize varieties as affected by pre-anthesis drought and rate of nitrogen fertilization. Crop Science 45 (2):516–23.
- Fitzpatrick, K. L., S. D. Tyerman, and B. N. Kaiser. 2008. Molybdate transport through the plant sulfate transporter SHST1. FEBS Letters 582 (10):1508–13.
- Friesen, D. K., A. S. R. Juo, and M. H. Miller. 1980. Liming and lime-phosphorus-zinc interactions in 2 nigerian ultisols .1. interactions in the soil. Soil Science Society of America Journal 44 (6):1221–26.
- Garcia, R. L., and J. J. Hanway. 1976. Foliar fertilization of soybeans during the seed-filling period. Agronomy Journal 68 (4):653–57.
- Ghasemi-Fasaei, R., and A. Ronaghi. 2008. Interaction of iron with copper, zinc, and manganese in wheat as affected by iron and manganese in a calcareous soil. Journal of Plant Nutrition 31 (5):839–48.
- Ghasemi-Fasaei, R., A. Ronaghi, M. Maftoun, N. A. Karimian, and P. N. Soltanpour. 2005. Iron-manganese interaction in chickpea as affected by foliar and soil application of iron in a calcareous soil. Communications in Soil Science and Plant Analysis 36 (13–14):1717–25.
- Ghosh, P. K., R. H. Wanjari, K. G. Mandal, K. M. Hati, and K. K. Bandyopadhyay. 2002. Recent trends in inter-relationship of nutrients with various agronomic practices of field crops in India. Journal of Sustainable Agriculture 21 (1):47–78.
- Gianquinto, G., A. Abu-Rayyan, L. Di Tola, D. Piccotino, and B. Pezzarossa. 2000. Interaction effects of phosphorus and zinc on photosynthesis, growth and yield of dwarf bean grown in two environments. Plant and Soil 220 (1–2):219–28.
- Gunes, A., M. Alpaslan, and A. Inal. 1998. Critical nutrient concentrations and antagonistic and synergistic relationships among the nutrients of NFT-grown young tomato plants. Communications in Soil Science and Plant Analysis 21 (10):2035–47.
- Habtegebrial, K., and B. R. Singh. 2006. Effects of timing of nitrogen and sulphur fertilizers on yield, nitrogen, and sulphur contents of Tef (Eragrostis tef (Zucc.) Trotter). Nutrient Cycling in Agroecosystems 75 (1–3):213–22.
- Heckman, J. R. 2007. Sweet corn nutrient uptake and removal. HortTechnology 17 (1):82–86.
- Heckman, J. R., J. T. Sims, D. B. Beegle, F. J. Coale, S. J. Herbert, T. W. Bruulsema, and W. J. Bamka. 2003. Nutrient removal by corn grain harvest. Agronomy Journal 95 (3):587–91.
- Heenan, D. P., and L. C. Campbell. 1981. Influence of potassium and manganese on growth and uptake of magnesium by soybeans (Glycine max (L.) Merr. cv. Bragg). Plant and Soil 61 (3):447–56.
- Heenan, D. P., and L. C. Campbell. 1983. Manganese and iron interactions on their uptake and distribution in soybean (Glycine max (L.) Merr.). Plant and Soil 70 (3):317–26.
- Hocking, P. J., P. J. Randall, and A. Pinkerton. 1987. Sulphur nutrition of sunflower (Helianthus annuus) as affected by nitrogen supply: Effects on vegetative growth, the development of yield components, and seed yield and quality. Field Crops Research 16 (2):157–75.
- Hoeks, P., J. C. Van Middelkoop, A. P. Philipsen, B. Talens, D. W. Bussink, A. J. Bos, W. Van Dijk, J. J. Schröder, G. Abbink, and N. Van Eekeren. 2012. Fertilization advice “Bemestingsadvies”. Lelystad: Animal Sciences Group, Commissie Bemesting Grasland en Voedergewassen.
- Hoopen, F. T., T. A. Cuin, P. Pedas, J. N. Hegelund, S. Shabala, J. K. Schjoerring, and T. P. Jahn. 2010. Competition between uptake of ammonium and potassium in barley and arabidopsis roots: Molecular mechanisms and physiological consequences. Journal of Experimental Botany 61 (9):2303–15.
- Huang, C. Y., S. J. Barker, P. Langridge, F. W. Smith, and R. D. Graham. 2000. Zinc deficiency up-regulates expression of high-affinity phosphate transporter genes in both phosphate-sufficient and -deficient barley roots. Plant Physiology 124 (1):415–22.
- Husted, S., M. U. Thomsen, M. Mattsson, and J. Schjoerring. 2005. Influence of nitrogen and sulphur form on manganese acquisition by barley (Hordeum vulgare). Plant and Soil 268 (1–2):309–17.
- Imtiaz, M., B. Alloway, M. Memon, P. Khan, S. Siddiqui, M. Aslam, and S. Shah. 2006. Zinc tolerance in wheat cultivars as affected by varying levels of phosphorus. Communications in Soil Science and Plant Analysis 37 (11–12):1689–702.
- Islam, M., S. Mohsan, S. Ali, R. Khalid, and S. Afzal. 2012. Response of chickpea to various levels of phosphorus and sulphur under rainfed conditions in pakistan. Romanian Agricultural Research 29:175–83.
- Izsáki, Z. 2009. Effect of nitrogen supply on nutritional status of maize. Communications in Soil Science and Plant Analysis 40 (1–6):960–73.
- Izsáki, Z. 2014. Effects of phosphorus supplies on the nutritional status of maize (Zea mays L.). Communications in Soil Science and Plant Analysis 45 (4):516–29.
- Jackson, G. D. 2000. Effects of nitrogen and sulfur on canola yield and nutrient uptake. Agronomy Journal 92 (4):644–49.
- Jakobsen, S. T. 1993. Interaction between plant nutrients .3. antagonism between potassium, magnesium and calcium. Acta Agriculturae Scandinavica Section B-Soil and Plant Science 43 (1):1–5.
- Johansson, O. A. H., and J. M. Hahlin. 1977. Potassium/Magnesium balance in soil for maximum yield. Proceedings, International Seminar on soil environment and fertility management in intesnive agriculture, Japan.1977.
- Jones, G. D., J. A. Lutz Jr., and T. J. Smith. 1977. Effects of phosphorus and potassium on soybean nodules and seed yield. Agronomy Journal 69 (6):1003–06.
- Juliano, B. O., M. G. B. Ibabao, C. M. Perez, R. B. Clark, J. W. Maranville, C. P. Mamaril, N. H. Choudhury, C. J. S. Momuat, and I. T. Corpuz. 1987. Effect of soil sulfur deficiency on sulfur amino-acids and elements in brown rice. Cereal Chemistry 64 (1):27–30.
- Kaufman, R. C., J. D. Wilson, S. R. Bean, D. R. Presley, H. Blanco-Canqui, and M. Mikha. 2013. Effect of nitrogen fertilization and cover cropping systems on sorghum grain characteristics. Journal of Agricultural and Food Chemistry 61 (24):5715–19.
- Keeratikasikorn, P., R. W. Bell, and J. F. Loneragan. 1991. Response of 2 peanut (arachis-hypogaea l) cultivars to boron and calcium. Plant and Soil 138 (1):61–66.
- Keuskamp, D. H., R. Kimber, P. Bindraban, C. Dimkpa, and W. D. C. Schenkeveld. 2015. Plant exudates for nutrient uptake. report 2015/4, VFRC, Washington, DC.
- Khurana, N., and C. Chatterjee. 2000. Deficiency of manganese is alleviated more by low zinc than low copper in wheat. Communications in Soil Science and Plant Analysis 31 (15–16):2617–25.
- Khurana, N., and C. Chatterjee. 2002. Low sulfur alters boron metabolism of mustard. Journal of Plant Nutrition 25 (3):679–87.
- Kobraee, S., and K. Shamsi. 2011. Effects of Zn, Fe and Mn on soybean production. Ecology, Environment and Conservation 17 (2):191–96.
- Kowalenko, C. G. 2004. Variations in within-season nitrogen and sulfur interaction effects on forage grass response to combinations of nitrogen, sulfur, and boron applications. Communications in Soil Science and Plant Analysis 35 (5–6):759–80.
- Kumar, V., V. S. Ahlawat, and R. S. Antil. 1985. Interactions of nitrogen and zinc in pearl-millet .1. effect of nitrogen and zinc levels on dry-matter yield and concentration and uptake of nitrogen and zinc in pearl-millet. Soil Science 139 (4):351–56.
- Kumar, V., B. K. Bhatia, and U. C. Shukla. 1981. Magnesium and zinc relationship in relation to dry-matter yield and the concentration and uptake of nutrients in wheat. Soil Science 131 (3):151–55.
- Kumar, V., and M. Singh. 1980. Interactions of sulfur, phosphorus, and molybdenum in relation to uptake and utilization of phosphorus by soybean. Soil Science 130 (1):26–31.
- Kumar, V., D. V. Yadav, and D. S. Yadav. 1990. Effects of nitrogen-sources and copper levels on yield, nitrogen and copper contents of wheat (triticum-aestivum l). Plant and Soil 126 (1):79–83.
- Kutman, U. B., B. Yildiz, and I. Cakmak. 2011. Effect of nitrogen on uptake, remobilization and partitioning of zinc and iron throughout the development of durum wheat. Plant and Soil 342 (1–2):149–64.
- Laboski, C. A. M., and J. B. Peters. 2012. Nutrient application guidelines for field, vegetable, and fruit crops in Wisconsin. Madison: Cooperative Extension of the University of Wisconsin-Extension.
- Landon, J. R. 1991. Booker tropical soil manual: A handbook for soil survey and agricultural land evaluation in the tropics and subtropics. Harlow: Longman.
- Landwirtschaftskammer Niedersachsen. 2008. Guidelines for fertilization in Lower Saxony. Extract from the fertilizer guidelines, micronutrients boron, manganese, copper and zinc “Richtwerte für die Düngung in Niedersachsen. Auszug aus den Düngungsrichtlinien, Mikronährstoffe Bor, Mangan, Kupfer und Zink”. Hannover, Germany: Landwirtschaftskammer Niedersachsen.
- Le Mare, P. H. 1977. Experiments on effects of phosphorus on the manganese nutrition of plants - II. Interactions of phosphorus, calcium and manganese in cotton grown with nutrient solutions. Plant and Soil 47 (3):607–20.
- Liu, H., C. Hu, X. Sun, Q. Tan, Z. Nie, and X. Hu. 2010. Interactive effects of molybdenum and phosphorus fertilizers on photosynthetic characteristics of seedlings and grain yield of Brassica napus. Plant and Soil 326 (1):345–53.
- Lobell, D. B., K. G. Cassman, and C. B. Field. 2009. Crop yield gaps: Their importance, magnitudes, and causes. Annual Review of Environment and Resources 34:179–204.
- Locascio, S. J., G. J. Hochmuth, F. M. Rhoads, S. M. Olson, A. G. Smajstrla, and E. A. Hanlon. 1997. Nitrogen and potassium application scheduling effects on drip-irrigated tomato yield and leaf tissue analysis. HortScience 32 (2):230–35.
- Lombin, G., and L. Singh. 1986. Fertilizer responses of groundnuts (arachis-hypogae l) under continuous intensive cultivation in the nigerian savannah. Fertilizer Research 10 (1):43–58.
- Lucena, C., I. Montilla, F. J. Romera, and E. Alcantara. 2003. Effects of several metals on both Fe(III)- and Cu(II)-reduction by roots of Fe-deficient cucumber plants. Journal of Plant Nutrition 26 (10–11):2069–79.
- Mallarino, A. P., and J. R. Webb. 1995. Long-term evaluation of phosphorus and zinc interactions in corn. Journal of Production Agriculture 8 (1):52–55.
- Mandal, B., S. Pal, and L. N. Mandal. 1998. Effect of molybdenum, phosphorus, and lime application to acid soils on dry matter yield and molybdenum nutrition of lentil. Journal of Plant Nutrition 21 (1):139–47.
- Marr, K. M., G. D. Batten, and L. G. Lewin. 1999. The effect of nitrogen fertiliser on yield, nitrogen and mineral elements in Australian brown rice. Australian Journal of Experimental Agriculture 39 (7):873–80.
- Martens, D. C., and D. T. Westermann. 1991. Fertilizer applications for correcting micronutrients in agriculture. In Micronutrients in agriculture, eds. J. J. Mortvedt, F. R. Cox, and L. M. Shuman, 2nd, Soil Science Society of America book series;no. 4, Madison: Soil Science Society of America.
- McGrath, S. P. 1985. The effects of increasing yields on the macroelement and microelement concentrations and offtakes in the grain of winter-wheat. Journal of the Science of Food and Agriculture 36 (11):1073–83.
- McGrath, S. P., and F. J. Zhao. 1996. Sulphur uptake, yield responses and the interactions between nitrogen and sulphur in winter oilseed rape (Brassica napus). Journal of Agricultural Science 126:53–62.
- Mengel, K., E. A. Kirkby, H. Kosegarten, and T. Appel. 2001. Principles of plant nutrition. Dordrecht: Kluwer Academic Publishers.
- Mohr, R. M., C. A. Grant, W. E. May, and F. C. Stevenson. 2007. The influence of nitrogen, phosphorus and potash fertilizer application on oat yield and quality. Canadian Journal of Soil Science 87 (4):459–68.
- Moinuddin, and S. Umar. 2004. Influence of combined application of potassium and sulfur on yield, quality, and storage behavior of potato. Communications in Soil Science and Plant Analysis 35 (7–8):1047–60.
- Moosavi, A. A., and A. Ronaghi. 2010. Growth and iron-manganese relationships in dry bean as affected by foliar and soil applications of iron and manganese in a calcareous soil. Journal of Plant Nutrition 33 (9):1353–65.
- Moraghan, J. T. 1992. Iron-manganese relationships in white lupine grown on a calciaquol. Soil Science Society of America Journal 56 (2):471–75.
- Mortvedt, J. J., F. R. Cox, and L. M. Shuman. 1991. Micronutrients in agriculture. Madison: Soil Science Society of America.
- Mukherjee, I., N. H. Campbell, J. S. Ash, and E. L. Connolly. 2006. Expression profiling of the Arabidopsis ferric chelate reductase (FRO) gene family reveals differential regulation by iron and copper. Planta 223 (6):1178–90.
- Nair, K. P. P., and G. R. Babu. 1975. Zinc-phosphorus-iron interaction studies in maize. Plant and Soil 42 (3):517–36.
- Narwal, R. P., V. Kumar, and J. P. Singh. 1985. Potassium and magnesium relationship in cowpea (vigna-unguiculata (l) walp). Plant and Soil 86 (1):129–34.
- Nautiyal, N., and C. Chatterjee. 2002. Copper-manganese interaction in cauliflower. Journal of Plant Nutrition 25 (8):1701–07.
- Nichols, B. A., B. G. Hopkins, D. Von Jolley, B. L. Webb, B. G. Greenwood, and J. R. Buck. 2012. Phosphorus and zinc interactions and their relationships with other nutrients in maize grown in chelator-buffered nutrient solution. Journal of Plant Nutrition 35 (1):123–41.
- Obiefuna, J. C., P. K. Majumder, and A. C. Ucheagwu. 1987. Fertilizer rates for increased pineapple production in the tropical ferrallitic soils of South Western Nigeria. Fertilizer Research 12 (2):99–105.
- Ohno, T., and D. L. Grunes. 1985. Potassium- magnesium interactions affecting nutrient uptake by wheat forage. Soil Science Society of America Journal 49 (3):685–90.
- Ologunde, O. O., and R. C. Sorensen. 1982. Influence of concentrations of K and Mg in nutrient solution on sorghum. Agronomy Journal 74 (1):41–46.
- Olsen, S. R., and F. S. Watanabe. 1979. Interaction of added gypsum in alkaline soils with uptake of iron, molybdenum, manganese, and zinc by sorghum. Soil Science Society of America Journal 43 (1):125–30.
- Omar, M. A., and T. El-Kobbia. 1965. Some observations on the interrelationship of potassium and magnesium. Journal of Soil Science of the United Arab Republic 5:43–49.
- Pagani, A., H. E. Echeverría, F. H. Andrade, and H. R. Sainz Rozas. 2012. Effects of nitrogen and sulfur application on grain yield, nutrient accumulation, and harvest indexes in maize. Journal of Plant Nutrition 35 (7):1080–97.
- Pan, W. L. 2012. Nutrient interactions in soil fertility and plant nutrition. In Handbook of soil sciences: Resource management and environmental impacts, eds. P. M. Huang, and Y. Li. Boca Raton, Fla: CRC.
- Pandey, R., V. Krishnapriya, and P. S. Bindraban. 2014. Biochemical nutrient pathways in plants applied as foliar spray: Phosphorus and Iron. Washington, DC, USA: Virtual Fertilizer Research Center (VFRC).
- Payne, G. G., M. E. Sumner, and C. O. Plank. 1986. Yield and composition of soybeans as influenced by soil pH, phosphorus, zinc, and copper. Communications in Soil Science & Plant Analysis 17 (3):257–73.
- Pedas, P., S. Husted, K. Skytte, and J. K. Schjoerring. 2011. Elevated phosphorus impedes manganese acquisition by barley plants. Frontiers in Plant Science 2 (Aug), Article 37.
- Pestana, M., P. J. Correia, T. Saavedra, F. Gama, S. Dandlen, G. Nolasco, and A. De Varennes. 2013. Root ferric chelate reductase is regulated by iron and copper in strawberry plants. Journal of Plant Nutrition 36 (13):2035–47.
- Petrie, S. E., and T. L. Jackson. 1984. Effects of nitrogen-fertilization on manganese concentration and yield of barley and oats. Soil Science Society of America Journal 48 (2):319–22.
- Ray, D. K., N. D. Mueller, P. C. West, and J. A. Foley. 2013. Yield trends are insufficient to double global crop production by 2050. PLoS ONE 8 (6):e66428.
- Redondo-Nieto, M., A. R. Wilmot, A. El-Hamdaoui, I. Bonilla, and L. Bolaños. 2003. Relationship between boron and calcium in the N2-fixing legume-rhizobia symbiosis. Plant, Cell and Environment 26 (11):1905–15.
- Rehm, G. W., and R. C. Sorensen. 1985. Effects of potassium and magnesium for corn grown on irrigated sandy soil. Soil Science Society of America Journal 49 (6):1446–50.
- Reijneveld, J. A., G. W. Abbink, A. J. Termorshuizen, and O. Oenema. 2014. Relationships between soil fertility, herbage quality and manure composition on grassland-based dairy farms. European Journal of Agronomy 56:9–18.
- Rengel, Z., G. D. Batten, and D. E. Crowley. 1999. Agronomic approaches for improving the micronutrient density in edible portions of field crops. Field Crops Research 60 (1–2):27–40.
- Rhoads, F. M., and S. M. Olson. 2001. Cabbage response to sulfur source and nitrogen rate. Annual Proceedings Soil and Crop Science Society of Florida 60:37–40.
- Riedell, W. E. 2010. Mineral-nutrient synergism and dilution responses to nitrogen fertilizer in field-grown maize. Journal of Plant Nutrition and Soil Science 173 (6):869–74.
- Robson, A. D., and M. G. Pitman. 1983. Interactions between nutrients in higher plants. In Inorganic plant nutrition, eds. A. Laeuchli, and R. L. Bieleski, 147–80. Berlin: Springer.
- Römheld, V. 1991. The role of phytosiderophores in acquisition of iron and other micronutrients in graminaceous species: An ecological approach. Plant and Soil 130 (1–2):127–34.
- Rotaru, V., and T. R. Sinclair. 2009. Interactive influence of phosphorus and iron on nitrogen fixation by soybean. Environmental and Experimental Botany 66 (1):94–99.
- Roy, R. N., A. Finck, G. J. Blair, and H. L. S. Tandon. 2006. Plant nutrition for food security. A Guide for Integrated Nutrient Management. FAO Fertilizer and Plant Nutrition Bulletin 16. Rome, Italy: FAO.
- Roy, R. N., and B. C. Wright. 1973. Sorghum growth and nutrient uptake in relation to soil fertility .1. dry-matter accumulation patterns, yield, and n-content of grain. Agronomy Journal 65 (5):709–11.
- Rubio, G., J. Zhu, and J. P. Lynch. 2003. A critical test of the two prevailing theories of plant response to nutrient availability. American Journal of Botany 90 (1):143–52.
- Safaya, N. M. 1976. Phosphorus-zinc interaction in relation to absorption rates of phosphorus, zinc, copper, manganese, and iron in corn. Soil Science Society of America Journal 40 (5):719–22.
- Saha, S., M. Saha, A. R. Saha, S. Mitra, S. K. Sarkar, A. K. Ghorai, and M. K. Tripathi. 2013. Interaction effect of potassium and sulfur fertilization on productivity and mineral nutrition of sunnhemp. Journal of Plant Nutrition 36 (8):1191–200.
- Sakal, R., A. P. Singh, and R. B. Sinha. 1988. Effect of different soil fertility levels on response of wheat to zinc application on calciorthent. Journal of the Indian Society of Soil Science 36:125–27.
- Salvagiotti, F., J. M. Castellarín, D. J. Miralles, and H. M. Pedrol. 2009. Sulfur fertilization improves nitrogen use efficiency in wheat by increasing nitrogen uptake. Field Crops Research 113 (2):170–77.
- Schulz, B. 2010. Functional classification of plant plasma membrane transporters. In Plant cell monographs, eds. A. S. Murphy, W. Peer, and B. Schulz, 131–76. Berlin Heidelberg, Germany: Springer Verlag.
- Seadh, S. E., M. I. El-Abady, A. M. El-Ghamry, and S. Farouk. 2009. Influence of micronutrients foliar application and nitrogen fertilization on wheat yield and quality of grain and seed. Journal of Biological Sciences 9 (8):851–58.
- Shi, R., Y. Zhang, X. Chen, Q. Sun, F. Zhang, V. Römheld, and C. Zou. 2010. Influence of long-term nitrogen fertilization on micronutrient density in grain of winter wheat (Triticum aestivum L.). Journal of Cereal Science 51 (1):165–70.
- Shinmachi, F., P. Buchner, J. L. Stroud, S. Parmar, F. J. Zhao, S. P. McGrath, and M. J. Hawkesford. 2010. Influence of sulfur deficiency on the expression of specific sulfate transporters and the distribution of sulfur, selenium, and molybdenum in wheat. Plant Physiology 153 (1):327–36.
- Shukla, U. C., and N. Singh. 1979. Phosphorus-copper relationship in wheat. Plant and Soil 53 (3):399–402.
- Sims, J. L., W. O. Atkinson, and C. Smitobol. 1975. Mo and N effects on growth, yield, and Mo composition of burley tobacco. Agronomy Journal 67 (6):824–28.
- Sims, J. L., J. E. Leggett, and U. R. Pal. 1979. Molybdenum and sulfur interaction effects on growth, yield, and selected chemical-constituents of burley tobacco. Agronomy Journal 71 (1):75–78.
- Sinclair, A. G., J. D. Morrison, L. C. Smith, and K. G. Dodds. 1996. Effects and interactions of phosphorus and sulphur on a mown white clover/ryegrass sward 2. Concentrations and ratios of phosphorus, sulphur, and nitrogen in clover herbage in relation to balanced plant nutrition. New Zealand Journal of Agricultural Research 39 (3):435–45.
- Singh, A. K. 1991. Response of pre-flood, early rainy-season maize (zea-mays) to graded-levels of nitrogen and phosphorus in ganga diara tract of bihar. Indian Journal of Agronomy 36 (4):508–10.
- Singh, D. P., L. H. Liu, S. K. Oiseth, J. Beloy, L. Lundin, M. J. Gidley, and L. Day. 2010. Influence of boron on carrot cell wall structure and its resistance to fracture. Journal of Agricultural and Food Chemistry 58 (16):9181–89.
- Singh, J. P., R. E. Karamanos, and J. W. B. Stewart. 1988. The mechanims of phosphorus-induced zinc deficiency in bean (Phaseolus vulgaris L.). Canadian Journal of Soil Science 68:345–58.
- Sinha, M. N., A. G. Kavitkar, and M. Parshad. 1973. Optimum nitrogen and phosphorus requirements of late-sown wheat (triticum-aestivum l). Indian Journal of Agricultural Sciences 43 (11):1002–05.
- Sinha, P., B. K. Dube, and C. Chatterjee. 2003. Phosphorus stress alters boron metabolism of mustard. Communications in Soil Science and Plant Analysis 34 (3–4):315–26.
- Sinha, P., R. Jain, and C. Chatterjee. 2000. Interactive effect of boron and zinc on growth and metabolism of mustard. Communications in Soil Science and Plant Analysis 31 (1–2):41–49.
- Smith, G. S., I. S. Cornforth, and H. V. Henderson. 1985. Critical leaf concentrations for deficiencies of nitrogen, potassium, phosphorus, sulfur, and magnesium in perennial ryegrass. New Phytologist 101 (3):393–409.
- Soltangheisi, A., Z. A. Rahman, C. F. Ishak, H. M. Musa, and H. Zakikhani. 2014. Effect of zinc and phosphorus supply on the activity of carbonic anhydrase and the ultrastructure of chloroplast in sweet corn (Zea mays var. saccharata). Asian Journal of Plant Sciences 13 (2):51–58.
- Steffen, W., K. Richardson, J. Rockström, S. E. Cornell, I. Fetzer, E. M. Bennett, R. Biggs, S. R. Carpenter, W. De Vries, C. A. De Wit, C. Folke, D. Gerten, J. Heinke, G. M. Mace, L. M. Persson, V. Ramanathan, B. Reyers, and S. Sörlin. 2015. Planetary boundaries: Guiding human development on a changing planet. Science 347:6223.
- Sumner, M. E., and M. P. W. Farina. 1986. Phosphorus interactions with other nutrients and lime in field cropping systems. Advances in Soil Science 5:201–36.
- Suttle, N. F., and E. J. Underwood. 2010. The mineral nutrition of livestock. Wallingford: CABI.
- Svecnjak, Z., M. Jenel, M. Bujan, D. Vitali, and I. V. Dragojevic. 2013. Trace element concentrations in the grain of wheat cultivars as affected by nitrogen fertilization. Agricultural and Food Science 22 (4):445–51.
- Tandon, P. K. 1996. Effect of zinc and iron supply on production potential of rice (Oryza sativa L). Journal of Agronomy and Crop Science-Zeitschrift Fur Acker Und Pflanzenbau 176 (3):213–16.
- Tilman, D., C. Balzer, J. Hill, and B. L. Befort. 2011. Global food demand and the sustainable intensification of agriculture. Proceedings of the National Academy of Sciences of the United States of America 108 (50):20260–64.
- Tisdale, S. L., W. L. Nelson, and J. D. Beaton. 1985. Soil fertility and fertilizers. New York: Macmillan.
- Touchton, J. T., J. W. Johnson, and B. M. Cunfer. 1980. The relationship between phosphorus and copper concentrations in wheat. Communications in Soil Science and Plant Analysis 11 (11):1051–66.
- Verma, T. S., and R. M. Bhagat. 1990. Zinc and nitrogen interaction in wheat grown in limed and unlimed acid alfisol. Fertilizer Research 22 (1):29–35.
- Vistoso, E. M., M. Alfaro, and M. L. Mora. 2012. Role of molybdenum on yield, quality, and photosynthetic efficiency of white clover as a result of the interaction with liming and different phosphorus rates in andisols. Communications in Soil Science and Plant Analysis 43 (18):2342–57.
- Wallace, A. 1990. Interactions of two parameters in crop production and in general biology: Sequential additivity, synergism, antagonism. Journal of Plant Nutrition 13 (3–4):327–42.
- Wapakala, W. W. 1973. The effect of nitrogen, phosphorus and copper on grain yield of wheat on a copper deficient soil. East African Agricultural and Forestry Journal 38 (3):229–40.
- Welch, R. M. 1995. Micronutrient nutrition of plants. Critical Reviews in Plant Sciences 14 (1):49–82.
- Westfall, D. G., W. B. Anderson, and R. J. Hodges. 1971. Iron and zinc response of chlorotic rice grown on calcareous soils. Agronomy Journal 63 (5):702–05.
- White, P. J. 2012. Ion uptake mechanisms of individual cells and roots: Short-distance transport. In Marschner’s mineral nutrition of higher plants, eds. P. Marschner, 7–47. San Diego: Academic Press.
- White, P. J., H. C. Bowen, B. Marshall, and M. R. Broadley. 2007. Extraordinarily high leaf selenium to sulfur ratios define ‘Se-accumulator’ plants. Annals of Botany 100 (1):111–18.
- White, P. J., H. C. Bowen, P. Parmaguru, M. Fritz, W. P. Spracklen, R. E. Spiby, M. C. Meacham, A. Mead, M. Harriman, L. J. Trueman, B. M. Smith, B. Thomas, and M. R. Broadley. 2004. Interactions between selenium and sulphur nutrition in Arabidopsis thaliana. Journal of Experimental Botany 55 (404):1927–37.
- White, P. J., J. E. Bradshaw, M. F. B. Dale, G. Ramsay, J. P. Hammond, and M. R. Broadley. 2009. Relationships between yield and mineral concentrations in potato tubers. HortScience 44 (1):6–11.
- Wilkinson, S. R., D. L. Grunes, and M. E. Sumner. 2000. Nutrient interactions in soil and plant nutrition. In Handbook of soil science, eds. M. E. Sumner, D89–D108. Boca Raton: CRC Press.
- Wu, C. Y. H., J. Lu, and Z. Y. Hu. 2014. Influence of sulfur supply on the iron accumulation in rice plants. Communications in Soil Science and Plant Analysis 45 (8):1149–61.
- Xiong, H., Y. Kakei, T. Kobayashi, X. Guo, M. Nakazono, H. Takahashi, H. Nakanishi, H. Shen, F. Zhang, N. K. Nishizawa, and Y. Zuo. 2013. Molecular evidence for phytosiderophore-induced improvement of iron nutrition of peanut intercropped with maize in calcareous soil. Plant, Cell and Environment 36 (10):1888–902.
- Xue, Y. F., W. Zhang, D. Y. Liu, S. C. Yue, Z. L. Cui, X. P. Chen, and C. Q. Zou. 2014. Effects of nitrogen management on root morphology and zinc translocation from root to shoot of winter wheat in the field. Field Crops Research 161:38–45.
- Yassen, A., E. A. A. Abou El-Nour, and S. Shedeed. 2010. Response of wheat to foliar spray with urea and micronutrients. Journal of American Science 6 (9):14–22.
- Zebarth, B. J., C. J. Warren, and R. W. Sheard. 1992. Influence of the rate of nitrogen-fertilization on the mineral-content of winter-wheat in ontario. Journal of Agricultural and Food Chemistry 40 (9):1528–30.
- Zhang, F., J. F. Niu, W. F. Zhang, X. P. Chen, C. J. Li, L. X. Yuan, and J. C. Xie. 2010. Potassium nutrition of crops under varied regimes of nitrogen supply. Plant and Soil 335 (1–2):21–34.
- Zhang, F. S., J. Shen, and Y. G. Zhu. 2006. Nutrient interactions in soil-plant system. In Encyclopedia of soil science, ed. R. Lal, 1153–56. New York/London: Taylor & Francis.
- Zhang, Y. Q., Y. Deng, R. Y. Chen, Z. L. Cui, X. P. Chen, R. Yost, F. S. Zhang, and C. Q. Zou. 2012. The reduction in zinc concentration of wheat grain upon increased phosphorus-fertilization and its mitigation by foliar zinc application. Plant and Soil 361 (1–2):143–52.
- Zinn, M., B. Witholt, and T. Egli. 2004. Dual nutrient limited growth: Models, experimental observations, and applications. Journal of Biotechnology 113 (1–3):263–79.
- Zuchi, S., S. Cesco, and S. Astolfi. 2012. High S supply improves Fe accumulation in durum wheat plants grown under Fe limitation. Environmental and Experimental Botany 77:25–32.
- Zuo, Y., and F. Zhang. 2008. Effect of peanut mixed cropping with gramineous species on micronutrient concentrations and iron chlorosis of peanut plants grown in a calcareous soil. Plant and Soil 306 (1–2):23–36.
Appendix 1.
Interactions between nutrients
Literature has been examined for studies about interactions between nutrients. Studies that determined the yield as a function of the supply of two nutrients combined or supplied separately have been included in . The yield (y) is given for the zero treatment (y0), for the treatment with nutrient a (ya), nutrient b (yb), and the combined treatment (yab). An interaction between two nutrients is synergistic when the combined effect of two nutrients on yield (yab/y0) is greater than the product of their individual effects (ya/y0 x yb/y0). When the combined effect is less, the interaction is antagonistic. The expected yield for the combined treatment (Eq. (1)) is calculated as proposed by Wallace (Citation1990) as the product of the relative yields from the single effects (ya and yb). Zero-interaction indicates the absence of interaction (yab/y0 = ya/y0 x yb/y0). A specific case of synergism has been defined by Wallace (Citation1990) as Liebig-synergism. In this case, the yield in the combined treatment (yab) is higher than any of the individual treatments (y0, ya or yb), and the yield at the starting point (y0) is limited dominantly by one nutrient. In the end point (yab), there is synergism.
Table 14. Dataset of 117 interactions between nutrients on crop yield (for explanation of table see text in Appendix 1) on the basis of 94 references, for 74 combinationsa of nutrients.
In some studies, a variety of concentrations has been studied. Only one combination of two nutrients is given in . In most cases, the highest supply of nutrients was chosen, except in the case of toxic effects (if there is a yield decrease due to the addition of a single nutrient that is known to be toxic at high concentrations). In those cases, the concentrations with the highest yield have been chosen.
Some studies have given an ANOVA table about the interaction and the effect of individual treatments. The type of interaction has been estimated from the ANOVA table and figures presented in these studies.
Note: ideally in nutrient interaction studies all other factors should be at an optimum level, except the nutrients under investigation (Fageria, Baligar, and Jones Citation2011). This is rather difficult to comply with when studying interactions between nutrients such as iron, manganese, and zinc where the availability is often determined by the soil conditions.